DOI:
10.1039/C7FO00724H
(Paper)
Food Funct., 2017,
8, 3104-3110
A study of glycaemic effects following acute anthocyanin-rich blueberry supplementation in healthy young adults
Received
16th May 2017
, Accepted 15th July 2017
First published on 28th July 2017
Abstract
The postprandial response to ingested carbohydrate is recognised as a marker of metabolic health. Postprandial hyperglycaemia is observed in type 2 diabetes mellitus and is a significant risk factor for cardiovascular disease. Cognitive deficits are also associated with type 2 diabetes. Therefore interventions which moderate postprandial glucose profiles are desirable. Here we investigated the impact of anthocyanin-rich wild blueberries on postprandial glucose response. Seventeen healthy young adults consumed a range of doses of freeze-dried wild blueberry powder, in smoothie form, in both sugar-matched and no-added-sugar conditions. Plasma glucose was determined by a capillary sampling method at baseline and at regular intervals up to 2.5 hours postprandially. Blueberries were observed to significantly extend the postprandial glucose response beyond the period observed for a sugar-matched control, characteristic of a beneficial glycaemic response. Furthermore, blueberries were observed to reduce peak postprandial glucose levels, although statistical significance was not achieved. The findings suggest a tempering of the postprandial glucose response in the presence of anthocyanin-rich blueberry, and are discussed with reference to likely glucoregulatory mechanisms of action and their implications for cognitive and type 2 diabetes research.
Introduction
The postprandial response to ingested carbohydrate is recognised as a marker of metabolic health. Postprandial hyperglycaemia is observed in type 2 diabetes mellitus and has been shown to be a risk factor for cardiovascular disease.1 Cognitive deficits are also associated with type 2 diabetes2,3 and hypertension.4 Therefore, interventions which moderate postprandial glucose by limiting periods of hyper- or hypoglycaemia are desirable.
Flavonoids are polyphenol phytonutrients found in foods such as berries, cocoa, and grains. Flavonoid-rich foods have been observed to influence blood glucose and glucose homeostasis. For example, key mechanisms have been identified in vitro including inhibition of carbohydrate digestion and glucose absorption, facilitation of insulin synthesis and secretion, and facilitation of glucose uptake by cells.5–10In vivo animal models have demonstrated that these mechanisms can impact upon the immediate postprandial period in addition to longer term glucoregulatory health.11 Epidemiological data support these findings;12,13 in particular intake of the flavonoid subgroup anthocyanins is associated with lower incidence of type 2 diabetes and increased insulin sensitivity. However few research trials have been conducted in humans that investigate moderation of the glycaemic response following anthocyanin-rich foods,14 or have considered how these effects may impact on cognitive function and type 2 diabetes.
Human research to date has produced mixed findings. Attenuated and/or delayed postprandial blood glucose concentrations have been observed in healthy adults following anthocyanin-rich interventions including cranberry juice,15 mixed berry puree,16 green tea with mixed freeze-dried fruit powders,17 and freeze-dried wild blueberry powder.18 In glucose intolerant subjects, attenuation of peak glucose has been observed following maqui berry extract.19 Despite these positive findings, other similar studies have failed to replicate these effects of anthocyanin-rich foods on glycaemic response. For example, in healthy adults, fresh strawberries,20 cranberry juice,21 and fresh blueberries and raspberries22 had no significant effect on postprandial blood glucose concentration relative to control conditions. In overweight adults, freeze-dried strawberry powder23 and acai fruit puree24 produced no glucose effects; however, significant benefits for the insulin response were evident. Methodological limitations were evident in a number of these studies, for example: low anthocyanins levels in the intervention,21,23 no quantification of anthocyanins level,15,17,20,22 infrequent blood sampling time points,24 and an inadequately matched control condition.18 In the latter case, glucose effects were observed but were attributed to the fructose content of the intervention rather than any anthocyanins, other flavonoids, or other polyphenol phytonutrients also present. However, the control condition in the study was not matched for fructose content making it impossible to eliminate fructose effects in this case.
Whilst findings are not conclusive, there is enough evidence to suggest that consumption of anthocyanin-rich foods can modulate the postprandial glycaemic response. The degree of this response and the associated implications for health outcomes in humans remain to be determined. A major shortcoming of the current literature is the absence of clearly defined anthocyanin concentrations, or indeed the concentrations of other polyphenol phytonutrients present. Indeed, it should be noted that anthocyanin-rich foods typically contain multiple flavonoid subgroups, in addition to a plethora of other polyphenol phytonutrients, and therefore when conducting investigations using anthocyanin-rich whole foods it is impossible to solely attribute bioactive effects to anthocyanins. Therefore, not surprisingly, knowledge of the specific impact of anthocyanins on blood glucose remains limited.6 In light of this, the aim of the current study is to determine postprandial blood glucose profiles following doses of blueberry, with quantified levels of anthocyanins, in healthy adults. Wild blueberries have been selected as they are a rich source of anthocyanins for which the rates of absorption and metabolism in humans are well documented across a range of doses,25 and for which there are known cognitive26,27 and cardiovascular benefits.28,29 While there are other polyphenol phytonutrients present, anthocyanins are the most abundant subgroup present, therefore we have quantified our doses according to anthocyanins content. In vitro evidence has specifically related anthocyanins to glucoregulatory mechanisms of action,5–10 and epidemiological data has associated habitual anthocyanins consumption with improved metabolic outcome.12,13
Materials and methods
Ethical statement
All subjects gave informed consent before participating. The study was conducted in accordance with the Declaration of Helsinki, and the protocol was reviewed by the University of Reading PCLS Ethics Committee (SREC 2015-053-CW, May 2015).
Participants
Seventeen young adults (4 male), with a mean age of 24.1 years (s.d. 4.9) and a mean BMI of 23.7 kg m−2 (s.d. 3.6) were recruited via email and social media from staff and student populations at the University of Reading. Participants were required to be non-smokers, free from diagnosed illness or disease, not pregnant, or taking any medication, and free from food allergies.
Design
The study was a double-blind, five-condition, counterbalanced, crossover design comparing doses of anthocyanin-rich blueberry powder containing 0 mg, 310 mg and 724 mg anthocyanins in both sugar-matched and no-added-sugar conditions (see Table 1 for details of drink preparation and composition). Doses were based on a previous FMD study that used the same source of blueberry powder; absorption and metabolism data were previously published alongside FMD data for these exact doses.25 Blood glucose measurements were recorded using a clinically approved finger prick test (Roche Accu-Chek Aviva) at regular intervals (specified below) for 2½ hours post ingestion. A trained researcher carried out the capillary sampling following World Health Organisation guidelines.
Table 1 Composition of each experimental condition. All conditions were prepared by dissolving the required quantities of freeze dried blueberry powder, sugars and ascorbic acid in 500 ml water. Sugar-matched conditions were matched to the naturally occurring sugar content of the highest dose. Blueberry powder, from the cultivar vaccinium angustifolium, was provided by the Wild Blueberry Association of North America
Condition |
Freeze-dried powder (g) |
Approx. fresh blueberry equivalent (g) |
Anthocyanins (mg) |
Vitamin C (mg) |
Fructose (g) |
Glucose (g) |
No-added-sugar control |
0 |
0 |
0 |
9.5 |
0 |
0 |
Sugar-matched control |
0 |
0 |
0 |
9.5 |
21 |
19 |
No-added-sugar low dose |
34 |
235 |
310 |
9.5 |
9 |
8 |
Sugar-matched low dose |
34 |
235 |
310 |
9.5 |
21 |
19 |
Sugar-matched high dose |
80 |
555 |
724 |
9.5 |
21 |
19 |
Procedure
Participants attended the lab a total of five mornings, each separated by a minimum of 4 days (mean 7.69, s.d. 2.80). Participants were required to follow a low polyphenol diet for 24 hours prior to each visit and were asked to fast for 2 hours immediately prior to attending. On arrival, baseline blood glucose was recorded. Participants then consumed one of five test condition drinks which were prepared by an independent researcher in an opaque flask to maintain double blinding. Drink order was counterbalanced. Participants were asked to consume the drink as quickly as possible within a maximum 10 minutes period.24,25 Blood glucose measurements were recorded at pre-consumption, 15, 30, 45, 60, 90, 120 and 150 minutes postprandially.
Data analysis
All data were analysed using IBM SPSS statistics version 22. As recommended by an independent statistician data were analysed with a linear mixed model (LMM), using an unstructured covariance matrix for repeated blood glucose measurements. Time, Dose and Time × Dose interaction were included as fixed factors in the model. Subjects were included as random effects and BMI was included as a covariate. Post hoc comparisons with Bonferroni corrections were applied to investigate any significant effects. For each dose response curve, incremental area under the curve (iAUC) was calculated using the trapezoid rule.30 Incremental analysis, determined by excluding the area below baseline, has been shown to be a more representative measure of rapid postprandial changes in glycaemic status than total AUC analysis which is strongly correlated with basal blood glucose level.30 Between-subjects differences in baseline glucose were taken into account during the calculation. LMM analysis was used to determine the effect of dose on iAUC, again using an unstructured covariance matrix for repeat doses. Dose was included as a fixed factor in the model, and BMI was included as a covariate.
Results
Following publication, data supporting the results reported in this paper will be openly available from the University of Reading Research Data Archive at http://researchdata.reading.ac.uk/. Mean blood glucose concentrations are summarised in Fig. 1. Mean iAUC values are summarised in Table 2.
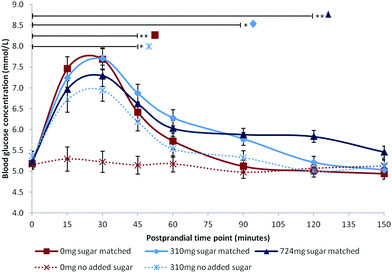 |
| Fig. 1 Mean blood glucose concentration recorded at baseline, and following each dose at fixed postprandial time points. Values are estimated marginal means adjusted for BMI. Error bars represent standard error of the mean. Horizontal bars indicate the final time point at which blood glucose remained significantly elevated compared with baseline for each dose, **(p < 0.01), *(p < 0.05). | |
Table 2 Mean iAUC values for each intervention condition. Values are estimated marginal means adjusted for BMI. Means not sharing a common superscript are significantly different from each other (Bonferroni-corrected, p < 0.05)
Condition |
iAUC (mmol min l−1) |
|
Mean |
SE |
No-added-sugar control |
34.38a |
8.66 |
No-added-sugar low dose |
78.47ab |
15.73 |
Sugar-matched control |
119.00b |
17.38 |
Sugar-matched low dose |
140.03b |
16.73 |
Sugar-matched high dose |
138.14b |
16.34 |
Blood glucose effects
All factors were statistically significant: Time [F(7,84.00) = 45.17, p < 0.001], Dose [F(4,59.06) = 15.12, p < 0.001], Time × Dose [F(28,84.00) = 4.53, p < 0.001]. BMI was a significant covariate [F(1,15.99) = 5.64, p = 0.030] with higher BMI predicting higher glucose levels [β = 0.047]. Post hoc comparisons for the three sugar-matched conditions revealed that the highest anthocyanin dose (724 mg) extended the glycaemic response such that blood glucose remained significantly elevated at 120 minutes compared with baseline levels [p = 0.008]. Blood glucose remained significantly elevated compared with baseline at 90 minutes [p = 0.016] following the sugar-matched low dose (310 mg), and at 45 minutes [p < 0.001] following the sugar-matched control. The apparent attenuations of mean peak observed in Fig. 1 for the sugar-matched blueberry doses, compared with the sugar-matched control condition, were non-significant at postprandial time points of 15 minutes (p > 0.999) and 30 minutes (p > 0.999). The no-added-sugar control condition profile confirmed that there was no significant blood glucose effect (hyper- or hypoglycaemic) associated with simply consuming a 500 ml sugar-free beverage.
iAUC
Dose was observed to be a significant predictor of iAUC [F(4,17.12) = 14.02, p < 0.001]. BMI did not reach significance as a covariate [F(1,17.88) = 0.07, p = 0.795]. Post hoc comparisons revealed no significant difference in iAUC between any of the three sugar-matched conditions [p > 0.1]. Other comparisons are summarised in Table 2.
Discussion
The results demonstrated that anthocyanin-rich blueberry significantly extended the postprandial glycaemic response compared to the equivalent sugar dose in the absence of blueberry. Indeed, blood glucose levels remained significantly elevated above baseline for 2 hours following a blueberry dose containing 724 mg anthocyanins, and for 1.5 hours following a lower 310 mg dose. These post-peak elevations were in the range of 0.5–1.5 mmol l−1 above fasting baseline which is well within the healthy postprandial blood glucose range31 and is metabolically beneficial through the avoidance of reactive hypoglycaemic episodes32 where glucose level falls close to or below fasting levels. Additionally, the level of blueberries required to elicit this effect is easily achievable within the normal diet, requiring the equivalent of 235–555 g fresh blueberries.
The findings of the current study are consistent with previous human research15,16 where extension of the postprandial glycaemic response was observed for mixed berry and cranberry interventions respectively. Here we have demonstrated an effect for blueberries, and have shown that the effect is not due to the presence of fructose as previously reported,18 but is likely related to anthocyanin content, or other similar polyphenol phytonutrients also present. Although the study did not analyse the full polyphenol profile of the blueberry intervention, or record metabolomic data following consumption, doses were matched to a previous study that reported postprandial increases in polyphenol metabolites following blueberry consumption,25 thereby notionally supporting this assumption.
Unlike many previous studies,15–17,19 we did not observe a statistically significant attenuation of peak glucose during the immediate postprandial period of 15–30 minutes, despite the apparent differences in peak height shown in Fig. 1. A possible explanation is that the intervention drinks were consumed after a 2 hours fast period in the absence of any starchy food. Previous studies included additional food items, therefore attenuation of peak glucose may be more noticeable in the presence of a high sucrose16 or more complex carbohydrate load.17,19 Our findings may, in part, also be due to the mediating effect of BMI on postprandial glucose response, as reflected by the significance of BMI as a covariate in this analysis. Postprandial glucose response has been shown to be associated with BMI33,34 and the issue of individual variability in glucose response has been previously highlighted.21,33 Future studies should consider whether the effects of anthocyanin-rich foods on postprandial metabolism are mediated by BMI; it is possible that overweight adults with high levels of subcutaneous fat may show a greater reduction in peak glucose following an anthocyanin-rich intervention relative to healthy normal weight adults; however this hypothesis could not be tested in our small sample of healthy young adults. Additionally, individual differences in the time taken to consume the drink may also have influenced the outcome. Ten minutes were allowed, but some participants consumed the drinks considerably faster than this (range 0.5–10 minutes). Peak glucose may have occurred prior to the onset of postprandial testing, or between the earlier testing time points for some of the participants, particularly following rapid absorption of glucose in the sugar-matched control which contained free sugars not bound with other nutrients. Thus, mean glucose peak may not have accurately reflected the true value for the control condition. Future studies should impose tighter control over the time taken to consume an intervention, and consider the use of continuous glucose monitoring to identify true peaks.
In order to minimise the influence of habitual polyphenol consumption, participants were required to follow a low polyphenol diet for 24 hours prior to each test session, however they were only required to fast for 2 hours immediately beforehand. Future research should consider including further dietary controls such as a standardised evening meal and a minimum 8 hours fast prior to baseline measures of blood glucose.
Another important consideration here is that the drinks were not matched for fibre content. Fibre is known to influence postprandial glucose response. For example, guar gum and sugar beet fibre (both non-starch polysaccharides) have been observed to attenuate peak postprandial blood glucose levels and reduce overall glucose AUC.35 However, the maintenance of raised glucose over a longer postprandial period, as observed in the current study, has not been previously associated with a high fibre intervention. It has been posited that it is predominantly soluble fibre that attenuates postprandial glucose through viscosity effects.16 As berries are typically low in soluble fibre and no significant attenuation of peak glucose was observed, the extended postprandial response following blueberry supplementation observed here is unlikely due to fibre confounds. Alternatively, anthocyanins have been observed to inhibit intestinal alpha-amylase and alpha-glucosidase activity in vitro,11,17,36,37 thereby slowing the rate of carbohydrate digestion. Furthermore, anthocyanins and other berry polyphenols have also been associated, in vivo, with inhibition of glucose transport from the intestine to blood plasma.11 Specific mechanisms, identified in vitro, include delayed intestinal absorption of glucose through inhibition of the sodium glucose co-transporter SGLT1,19,38 and the glucose transporter GLUT2.39,40 Therefore, any combination of these mechanisms is likely to underlie the immediate postprandial effects observed here and these potential mechanisms of action warrant further investigation in future studies.
There are a number of important implications for this work. Numerous cognitive benefits of flavonoid-rich foods, including foods rich in anthocyanins, have been previously documented including reduced risk of neurodegenerative disease, and short term improvements to cognition during the immediate postprandial period. It is possible that previously observed cognitive effects, following acute intervention with anthocyanin-rich foods, are mediated by variations in blood glucose levels rather than, or in addition to, other hypothesised mechanisms of action such as increased cerebral blood flow (CBF).41 Increased glucose availability in the late postprandial period following ingestion of anthocyanin-rich foods may convey a cognitive advantage, given that low circulating glucose levels are often correlated with cognitive deficits. Indeed, it has been demonstrated that foods which elicit a favourable glycaemic response are beneficial for cognition,42 and such effects may even extend beyond the first postprandial response period, continuing to influence cognition after subsequent food intake.43 Therefore, it is possible that circulating anthocyanin metabolites may also induce 2nd meal effects, which is an important consideration for intervention studies where a standardised meal is provided prior to extended cognitive testing time points. Further work is needed to confirm whether effects on glycaemic response, such as the effects following anthocyanin-rich blueberry observed here, correlate with changes in cognitive performance.
The present data may also have implications for type 2 diabetes where regulation of postprandial glucose response is important in the prevention and treatment of the disease, and in the reduction of associated risk factors such as cardiovascular disease.1 Glucoregulatory effects similar to the current study have been reported following the consumption of low glycaemic index foods, which are reported to reduce incidence of hypoglycaemia and type 2 diabetes.32 It should be noted, however, that no hypoglycaemic episodes were observed following the control intervention in the current study, suggesting that these benefits may only be relevant in subjects with pre- or type 2 diabetes, rather than in healthy young adults. Nevertheless, anthocyanin-rich blueberries have been positively associated with multiple health outcomes including enhanced cognition,26,27 decreased blood pressure,44 and improved vasoreactivity,25 all of which are compromised in type 2 diabetes.2,3 Furthermore, anthocyanins, and particularly blueberry consumption, are significantly associated with lower type 2 diabetes mellitus risk.12,13 In addition to the regulatory mechanisms described previously, anthocyanins have also been reported, in vitro, to further inhibit alpha-glucosidase activity in synergy with Acarbose,45 a common anti-diabetic drug. Other reviewed blueberry effects on metabolic syndrome include evidence of a lowering of leptin concentrations thereby decreasing the tendency towards obesity, improved pancreatic beta cell function facilitating insulin secretion,46 and subsequent improved insulin sensitivity.9 Other flavonoid subclasses have also been observed, in vitro, to regulate tissue and cell specific isoforms of glucose transporters (GLUTs1–5),5 the rate determining step for glucose uptake by all cells including transport of glucose across the blood–brain barrier.47 Additional evidence also suggests that anthocyanin-rich berries may inhibit glycative activity, where glucose interacts with proteins and lipids in both exogenous food sources and endogenous body tissue.48 The reactive products of such activity (AGEs) have been linked with both hyperglycaemia and cognitive decline. Cognitive outcomes have not been assessed in the current study, however these mechanisms would support enhancement of cognition in type 2 diabetes following anthocyanin-rich intervention. The combined evidence suggests there is clear potential for a diet-based blueberry intervention to benefit the risk factors and co-morbidities associated with type 2 diabetes.
In conclusion, anthocyanin-rich blueberry influenced the postprandial glucose response by extending the availability of blood glucose in a dose-dependent manner. Possible mechanisms of action have been discussed alongside possible implications for cognitive and clinical research.
Conflict of Interest
There are no conflicts of interest to declare.
Acknowledgements
This work was supported by the Economic and Social Research Council (ESRC) [grant number 1371582]. Freeze dried blueberry powder was supplied without charge by the Wild Blueberry Association of North America (WBANA). Neither ESRC nor WBANA had a role in the design, analysis or writing of this article.
References
- E. Bonora and M. Muggeo, Postprandial blood glucose as a risk factor for cardiovascular disease in Type II diabetes: The epidemiological evidence, Diabetologia, 2001, 44, 2107–2114 CrossRef CAS PubMed.
- C.-C. Chung, D. Pimentel, A. J. Jor'dan, Y. Hao, W. Milberg and V. Novak, Inflammation-associated declines in cerebral vasoreactivity and cognition in type 2 diabetes, Neurology, 2015, 85, 450–458 CrossRef CAS PubMed.
- D. J. Lamport, C. L. Lawton, M. W. Mansfield and L. Dye, Impairments in glucose tolerance can have a negative impact on cognitive function: A systematic research review, Neurosci. Biobehav. Rev., 2009, 33, 394–413 CrossRef CAS PubMed.
- H. C. S. Muela, V. A. Costa-Hong, M. S. Yassuda, N. C. Moraes, C. M. Memória, M. F. Machado, T. A. Macedo, E. B. S. Shu, A. R. Massaro, R. Nitrini, A. J. Mansur and L. A. Bortolotto, Hypertension Severity Is Associated With Impaired Cognitive Performance, J. Am. Heart Assoc., 2017, 6, e004579 CrossRef PubMed.
- L. H. Cazarolli, L. Zanatta, E. H. Alberton, M. S. Reis Bonorino Figueiredo, P. Folador, R. G. Damazio, M. G. Pizzolatti and F. R. Mena Barreto Silva, Flavonoids: cellular and molecular mechanism of action in glucose homeostasis, Mini-Rev. Med. Chem., 2008, 8, 1032–1038 CrossRef CAS PubMed.
-
M. Zia Ul Haq, M. Riaz and B. Saad, Anthocyanins and Human Health: Biomolecular and therapeutic aspects, Springer International Publishing, 2016 Search PubMed.
- K. Hanhineva, R. Torronen, I. Bondia-Pons, J. Pekkinen, M. Kolehmainen, H. Mykkänen and K. Poutanen, Impact of dietary polyphenols on carbohydrate metabolism, Int. J. Mol. Sci., 2010, 11, 1365–1402 CrossRef CAS PubMed.
- R. A. S. Sancho and G. M. Pastore, Evaluation of the effects of anthocyanins in type 2 diabetes, Food Res. Int., 2012, 46, 378–386 CrossRef CAS.
- S. Norberto, S. Silva, M. Meireles, A. Faria, M. Pintado and C. Calhau, Blueberry anthocyanins in health promotion: A metabolic overview, J. Funct. Foods, 2013, 5, 1518–1528 CrossRef CAS.
- G. Williamson, Possible effects of dietary polyphenols on sugar absorption and digestion, Mol. Nutr. Food Res., 2013, 57, 48–57 CAS.
- T. Hanamura, C. Mayama, H. Aoki, Y. Hirayama and M. Shimizu, Antihyperglycemic effect of polyphenols from Acerola (Malpighia emarginata DC.) fruit, Biosci., Biotechnol., Biochem., 2006, 70, 1813–1820 CrossRef CAS PubMed.
- A. Jennings, A. A. Welch, T. Spector, A. Macgregor and A. Cassidy, Intakes of Anthocyanins and Flavones Are Associated with Biomarkers of Insulin Resistance and Inflammation in Women, J. Nutr., 2014, 144, 202–208 CrossRef CAS PubMed.
- N. M. Wedick, A. Pan, A. Cassidy, E. B. Rimm, L. Sampson, B. Rosner, W. Willett, F. B. Hu, Q. Sun and R. M. Van Dam, Dietary flavonoid intakes and risk of type 2 diabetes in US men and women, Am. J. Clin. Nutr., 2012, 95, 925–933 CrossRef CAS PubMed.
- B. Burton-Freeman, Postprandial metabolic events and fruit-derived phenolics: a review of the science, Br. J. Nutr., 2010, 104(Suppl), S1–14 CrossRef CAS PubMed.
- T. Wilson, A. P. Singh, N. Vorsa, C. D. Goettl, K. M. Kittleson, C. M. Roe, G. M. Kastello and F. R. Ragsdale, Human glycemic response and phenolic content of unsweetened cranberry juice, J. Med. Food, 2008, 11, 46–54 CrossRef CAS PubMed.
- R. Törrönen, E. Sarkkinen, N. Tapola, E. Hautaniemi, K. Kilpi and L. Niskanen, Berries modify the postprandial plasma glucose response to sucrose in healthy subjects, Br. J. Nutr., 2010, 103, 1094–1097 Search PubMed.
- H. Nyambe-Silavwe and G. Williamson, Polyphenol- and fibre-rich dried fruits with green tea attenuate starch-derived postprandial blood glucose and insulin: a randomised, controlled, single-blind, cross-over intervention, Br. J. Nutr., 2016, 116, 443–450 CrossRef CAS PubMed.
- C. D. Kay and B. J. Holub, The effect of wild blueberry (Vaccinium angustifolium) consumption on postprandial serum antioxidant status in human subjects, Br. J. Nutr., 2002, 88, 389–397 CrossRef CAS PubMed.
- J. Hidalgo, C. Flores, M. A. Hidalgo, M. Perez, D. D. Caceres and R. A. Burgos, Delphinol® standardized maqui berry extract reduces postprandial blood glucose increase in individuals with impaired glucose regulation by novel mechanism Sodium Glucose Cotransporter Inhibition, Panminerva Med., 2014, 56, 1–7 CAS.
- G. Cao, R. M. Russell, N. Lischner and R. L. Prior, Human Nutrition and Metabolism Serum Antioxidant Capacity Is Increased by Consumption of Strawberries, Spinach, Red Wine or Vitamin C in Elderly Women, J. Nutr., 1998, 128, 2383–2390 CAS.
- J. A. Vinson, P. Bose, J. Proch, H. Al Kharrat and N. Samman, Cranberries and cranberry products: Powerful in vitro, ex vivo, and in vivo sources of antioxidants, J. Agric. Food Chem., 2008, 56, 5884–5891 CrossRef CAS PubMed.
- M. E. Clegg, M. Pratt, C. M. Meade and C. J. K. Henry, The addition of raspberries and blueberries to a starch-based food does not alter the glycaemic response, Br. J. Nutr., 2011, 106, 335–338 CrossRef CAS PubMed.
- I. Edirisinghe, K. Banaszewski, J. Cappozzo, K. Sandhya, C. L. Ellis, R. Tadapaneni, C. T. Kappagoda and B. M. Burton-Freeman, Strawberry anthocyanin and its association with postprandial inflammation and insulin, Br. J. Nutr., 2011, 106, 913–922 CrossRef CAS PubMed.
- R. M. Alqurashi, L. A. Galante, I. R. Rowland, J. P. E. Spencer and D. M. Commane, Consumption of a flavonoid-rich acai meal is associated with acute improvements in vascular function and a reduction in total oxidative status in healthy overweight men, Am. J. Clin. Nutr., 2016, 104, 1227–1235 CrossRef CAS PubMed.
- A. Rodriguez-Mateos, C. Rendeiro, T. Bergillos-meca, S. Tabatabaee, T. W. George, C. Heiss and J. P. E. Spencer, Intake and time dependence of blueberry flavonoid – induced improvements in vascular function: a randomized, controlled, double-blind, crossover intervention study with mechanistic insights into biological activity, Am. J. Clin. Nutr., 2013, 98, 1179–1191 CrossRef CAS PubMed.
- L. Bell, D. J. Lamport, L. T. Butler and C. M. Williams, A review of the cognitive effects observed in humans following acute supplementation with flavonoids, and their associated mechanisms of action, Nutrients, 2015, 7, 10290–10306 CrossRef CAS PubMed.
- D. J. Lamport, L. Dye, J. D. Wightman and C. L. Lawton, The effects of flavonoid and other polyphenol consumption on cognitive performance: A systematic research review of human experimental and epidemiological studies, Nutr. Ageing, 2012, 1, 5–25 Search PubMed.
- B. R. Cutler, C. Petersen and P. V. Anandh Babu, Mechanistic insights into the vascular effects of blueberries: Evidence from recent studies, Mol. Nutr. Food Res., 2017, 61, 1600271 Search PubMed.
- T. C. Wallace, M. Slavin and C. L. Frankenfeld, Systematic review of anthocyanins and markers of cardiovascular disease, Nutrients, 2016, 8, 1–13 Search PubMed.
- J.-P. Le Floch, P. Escuyer, E. Baudin, D. Baudon and L. Perlemuter, Blood Glucose Area Under the Curve: Methodological Aspects, Diabetes Care, 1990, 13, 172–175 CrossRef CAS PubMed.
- A. D. Association, Diagnosis and classification of diabetes mellitus, Diabetes Care, 2014, 37, 81–90 CrossRef PubMed.
- W. Willett, J. Manson and S. Liu, Glycemic index, glycemic load, and risk of type 2 diabetes, Am. J. Clin. Nutr., 2002, 76, 274S–280S CAS.
- J. F. Carroll, K. A. Kaiser, S. F. Franks, C. Deere and J. L. Caffrey, Influence of BMI and gender on postprandial hormone responses, Obesity, 2007, 15, 2974–2983 CrossRef CAS PubMed.
- M. K. Hopper, C. E. Koch and J. L. Koch, Postprandial Blood Glucose Response to Meals of Varying Composition; Influence of BMI and Physical Fitness, FASEB J., 2013, 27, 1192 Search PubMed.
- L. M. Morgan, J. A. Tredger, J. Wright and V. Marks, The effect of soluble- and insoluble-fibre supplementation on post-prandial glucose tolerance, insulin and gastric inhibitory polypeptide secretion in healthy subjects, Br. J. Nutr., 1990, 64, 103–110 CrossRef CAS PubMed.
- K. Tadera, Y. Minami, K. Takamatsu and T. Matsuoka, Inhibition of alpha-glucosidase and alpha-amylase by flavonoids, J. Nutr. Sci. Vitaminol., 2006, 52, 149–153 CrossRef CAS PubMed.
- G. J. McDougall, F. Shpiro, P. Dobson, P. Smith, A. Blake and D. Stewart, Different Polyphenolic Components of Soft Fruits Inhibit Alpha-Amylase and Alpha-Glucosidase, J. Agric. Food Chem., 2005, 53, 2760–2766 CrossRef CAS PubMed.
- K. Johnston, P. Sharp, M. Clifford and L. Morgan, Dietary polyphenols decrease glucose uptake by human intestinal Caco-2 cells, FEBS Lett., 2005, 579, 1653–1657 CrossRef CAS PubMed.
- J. Song, O. Kwon, S. Chen, R. Daruwala, P. Eck, J. B. Park and M. Levine, Flavonoid inhibition of sodium-dependent vitamin C transporter 1 (SVCT1) and glucose transporter isoform 2 (GLUT2), intestinal transporters for vitamin C and glucose, J. Biol. Chem., 2002, 277, 15252–15260 CrossRef CAS PubMed.
- O. Kwon, P. Eck, S. Chen, C. P. Corpe, J.-H. Lee, M. Kruhlak and M. Levine, Inhibition of the intestinal glucose transporter GLUT2 by flavonoids, FASEB J., 2007, 21, 366–377 CrossRef CAS PubMed.
- C. Rendeiro, J. S. Rhodes and J. P. E. Spencer, The mechanisms of action of flavonoids in the brain: Direct versus indirect effects, Neurochem. Int., 2015, 89, 126–139 CrossRef CAS PubMed.
- D. J. Lamport, C. L. Lawton, M. W. Mansfield, C. A. J. Moulin and L. Dye, Type 2 diabetes and impaired glucose tolerance are associated with word memory source monitoring recollection deficits but not simple recognition familiarity deficits following water, low glycaemic load, and high glycaemic load breakfasts, Physiol. Behav., 2014, 124, 54–60 CrossRef CAS PubMed.
- D. J. Lamport, E. Hoyle, C. L. Lawton, M. W. Mansfield and L. Dye, Evidence for a second meal cognitive effect: glycaemic responses to high and low glycaemic index evening meals are associated with cognition the following morning, Nutr. Neurosci., 2011, 14, 66–71 CrossRef CAS PubMed.
- A. Basu, M. Du, M. J. Leyva, K. Sanchez, N. M. Betts, M. Wu, C. E. Aston and T. J. Lyons, Blueberries Decrease Cardiovascular Risk Factors in Obese Men and Women with Metabolic Syndrome, J. Nutr., 2010, 140, 1582–1587 CrossRef CAS PubMed.
- S. Akkarachiyasit, P. Charoenlertkul, S. Yibchok-Anun and S. Adisakwattana, Inhibitory activities of cyanidin and its glycosides and synergistic effect with acarbose against intestinal α-glucosidase and pancreatic α-amylase, Int. J. Mol. Sci., 2010, 11, 3387–3396 CrossRef CAS PubMed.
- L. C. Martineau, A. Couture, D. Spoor, A. Benhaddou-Andaloussi, C. Harris, B. Meddah, C. Leduc, A. Burt, T. Vuong, P. Mai Le, M. Prentki, S. A. Bennett, J. T. Arnason and P. S. Haddad, Anti-diabetic properties of the Canadian lowbush blueberry Vaccinium angustifolium Ait, Phytomedicine, 2006, 13, 612–623 CrossRef CAS PubMed.
- S. J. Vannucci, F. Maher and I. A. Simpson, Glucose transporter proteins in brain: Delivery of glucose to neurons and glia, Glia, 1997, 21, 2–21 CrossRef CAS PubMed.
- N. Thangthaeng, S. M. Poulose, M. G. Miller and B. Shukitt-Hale, Preserving Brain Function in Aging: The Anti-glycative Potential of Berry Fruit, NeuroMol. Med., 2016, 18, 465–473 CrossRef CAS PubMed.
|
This journal is © The Royal Society of Chemistry 2017 |