DOI:
10.1039/C7QO00714K
(Research Article)
Org. Chem. Front., 2018,
5, 19-23
Photoredox-catalyzed cascade addition/cyclization of N-propargyl aromatic amines: access to 3-difluoroacetylated or 3-fluoroacetylated quinolines†
Received
13th August 2017
, Accepted 17th September 2017
First published on 18th September 2017
Abstract
3-Difluoroacetylated quinolines and 3-fluoroacetylated quinolines were synthesized via a visible-light-induced cascade addition/cyclization of N-propargyl aromatic amines with ethyl bromodifluoroacetate or ethyl bromofluoroacetate catalyzed by fac-Ir(ppy)3 under mild conditions. For the substrates bearing various substituents on the aniline ring and benzene ring, the reaction proceeded smoothly to give the corresponding difluoroacetylated or fluoroacetylated quinolines in moderate to high yields.
Introduction
Among the common fused heteroaromatic compounds, quinolines occupy a distinctly important position because of the valued application of this structural motif in natural products1 and pharmaceuticals.2 Quinine, for example, a quinoline derivative which was isolated from the bark of cinchona tree in 1820, is a general drug used to treat malaria for more than one century. Quinoline metal complexes were also efficient electrogenerated chemiluminescence materials.3 The 8-aminoquinoline amide is known to be a good directing group for selective C–H bond functionalization (Fig. 1).4 After Skraup's discovery about the formation of quinoline by heating aniline with glycerol, sulfuric acid and an oxidizing agent in 1880,5 many modified methods were achieved by using aniline and its derivatives as the basic starting materials.6N-Propargylamines are extensively used for constructing many significant nitrogen-containing heterocyclic compounds.7 The cyclization and aromatization of N-propargyl aromatic amines have been used to access a series of substituted quinolines. In 2001, Iqbal and co-workers developed a CuCl-catalyzed three-component reaction of arylamine, aldehyde and terminal alkyne for the synthesis of quinoline derivatives, and the N-propargylamines were proved to be the intermediate products in this transformation.8 Several other studies via multicomponent reactions using similar protocols to furnish quinoline skeletons were reported in succession.9 The intermolecular electrophilic cyclization of N-propargyl aromatic amines with ICl, I2, Br2 and PhSeBr resulted in a series of substituted quinolines.10 Tang, Zhao and co-workers explored an intermolecular cascade radical addition/cyclization of N-propargyl aromatic amines with arylsulfonylhydrazides or H-phosphine oxides mediated by tert-butyl hydroperoxide to prepare 3-arylsulfonylquinolines or 3-phosphinoylquinolines.11
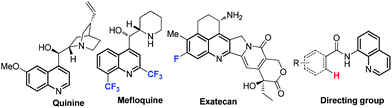 |
| Fig. 1 Some representative quinoline derivatives. | |
One of the research interests of our group focuses on the synthesis of functionalized heterocycles, especially, the “drug bias” heterocyclic compounds. For example, cyanomethylated coumarins, sulfonylmethyl or nitromethyl substituted isoquinoline-1,3(2H,4H)-diones were constructed via cascade radical addition/cyclizations.12 As we all know, the organofluorine compounds are of increasing significance in the field of drug design and discovery because of the fluorine's special electronegativity and lipophilicity.13 The fluorine-containing quinoline derivative mefloquine shows very good efficacy in treating malaria, and exatecan as a fluorinated camptothecin analogue exhibits good antitumor activity (Fig. 1).14 The potential application value of fluorinated heterocycles promotes us to study the efficient methods for the synthesis of these compounds. Both bromodifluoroacetate and bromofluoroacetate are cheap fluorine-containing reagents and have been successfully incorporated into a series of alkenes, alkynes and heterocyclic compounds under photoredox catalysis.15 Herein, we describe a visible light-induced cascade addition/cyclization reaction of N-propargyl aromatic amines to prepare difluoroacetylated quinolines or fluoroacetylated quinolines by using bromodifluoroacetate or bromofluoroacetate as the fluorine resource at room temperature.
Results and discussion
We initiated our study by evaluating the cyclization of N-(3-phenylprop-2-yn-1-yl)aniline (1a) with ethyl bromodifluoroacetate (2) in the presence of 2 mol% of the photocatalyst fac-Ir(ppy)3 and 2.5 equiv. of K2CO3. After irradiation with 5 W blue LEDs for 12 h in N,N-dimethylacetamide (DMA) under an Ar atmosphere, the desired product ethyl 2,2-difluoro-2-(4-phenylquinolin-3-yl)acetate (3a) was obtained in 62% yield (Table 1, entry 1). A range of solvents were tested and CH3CN showed the best efficiency with a delightful yield of 86% (entries 2–6). The reaction hardly took place in the absence of a base (entry 7). The inorganic and organic bases were sequentially screened and the results showed that K2CO3 was the best one (entries 6, 8–12). According to the screening experiments with several other photocatalysts such as eosin Y, Ru(phen)3Cl2 and Ir(ppy)2(dtbbpy)PF6, fac-Ir(ppy)3 was the most effective and the reaction gave 3a in 86% yield in a 2 mol% catalyst loading (entries 6, 13–15). When the amount of catalyst fac-Ir(ppy)3 was reduced to 1 mol%, product 3a was obtained in lower yield (55%), and a comparable yield (85%) was obtained with 5 mol% of catalyst (entries 16 and 17). Furthermore, the cyclization reaction could not occur without any photocatalyst, light irradiation or in air (entries 18–20).
Table 1 Optimization of the reaction conditionsa

|
Entry |
Photocatalyst (mol%) |
Base |
Solvent |
Yield (%) |
Reaction conditions: 1a (0.2 mmol), 2 (0.5 mmol), base (0.5 mmol), photocatalyst (2 mol%) and solvent (2 mL) were carried out in a sealed tube under an Ar atmosphere upon irradiation of 5 W blue LEDs at room temperature for 12 h.
In the dark.
In air.
|
1 |
fac-Ir(ppy)3 (2) |
K2CO3 |
DMA |
62 |
2 |
fac-Ir(ppy)3 (2) |
K2CO3 |
DMSO |
0 |
3 |
fac-Ir(ppy)3 (2) |
K2CO3 |
Toluene |
40 |
4 |
fac-Ir(ppy)3 (2) |
K2CO3 |
Dioxane |
65 |
5 |
fac-Ir(ppy)3 (2) |
K2CO3 |
DCM |
81 |
6
|
fac-Ir(ppy)
3
(2)
|
K
2
CO
3
|
CH
3
CN
|
86
|
7 |
fac-Ir(ppy)3 (2) |
— |
CH3CN |
Trace |
8 |
fac-Ir(ppy)3 (2) |
Cs2CO3 |
CH3CN |
66 |
9 |
fac-Ir(ppy)3 (2) |
K3PO4 |
CH3CN |
75 |
10 |
fac-Ir(ppy)3 (2) |
NaHCO3 |
CH3CN |
62 |
11 |
fac-Ir(ppy)3 (2) |
DABCO |
CH3CN |
79 |
12 |
fac-Ir(ppy)3 (2) |
2,6-Lutidine |
CH3CN |
66 |
13 |
eosin Y (2) |
K2CO3 |
CH3CN |
0 |
14 |
Ru(phen)3Cl2 (2) |
K2CO3 |
CH3CN |
54 |
15 |
Ir(ppy)2(dtbbpy)PF6 (2) |
K2CO3 |
CH3CN |
80 |
16 |
fac-Ir(ppy)3 (1) |
K2CO3 |
CH3CN |
55 |
17 |
fac-Ir(ppy)3 (5) |
K2CO3 |
CH3CN |
85 |
18 |
— |
K2CO3 |
CH3CN |
0 |
19b |
fac-Ir(ppy)3 (2) |
K2CO3 |
CH3CN |
0 |
20c |
fac-Ir(ppy)3 (2) |
K2CO3 |
CH3CN |
0 |
Having identified the optimal conditions for the cyclization of N-propargyl aromatic amines under photoredox catalysis, we then studied the substrate scope of this reaction by using bromodifluoroacetate as the reaction partner (Scheme 1). The effect of various substituents (R1) on the aromatic ring A of 1 was first examined. The reactions of N-propargyl aromatic amines with electron-donating groups such as methyl and methoxyl on the ring A proceeded smoothly and gave the corresponding products in good yields (3b–3e). For the meta-methyl substituted reactant 1d, two regioisomers 3d and 3d′ (1
:
3) were obtained in 81% combined yield. The para-trifluoromethoxyl and para-halogenated substrates were also suitable for the reaction to yield the desired products 3f–3h in satisfactory yields. When a strong electron-withdrawing trifluoromethyl group existed on the same position, however, the reaction only gave product 3i in 35% yield. The electron effect of the substituents on the aromatic ring B had almost no influence on the reaction since both electron-donating groups (methyl and methoxyl) and electron-withdrawing groups (chloro, cyano, acetyl, ester and phenyl) were compatible under the same conditions and the reaction gave products 3j–3r in good yields (64%–86%). It should be noted that the presence of a sterically hindered fluorenyl on the carbon–carbon triple bond did not obstruct the reaction and a high yield of 86% was obtained (3s). The presence of the heterocyclic group 2-thenyl was also permitted (3t). However, N-(but-2-yn-1-yl)aniline (1u) was far less reactive to generate the corresponding product 3u. In addition, comparable yields could also be obtained from the reactants with substituents on the two aromatic rings A and B (3v–3y).
 |
| Scheme 1 Synthesis of 3-difluoroacetylated quinoline derivatives. | |
We next examined the cascade addition/cyclization reaction of N-propargyl aromatic amines with ethyl bromofluoroacetate. In line with our expectation, the reaction also furnished 3-fluoroacetylated quinolines with moderate to good yields under the same conditions (Scheme 2, 5a, 5e, 5g, and 5r).
 |
| Scheme 2 Synthesis of 3-fluoroacetylated quinoline derivatives. | |
The reaction could be easily scaled up to 5 mmol of 1a, and product 3a was obtained in 75% yield (Scheme 3, eqn (1)). To demonstrate the synthetic utility of this reaction, we carried out further transformation of the product. Upon treatment with NaBH4, product 3a could be easily reduced to give 2,2-difluoro-2-(4-phenylquinolin-3-yl)ethan-1-ol (6) in 91% yield (Scheme 3, eqn (2)). The hydrolysis of the ester group of 3a resulted in the desired product 2,2-difluoro-2-(4-phenylquinolin-3-yl)acetic acid (7) in 90% yield (Scheme 3, eqn (3)).
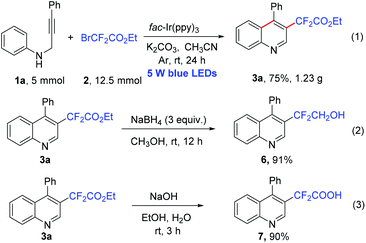 |
| Scheme 3 Synthesis of 3a on a gram scale and its related transformations. | |
To better understand the mechanism of the cyclization reaction, several control experiments were performed as shown in Scheme 4. When the radical scavenger 2,2,6,6-tetramethyl-1-piperidinyloxy (TEMPO) was added to the model reaction system, the reaction was obviously inhibited and no desired product 3a was produced (Scheme 4, eqn (1)). When the model reaction was carried out with ethene-1,1-diyldibenzene, only a coupling product 8 was isolated in 66% yield (Scheme 4, eqn (2)). The two experiments suggested that the cyclization reaction perhaps took place via a radical pathway. The intramolecular cyclization could not occur in the absence of 2 under the standard conditions, which indicated that the addition to a carbon–carbon triple bond might be prior to the cyclization of N-propargyl aromatic amine (Scheme 4, eqn (3)).
 |
| Scheme 4 Control experiments. | |
Based on the above results and relative literature reports, a plausible reaction mechanism is depicted in Scheme 5. Initially, irradiation of the photocatalyst fac-Ir(ppy)3 with visible light led to the formation of an excited state Ir(III)*, which underwent oxidative quenching with BrCF2COOC2H5 or BrCHFCOOC2H5via a single electron transfer (SET) process to generate the [fac-Ir(IV)(ppy)3]+ and radical A.16 The regioselective addition of radical A to the triple bond of N-propargyl aromatic amine 1a produced the alkenyl radical B, followed by intramolecular cyclization to provide aryl radical C, which was oxidized by Ir(IV)+ to generate the carbocation D and regenerate the IrIII-photocatalyst for the next cycle. The deprotonation of D in the presence of a base gave 1,2-dihydroquinoline E. Finally, the dehydro-aromatization of E under the irradiation of visible light furnished the desired product 3a.
 |
| Scheme 5 Proposed mechanism. | |
Conclusions
In summary, we have developed an efficient protocol for the preparation of 3-difluoroacetylated quinolines and 3-fluoroacetylated quinolines via fac-Ir(ppy)3 catalyzed fluoroacetylation, cyclization cascade aromatization of N-propargyl aromatic amines with ethyl bromodifluoroacetate or ethyl bromofluoroacetate under visible light irradiation. This method enables highly regioselective addition of a fluoroalkyl radical to the triple bond of N-propargyl aromatic amines with good group tolerance and satisfactory yields.
Conflicts of interest
There are no conflicts to declare.
Acknowledgements
This work was supported by the National Natural Science Foundation of China (Projects 21672104 and 21502097), the Key International (Regional) Joint Research Program of the NSFC (Grant No. 21420102002), the Natural Science Foundation of the Education Department of Jiangsu province (15KJB150015), and the Priority Academic Program Development of Jiangsu Higher Education Institutions. The authors also thank Mr Hailong Liu for the determination of HRMS.
Notes and references
- For selected papers and reviews, see:
(a) K. E. Sayed, M. S. Al-Said, F. S. El-Feraly and S. A. Ross, J. Nat. Prod., 2000, 63, 995 CrossRef PubMed;
(b) A. Teichert, J. Schmidt, A. Porzel, N. Arnold and L. Wessjohann, J. Nat. Prod., 2000, 71, 1092 CrossRef PubMed;
(c) J. P. Michael, Nat. Prod. Rep., 2008, 25, 166 RSC;
(d) P.-Y. Chung, Z.-X. Bian, H.-Y. Pun, D. Chan, A. S.-C. Chan, C.-H. Chui, J. C.-O. Tang and K.-H. Lam, Future Med. Chem., 2015, 7, 947 CrossRef CAS PubMed.
-
(a) R. Musiol, J. Jampilek, V. Buchta, L. Silva, H. Niedbala, B. Podeszwa, A. Palka, K. Majerz-Maniecka, B. Oleksynd and J. Polanski, Bioorg. Med. Chem., 2006, 14, 3592 CrossRef CAS PubMed;
(b) S. Adsule, V. Barve, D. Chen, F. Ahmed, Q. P. Dou, S. Padhye and F. H. Sarkar, J. Med. Chem., 2006, 49, 7242 CrossRef CAS PubMed;
(c) S. Eswaran, A. V. Adhikari, I. H. Chowdhury, N. K. Pal and K. D. Thomas, Eur. J. Med. Chem., 2010, 45, 3374 CrossRef CAS PubMed;
(d) F. Hayat, E. Moseley, A. Salahuddin, R. L. V. Zyl and A. Azam, Eur. J. Med. Chem., 2011, 46, 1897 CrossRef CAS PubMed;
(e) A. Salahuddin, A. Inam, R. L. V. Zyl, D. C. Heslop, C.-T. Chen, F. Avecilla, S. M. Agarwal and A. Azam, Bioorg. Med. Chem., 2013, 21, 3080 CrossRef CAS PubMed.
- J. I. Kim, I.-S. Shin, H. Kim and J.-K. Lee, J. Am. Chem. Soc., 2005, 127, 1614 CrossRef CAS PubMed.
-
(a) V. G. Zaitsev, D. Shabashov and O. Daugulis, J. Am. Chem. Soc., 2005, 127, 13154 CrossRef CAS PubMed;
(b) D. Shabashov and O. Daugulis, J. Am. Chem. Soc., 2010, 132, 3965 CrossRef CAS PubMed;
(c) M. Nishino, K. Hirano, T. Satoh and M. Miura, Angew. Chem., Int. Ed., 2013, 52, 4457 CrossRef CAS PubMed;
(d) R. Shang, L. Ilies, A. Matsumoto and E. Nakamura, J. Am. Chem. Soc., 2013, 135, 6030 CrossRef CAS PubMed.
- F. W. Bergstrom, Chem. Rev., 1944, 35, 77 CrossRef CAS.
- For selected reviews, see:
(a) V. V. Kouznetsov, L. Y. V. Méndez and C. M. M. Gómez, Curr. Org. Chem., 2005, 9, 141 CrossRef CAS;
(b) S. Madapa, Z. Tusi and S. Batra, Curr. Org. Chem., 2008, 12, 1116 CrossRef CAS;
(c) S. M. Prajapati, K. D. Patel, R. H. Vekariya, S. N. Panchal and H. D. Patel, RSC Adv., 2014, 4, 24463 RSC;
(d) J. B. Bharate, R. A. Vishwakarma and S. B. Bharate, RSC Adv., 2015, 5, 42020 RSC;
(e) G. Chelucci and A. Porcheddu, Chem. Rec., 2017, 17, 200 CrossRef CAS PubMed.
-
(a) G. Abbiati, A. Arcadi, G. Bianchi, S. D. Giuseppe, F. Marinelli and E. Rossi, J. Org. Chem., 2003, 68, 6959 CrossRef CAS PubMed;
(b) E. Merkul, C. Boersch, W. Frank and T. J. J. Müller, Org. Lett., 2009, 11, 2269 CrossRef CAS PubMed;
(c) T. P. Lebold, A. B. Leduc and M. A. Kerr, Org. Lett., 2009, 11, 3770 CrossRef CAS PubMed;
(d) Z. Jiang, P. Lu and Y.-G. Wang, Org. Lett., 2012, 14, 6266 CrossRef CAS PubMed;
(e) B.-X. Yuan, F.-M. Zhang, Z.-M. Li, S.-H. Yang and R.-L. Yan, Org. Lett., 2016, 18, 5928 CrossRef CAS PubMed.
- H. Z. S. Huma, R. Halder, S. S. Kalra, J. Das and J. Iqbal, Tetrahedron Lett., 2002, 43, 6485 CrossRef.
-
(a) F.-P. Xiao, Y.-L. Chen, Y. Liu and J.-B. Wang, Tetrahedron, 2008, 64, 2755 CrossRef CAS;
(b) K. Cao, F.-M. Zhang, Y.-Q. Tu, X.-T. Zhuo and C.-A. Fan, Chem. – Eur. J., 2009, 15, 6332 CrossRef CAS PubMed;
(c) A. Kulkarni and B. Török, Green Chem., 2010, 12, 875 RSC;
(d) C. E. Meyet and C. H. Larsen, J. Org. Chem., 2014, 79, 9835 CrossRef CAS PubMed.
-
(a) X.-X. Zhang, M. A. Campo, T.-L. Yao and R. C. Larock, Org. Lett., 2005, 7, 763 CrossRef CAS PubMed;
(b) X.-X. Zhang, T.-L. Yao, M. A. Campo and R. C. Larock, Tetrahedron, 2010, 66, 1177 CrossRef CAS PubMed.
-
(a) L.-L. Zhang, S. Chen, Y.-Z. Gao, P.-B. Zhang, Y.-L. Wu, G. Tang and Y.-F. Zhao, Org. Lett., 2016, 18, 1286 CrossRef CAS PubMed;
(b) P.-B. Zhang, L.-L. Zhang, Y.-Z. Gao, G. Tang and Y.-F. Zhao, RSC Adv., 2016, 6, 60922 RSC.
-
(a) Y.-L. Yu, S.-Y. Zhuang, P. Liu and P.-P. Sun, J. Org. Chem., 2016, 81, 11489 CrossRef CAS PubMed;
(b) X. Liu, T.-T. Cong, P. Liu and P.-P. Sun, Org. Biomol. Chem., 2016, 14, 9416 RSC;
(c) X.-X. Li, S.-Y. Zhuang, X.-X. Fang, P. Liu and P.-P. Sun, Org. Biomol. Chem., 2017, 15, 1821 RSC.
-
(a) S. Purser, P. R. Moore, S. Swallow and V. Gouverneur, Chem. Soc. Rev., 2008, 37, 320 RSC;
(b) J. Wang, M. Sánchez-Roselló, J. L. Aceña, C. D. Pozo, A. E. Sorochinsky, S. Fustero, V. A. Soloshonok and H. Liu, Chem. Rev., 2014, 114, 2432 CrossRef CAS PubMed.
- F.-X. Sun, A. Tohgo, M. Bouvet, S. Yagi, R. Nassirpour, A. R. Moossa and R. M. Hoffman, Cancer Res., 2003, 63, 80 CAS.
-
(a) J. D. Nguyen, J. W. Tucker, M. D. Konieczynska and C. R. J. Stephenson, J. Am. Chem. Soc., 2011, 133, 4160 CrossRef CAS PubMed;
(b) C.-J. Wallentin, J. D. Nguyen, P. Finkbeiner and C. R. J. Stephenson, J. Am. Chem. Soc., 2012, 134, 8875 CrossRef CAS PubMed;
(c) H. Jiang, Y.-Z. Cheng, R.-Z. Wang, M.-M. Zheng, Y. Zhang and S.-Y. Yu, Angew. Chem., Int. Ed., 2013, 52, 13289 CrossRef CAS PubMed;
(d) X.-J. Wei, D.-T. Yang, L. Wang, T. Song, L.-Z. Wu and Q. Liu, Org. Lett., 2013, 15, 6054 CrossRef CAS PubMed;
(e) X.-Y. Sun and S.-Y. Yu, Org. Lett., 2014, 16, 2938 CrossRef CAS PubMed;
(f) S. Tang, Y.-L. Deng, J. Li, W.-X. Wang, G.-L. Ding, M.-W. Wang, Z.-P. Xiao, Y.-C. Wang and R.-L. Sheng, J. Org. Chem., 2015, 80, 12599 CrossRef CAS PubMed;
(g) F. Gao, C. Yang, N. Ma, G.-L. Gao, D.-Z. Li and W.-J. Xia, Org. Lett., 2016, 18, 600 CrossRef CAS PubMed;
(h) X.-Y. Sun and S.-Y. Yu, Chem. Commun., 2016, 52, 10898 RSC.
-
(a) W.-J. Fu, M. Zhu, G.-L. Zou, C. Xu and Z.-Q. Wang, Asian J. Org. Chem., 2014, 3, 1273 CrossRef CAS;
(b) Z.-X. Gu, H.-L. Zhang, P. Xu, Y.-X. Cheng and C.-J. Zhu, Adv. Synth. Catal., 2015, 357, 3057 CrossRef CAS;
(c) K. Tong, T.-Y. Zheng, Y. Zhang and S.-Y. Yu, Adv. Synth. Catal., 2015, 357, 3681 CrossRef CAS;
(d) W.-J. Fu, M. Zhu, G.-L. Zou, C. Xu, Z.-Q. Wang and B.-M. Ji, J. Org. Chem., 2015, 80, 4766 CrossRef CAS PubMed;
(e) P. Xu, K.-D. Hu, Z.-X. Gu, Y.-X. Cheng and C.-J. Zhu, Chem. Commun., 2015, 51, 7222 RSC;
(f) J. Li, J.-Z. Chen, W. Jiao, G.-Q. Wang, Y. Li, X. Cheng and G.-G. Li, J. Org. Chem., 2016, 81, 9992 CrossRef CAS PubMed;
(g) S. B. Nagode, A. K. Chaturvedi and N. Rastogi, Asian J. Org. Chem., 2017, 6, 453 CrossRef CAS;
(h) L.-C. Yu, J.-W. Gu, S. Zhang and X. Zhang, J. Org. Chem., 2017, 82, 3943 CrossRef CAS PubMed.
Footnote |
† Electronic supplementary information (ESI) available: Full experimental information, compound characterization and copies of NMR spectra. See DOI: 10.1039/c7qo00714k |
|
This journal is © the Partner Organisations 2018 |