A proposed nomenclature for biological processes that remove nitrogen†
Received
19th August 2016
, Accepted 23rd November 2016
First published on 24th November 2016
Abstract
For development and optimization of novel biological nutrient removal processes, factors affecting energy and greenhouse gas emissions (e.g. nitrous oxide) are increasingly important. Energy efficient pathways are exploited, such as short-cut processes for treatment of concentrated side streams. These pathways are usually well described, but a consistent nomenclature is still lacking: some functionally equivalent processes are referred to using distinct names, and some functionally distinct processes are referred to with the same name. To address this issue, we propose a standardized terminology, in which a root term designates the end product for oxidation pathways or the reactant for reduction pathways; a qualifier is used to designate the predominant oxidation–reduction regime (ORR) and an optional indicator is used to designate partial turnover rates, e.g. partial ammonia oxidation to nitrite.
Water impact
Recent advances in understanding biological nitrogen removal (BNR) processes have resulted in a large variety of different technologies applying short-cuts in the microbial metabolism. These developments and investigations have led to an inconsistency in the applied terminology. To overcome this issue, we proposed a transparent logic and nomenclature that enables distinct determination of every presently known catalyzed reaction in BNR.
|
1 Introduction
Nutrient removal is required to protect water quality and the environment and is thus a primary objective of wastewater treatment. The conventional activated sludge (CAS) process, an approach invented in 1914, has been optimized for removal of carbon (C), nitrogen (N), and phosphorus (P).1,2 While these processes are effective, their energy requirements are high, a fact that has spurred interest in strategies that save energy3,4 and enable resource recovery, avoid greenhouse gas emissions, and decrease operational costs. Wastewater treatment is at a turning point where nutrient removal will be achieved through more sustainable and integrated systems aiming at resource and energy recovery.1
Removal of organic carbon and production of methane by anaerobic digestion of organic waste streams are well established for energy recovery at municipal wastewater treatment plants. Typically, these waste streams contain high levels of nitrogen, and a consequence is the release of high concentrations of ammonia in the digester's effluents and in the reject water from sludge dewatering, respectively. Returning these concentrated streams to the headworks imposes an additional nitrogen load on the mainstream and can potentially drive the CAS to its limits, in which case there is insufficient reducing power for denitrification. To overcome these issues, novel biological nitrogen removal (BNR) processes have been developed in the last decade. These include processes like Stable High Ammonia Removal Over Nitrite (SHARON®),5Complete Autotrophic Nitrogen removal Over Nitrite (CANON)6 and ANaerobic AMMonium OXidation (ANAMMOX®).7 All of these technologies are characterized by the use of a short-cut via nitrite (NO2−) pathway of microbial metabolism. Such a pathway can reduce the oxygen and carbon demands for reducing power and increasing potential for energy recovery compared to CAS. Although these technical applications have primarily been applied to side stream BNR, ongoing research is presently directed to investigating mainstream implementation as well.8,9 Despite their energy benefits, these processes could potentially release significant GHG emissions such as nitrous oxide (N2O), when compared to conventional BNR processes10–13 (N2O has a global warming potential 310 times higher than that of CO2).14 Research is on-going to prevent greenhouse gas emissions through improved process stability and control.15
Novel alternative strategies are also being developed and came to the fore recently. One approach consists of bioelectrochemical hybrid-systems to substitute the necessary amount of carbon with an electrical current to gain sufficient reducing power.16,17 Another strategy is nitrogen removal coupled with energy recovery. In this respect, the Coupled Aerobic-Anoxic Nitrous Decomposition Operation18,19 (CANDO) has been proposed for intentional N2O production and its subsequent use as an energy-yielding co-oxidant for biogas combustion. The CANDO process might also facilitate P removal and biopolymer production.19
The opportunities provided by these state-of-the-art treatment technologies are presently of high scientific relevance underscored by a growing number of peer-reviewed journal articles in this domain. As an example, publications focusing on nitritation (oxidation of ammonia (NH4+) to nitrite, NH4+ → NO2−) and denitritation (reduction of nitrite to nitrogen gas (N2), NO2− → N2) peaked in 2015 with a share of ∼25% within the total publication number from 2006–2015. The results were obtained from an online research in the Web of Science™ Core Collection (Fig. S1 in ESI†). These studies revealed several novel reaction mechanisms and pathways. Some of them were defined coincidentally by different authors resulting in partial ambiguity in the chosen terminology. Two expressions related to N2O emissions serve as an example of ambiguous terminology. The first is “nitrifier denitrification”, a phrase originally coined to describe unintended N2O generation when NO2− is reduced via nitric oxide (NO).20 Problems become clear when this phrase is parsed into “nitrifier” and “denitrification”. The term “nitrifier” is problematic, because it implies active nitrite-oxidizing bacteria (NOB) as a potential key-player in the production of N2O. So far, the presence of both NH4+ and NO2− oxidation related genes in a single organism has been reported for only two Nitrospira species by van Kessel et al.,21 indicating that there are organisms engaging in both pathways. However, production of N2O during the oxidation of NO2− to NO3−, accounting for most NOB, is not known. The use of the term “denitrification” is also problematic because it implies complete denitrification to N2. The second example is “partial denitrification”.19 This phrase refers to production of N2O as the end product of denitrification or to conversion of NO3− to NO2− and possibly also a partial conversion of NO2− or NO3− to N2. Phrases such as “nitrifier denitrification” and “partial denitrification” are thus imprecise and do not adequately differentiate pathways and products. Both expressions demonstrate that denotation has become imprecise and underscore the need for a consistent terminology that differentiates pathways to avoid misunderstanding in the scientific community.
We propose here a conservative consolidation of existing terminology by including new definitions based upon a comprehensive literature review and transparent logic. The goal is to provide a combination of consistent determinants properly representing distinct reaction conditions and underlying processes. We do not claim that the approach in its present form is perfect, but we hope that our contribution could engage a discussion in the scientific community towards a standardization of the terminology facilitating communication in the future.
2 Scientific approach
We begin by compiling and comparing current terminology for all known BNR metabolic pathways in terms of singularity and distinctiveness. We then propose terminology that establishes a uniform nomenclature for biotechnology and process engineering. Our aim is to integrate novel elements into historically accepted terminology (such as “nitrification” and “denitrification”) so as to establish an unambiguous nomenclature. Names for oxidation sequences are formulated by identifying a root term based upon the reaction product. Oxidation of NH4+ to NO2− is therefore designated “NITRITation”; oxidation of NO2− to NO3− is designated “NITRATation”. Analogous historical logic is applied for denitrification, with preservation of the prefix “de” for reduction sequences. In this case, however, the focus is on the initial reactant. Reduction of NO3− to NO2− is designated “DENITRATation”; reduction of NO2− to N2 is designated “DENITRITation”. With respect to NO2−, the metabolic intermediates, i.e. nitric oxide and nitrous oxide, require an additional indicator – the final reaction product – in order to differentiate them from nitrogen removal via N2.
For determination of reaction pathways, mechanisms and dominant organisms, the oxidation–reduction regime (ORR) is used as a qualifier. We initially considered ranges of dissolved oxygen (DO) concentration for each ORR, but, to the best of our knowledge, specific threshold values have yet to be defined, except for the fully anoxic case where DO is zero. Further research is needed to elucidate the DO ranges for oxidoreductase activities and DO profiles within biofilms so as to differentiate such processes from the mean DO values commonly applied to the bulk mixed liquor. For now, we define just two ranges within the DO constrained ORRs: (1) low (sub-oxic) and (2) high DO (aerobic). Finally, “partial” is applied as an optional second determinant to designate incomplete conversion of reactants. An example would be “partial nitritation” for the case where <100% of the initial NH4+ load is converted to NO2−. Based on these results, the logical combination of our terminology consists of two highly recommended and one optional quantitative indicator:
Oxidation: quantitative indicator* + ORR + reaction pathway (product specific)
Reduction: quantitative indicator* + ORR + reaction pathway (educt specific)
*only if necessary
This approach represents a flexible nomenclature for complete integration of the presently relevant processes and to potentially derive the terms for novel treatment steps in the future.
3 Proposed terminology
3.1 Pathways of conventional BNR processes
Mainstream nitrification/denitrification (N/DN) processes are still the most widely applied techniques for nitrogen removal in conventional biological wastewater treatment. The underlying pathways are complete oxidation of NH4+ during nitrification and reduction of NO3− to N2via denitrification (Fig. 1) resulting in NO3− and N2 as desired products, respectively. The underlying pathways are well understood and have been the basis for design and operation of conventional BNR processes for decades:22 | Nitrification(aerobic oxidation): NH4+ → NH2OH → NO2− → NO3− → N2O | (1) |
| Denitrification (anoxic reduction): NO3− → NO2− → NO → N2O → N2 | (2) |
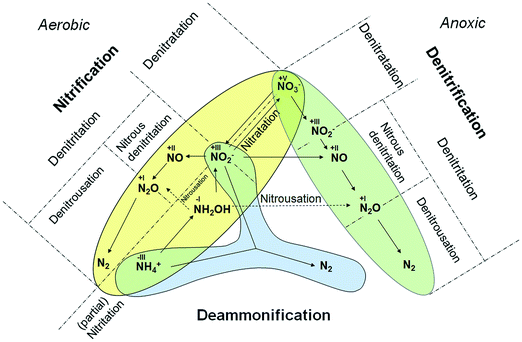 |
| Fig. 1 Schematic representation of the proposed terminology. The continuously lined arrows indicate microbially catalyzed reaction pathways, the dashed lined arrows chemical reactions, reaction pathways are indicted by dash-dotted lines. In yellow: The aerobic and sub-oxic reaction pathways summarized in the “aerobic” regime. In green: Reductive pathways including the intermediate steps in nitrite reduction to N2O. Denitrousation is included for both aerobic and anoxic regimes with respect to the mitigation of N2O emissions. In blue: The deammonification pathway. | |
Within N/DN, some metabolites are shared intermediates for oxidation and reduction pathways. The major intermediates are nitrite (NO2−), nitrate (NO3−) and nitrous oxide (N2O) (eqn (1) and (2)). During nitrification, N2O is both a stable by-product of chemical oxidation via hydroxylamine (NH2OH)20 and a product of microbial denitrification pathways. Fig. 1 provides an overview of the discussed microbial pathways together with the proposed nomenclature. Nitric oxide (NO) can also be generated chemically by disproportionation of nitrous acid (HNO2) in wastewater treatment systems, however, appreciable quantities are only released under acidic conditions23 and such conditions are uncommon in BNR systems.
3.2 Role of the ORR
The key process parameter commonly manipulated to control reaction pathways in engineered BNR processes is the DO, a parameter that sets the predominant ORR.24–26 The ORR in BNR processes can typically be characterized as aerobic (oxidation pathways), anoxic (reduction pathways), and sub-oxic (reduction and oxidation pathways occur simultaneously26). These categories capture the predominant biochemical dynamics within a particular process. The ORR also constrains and defines the structure and function of the microbial community and dominance of key organisms. Ammonia oxidizing bacteria (AOB) and nitrite oxidizing bacteria are predominant under aerobic conditions; denitrifying bacteria (DB) are predominant under anoxic conditions. Under sub-oxic conditions, a process phase quite typical for contemporary treatment technologies, such as the SHARON or CANON processes, AOB are the dominant organisms27,28 and can catalyze N reduction reactions.20,29
3.3 Pathways of novel BNR processes
In contrast to the terminology used for conventional nitrification/denitrification, the terminology that is used to describe metabolic pathways in contemporary BNR processes is diverse and inconsistent. The typical first step is the oxidation of NH4+ to NO2−. Nitrite is the precursor for subsequent reduction in several well-established processes (e.g., denitritation, SHARON®, CANON, DEMON® and ANITAMOX®). Nitrogen elimination as N2 is carried out by anaerobic ammonia oxidizing (so-called Anammox) bacteria8 (planctomycetes) or by DB.30 Frequently, these treatments are carried out simultaneously in single-stage systems.31,32 From a biochemical point-of-view, however, the seemingly “simultaneous” reactions are a chain of oxidation and reduction reactions at different micro-scale ORRs.
3.3.1 Aerobic ORR.
In contemporary practice of aerobic and sub-oxic systems, the adjective “full” refers to processes that are designed to achieve essentially 100% conversion of reactants (e.g. NH4+) to products (e.g. NO2−, NO3−). In contrast, the adjective “partial”33–35 is used to refer to processes designed to achieve less than 100% conversion30,36,37e.g. “partial nitritation” or is used to differentiate short-cut technologies from conventional treatment e.g. “partial nitrification” (NH4+ → NO2− instead of NH4+ → NO3−) (Table 1, eqn (3.a) and (3.b)). Frequently, the intended use is not specified and has to be assessed by the context of the particular publication. In order to enable a distinctive definition, we propose use of the word “partial” to designate all processes that bring about partial oxidation or reduction of educts, respectively.
Table 1 Literature compilation of BNR steps, according to ORR (A: aerobic, S: sub-oxic, An: anoxic), change in the oxidation state of nitrogen (O: oxidation, R: reduction) and currently used terminology with qualitative indicators. The same background color represents ambiguity; italicized letters represent imprecise terms; and adopted terms are listed in bold green font)
Oxidation of nitrite to nitrate (Table 1, eqn (4)), a typical side reaction in nitritation processes,38,39 is carried out by nitrite oxidizing bacteria (NOB) via “nitratation”.40 This terminology is in accordance with the proposed scheme and is adopted as is. In contrast, generation of N2O via NH2OH under aerobic and sub-oxic conditions, an important reaction for greenhouse gas emissions at wastewater treatment plants11,14,41 and energy recovery options,18,19 does not follow an agreed-upon terminology (Table 1, eqn (5)). Processes that generate N2O clearly need better definition. Investigations by Daelman et al.11 revealed that the share of N2O within the total carbon footprint can be as high as 78%. However, N2O is also a potential energy recovery option as co-oxidant in biogas combustion processes.18 To systematize the nomenclature, we propose the term “nitrousation” for its aerobic microbial generation of N2O (Fig. 1).
3.3.2 Anoxic ORR.
For the reductive pathways, historically the prefix “de-” has been commonly used (e.g., “Denitrification”). To be conservative, we propose to generally maintain the logic, i.e. initial nitrogen compound plus prefix “de-”. This will result in two terms, “denitratation” (Table 1, eqn (6)) and “denitritation” (Table 1, eqn (7)), which have already been applied in this context and are adopted for the novel terminology framework.
In studies by Scherson et al.,18,19 an anoxic carbon-limited biochemical reaction pathway for N2O production (Table 1, eqn (8)) has been referred to as “partial denitrification”. Considering the historical interpretation of “denitrification”, the expression is misleading as it relates to the production of N2. However, applying the scheme for reductive pathways to the CANDO process causes the problem that “denitritation” already refers to nitrogen removal via N2. As a qualitative indicator for a clear product specification, we propose “nitrous” in addition – resulting in the expression “nitrous denitritation” (Fig. 1).
Independent from N/DN, the oxidation reduction reaction carried out under anoxic conditions by planctomycetes is known as “deammonification”42–44 as well as “anaerobic ammonium oxidation”13,33,45–48 or “ANAMMOX®” (Table 1, eqn (9)). The process is based on an alternative pathway49 in the biological nitrogen metabolism and decoupled from the reaction chains in N/DN. In contrast to the potential bi-directional reaction pathways in N/DN, deammonification is uni-directional (Fig. 1). In contrast to nitritation or denitritation, deammonification is an intracellular reaction pathway without any transition links within the metabolic web. Due to its isolation, the one-directional redox-reaction and lack of available intermediates, the process is integrated independently (Fig. 1). However, since ANAMMOX® is a licensed process by Paques (EL Balk, NL), we continue using the term “deammonification” in order to distinguish between the technical process as a registered trademark and the underlying metabolic pathway.
3.3.3 Sub-oxic ORR.
During sub-oxic conditions, AOB catalyze reduction and oxidation reactions “simultaneously”.50 Depending upon reactor conditions, different products can be produced under reductive conditions (i.e., NO, N2O, N2). The production of N2O in this respect represents the same reaction pathway as in the second stage of the CANDO process (Table 1, eqn (10)), but the mechanism is related to the sensitivity of nitrous oxide reductase to oxygen51–53 instead of carbon limitation. A commonly used phrase in this context is “nitrifier denitrification”,20,29,54,55 but with respect to the microbial community as well the terminal reduction step, this expression is misleading. The term “nitrifier” implies the participation of nitrite oxidizing microorganisms, and denitrification historically implies N2 as the final product.22 In order to specify this particular reaction pathway, the term “nitrous denitritation” is proposed. To distinguish between the CANDO process and this reaction, a proper definition of the ORR is necessary. According to the previous explanations, the determinant “sub-oxic” is adequate resulting in “sub-oxic nitrous denitritation”.
A comparison of Gong et al.56 and Scherson et al.18,19 elucidates another ambiguous expression. Both authors use the phrase “partial denitrification”, but very different processes are described: Scherson et al.18,19 refer to N2O production, while Gong et al.56 refer to sub-oxic reduction of NO3− to NO2− (Table 1, eqn (11)). According to our proposed scheme, the pathway of Gong et al. can be distinctly described as “sub-oxic denitratation”. Moreover, because the dominant organisms (i.e. AOB and NOB) under sub-oxic conditions are the same as under “aerobic” conditions, it is clear that the reduction reaction mechanisms are related to “aerobic” organisms and the “sub-oxic” determinant can thus be omitted from the terminology.
4 Conclusion
This work has shown that the structure of different combinations of metabolic pathways applied in BNR processes has become more complex and inconsistent, leading to ambiguity and confusion within the research community and industry. For future communication and development of BNR processes, we therefore propose to apply a consolidated terminology that enables a more exact definition of the metabolic pathways.
The presented approach enables a distinct identification of every possible step that is important for BNR technologies (Fig. 1). Because of its flexibility it can also be adapted for novel derivatives of state-of-the-art BNR technologies. For a process removing nitrogen as nitric oxide, for example, application of the proposed nomenclature would give the phrase “nitric denitritation”. For the reduction of NO3− to N2O “nitrous denitrification” can be derived. While such processes have not been technically applied yet, it illustrates the flexibility of the terminology. Additionally, presently emerging bioelectrochemical technologies also fit into the scheme, since their catalyzed oxidation reduction reactions are the same as in biological systems. Hence, with the adoption of our presented approach a more exact communication about all known biological and bioelectrochemical technologies can be facilitated and will potentially avoid further misunderstanding. However, we do not claim that the presented approach is perfect, but it hopefully triggers further discussion within the community to agree on a commonly accepted terminology.
Acknowledgements
The International Graduation School of Science and Engineering (IGSSE) of TUM is gratefully acknowledged for financial support (Project Water 06, PANOWA). Furthermore, we appreciate the support of the Bavaria California Technology Center (BaCaTeC) for supporting the scientific exchange between our two institutions (No. 22 [2013-2]).
References
-
C. L. Grady Jr, G. T. Daigger, N. G. Love and C. D. M. Filipe, Biological wastewater treatment, CRC press, 2011 Search PubMed.
-
D. Jenkins and J. Wanner, Activated Sludge–100 Years and Counting, Water Intelligence Online, 2014, vol. 13, p. 9781780404943 Search PubMed.
- J. D. Englehardt, T. Wu, F. Bloetscher, Y. Deng, P. Du Pisani, S. Eilert, S. Elmir, T. Guo, J. Jacangelo, M. LeChevallier, H. Leverenz, E. Mancha, E. Plater-Zyberk, B. Sheikh, E. Steinle-Darling and G. Tchobanoglous, Net-zero water management: Achieving energy-positive municipal water supply, Environ. Sci.: Water Res. Technol., 2016, 2(2), 250–260, 10.1039/C5EW00204D.
- H. Gao, Y. D. Scherson and G. F. Wells, Towards energy neutral wastewater treatment: methodology and state of the art, Environ. Sci.: Processes Impacts, 2014, 16(6), 1223–1246, 10.1039/c4em00069b.
- A. Galí, J. Dosta, M. C. M. van Loosdrecht and J. Mata-Álvarez, Biological Nitrogen Removal via Nitrite of Reject Water with a SBR and Chemostat SHARON/Denitrification Process, Ind. Eng. Chem. Res., 2006, 45(22), 7656–7660, DOI:10.1021/ie0603565.
- K. A. Third, A. O. Sliekers, J. G. Kuenen and M. S. Jetten, The CANON system (Completely Autotrophic Nitrogen-removal Over Nitrite) under ammonium limitation: interaction and competition between three groups of bacteria, Syst. Appl. Microbiol., 2001, 24(4), 588–596, DOI:10.1078/0723-2020-00077.
- S. Lackner, E. M. Gilbert, S. E. Vlaeminck, A. Joss, H. Horn and M. C. M. van Loosdrecht, Full-scale partial nitritation/anammox experiences--an application survey, Water Res., 2014, 55, 292–303, DOI:10.1016/j.watres.2014.02.032.
- M. Laureni, D. G. Weissbrodt, I. Szivák, O. Robin, J. L. Nielsen, E. Morgenroth and A. Joss, Activity and growth of anammox biomass on aerobically pre-treated municipal wastewater, Water Res., 2015, 80, 325–336 CrossRef CAS PubMed.
- D. T. Tan and D. Shuai, Research highlights: Advances and challenges in developing mainstream anammox treatment, Environ. Sci.: Water Res. Technol., 2015, 1(5), 546–549, 10.1039/C5EW90020D.
- A. Aboobakar, E. Cartmell, T. Stephenson, M. Jones, P. Vale and G. Dotro, Nitrous oxide emissions and dissolved oxygen profiling in a full-scale nitrifying activated sludge treatment plant, Water Res., 2013, 47(2), 524–534, DOI:10.1016/j.watres.2012.10.004.
- M. R. Daelman, E. M. van Voorthuizen, L. van Dongen, E. I. Volcke and M. C. van Loosdrecht, Methane and nitrous oxide emissions from municipal wastewater treatment–results from a long-term study, Water Sci. Technol., 2013, 67(10), 2350–2355 CrossRef CAS PubMed.
- M. Pijuan, J. Torà, A. Rodríguez-Caballero, E. César, J. Carrera and J. Pérez, Effect of process parameters and operational mode on nitrous oxide emissions from a nitritation reactor treating reject wastewater, Water Res., 2014, 49, 23–33, DOI:10.1016/j.watres.2013.11.009.
- C. Domingo-Félez, A. G. Mutlu, M. M. Jensen and B. F. Smets, Aeration strategies to mitigate nitrous oxide emissions from single-stage nitritation/anammox reactors, Environ. Sci. Technol., 2014, 48(15), 8679–8687, DOI:10.1021/es501819n.
- A. R. Ravishankara, J. S. Daniel and R. W. Portmann, Nitrous oxide (N2O): the dominant ozone-depleting substance emitted in the 21st century, Science, 2009, 326(5949), 123–125, DOI:10.1126/science.1176985.
- A. Bartrolí, J. Pérez and J. Carrera, Applying ratio control in a continuous granular reactor to achieve full nitritation under stable operating conditions, Environ. Sci. Technol., 2010, 44(23), 8930–8935, DOI:10.1021/es1019405.
- S. Tejedor-Sanz, T. Bacchetti de Gregoris, J. J. Salas, L. Pastor and A. Esteve-Núñez, Integrating a microbial electrochemical system into a classical wastewater treatment configuration for removing nitrogen from low COD effluents, Environ. Sci.: Water Res. Technol., 2016, 2(5), 884–893, 10.1039/C6EW00100A.
- M. Rodríguez Arredondo, P. Kuntke, A. W. Jeremiasse, T. H. J. A. Sleutels, C. J. N. Buisman and A. ter Heijne, Bioelectrochemical systems for nitrogen removal and recovery from wastewater, Environ. Sci.: Water Res. Technol., 2015, 1(1), 22–33, 10.1039/C4EW00066H.
- Y. D. Scherson, G. F. Wells, S.-G. Woo, J. Lee, J. Park, B. J. Cantwell and C. S. Criddle, Nitrogen removal with energy recovery through N2O decomposition, Energy Environ. Sci., 2013, 6(1), 241–248 CAS.
- Y. D. Scherson, S.-G. Woo and C. S. Criddle, Production of nitrous oxide from anaerobic digester centrate and its use as a co-oxidant of biogas to enhance energy recovery, Environ. Sci. Technol., 2014, 48(10), 5612–5619, DOI:10.1021/es501009j.
- N. Wrage, G. L. Velthof, M. L. van Beusichem and O. Oenema, Role of nitrifier denitrification in the production of nitrous oxide, Soil Biol. Biochem., 2001, 33(12), 1723–1732 CrossRef CAS.
- M. A. H. J. van Kessel, D. R. Speth, M. Albertsen, P. H. Nielsen, H. J. M. Op den Camp, B. Kartal, M. S. M. Jetten and S. Lücker, Complete nitrification by a single microorganism, Nature, 2015, 528(7583), 555–559, DOI:10.1038/nature16459.
-
E. Metcalf, Wastewater Engineering: Treatment, Disposal, Reuse, Metcalf & Eddy. Inc., McGraw-Hill, New York, 2003 Search PubMed.
- F. Schreiber, P. Wunderlin, K. M. Udert and G. F. Wells, Nitric oxide and nitrous oxide turnover in natural and engineered microbial communities: biological pathways, chemical reactions, and novel technologies, Front. Microbiol., 2012, 3, 372 Search PubMed.
- S. Lackner and H. Horn, Evaluating operation strategies and process stability of a single stage nitritation-anammox SBR by use of the oxidation–reduction potential (ORP), Bioresour. Technol., 2012, 107, 70–77, DOI:10.1016/j.biortech.2011.12.025.
- S. Lackner, C. Lindenblatt and H. Horn, ‘Swinging ORP’ as operation strategy for stable reject water treatment by nitritation–anammox in sequencing batch reactors, Chem. Eng. J., 2012, 180, 190–196, DOI:10.1016/j.cej.2011.11.043.
- J. B. Holman and D. G. Wareham, Oxidation–reduction potential as a monitoring tool in a low dissolved oxygen wastewater treatment process, J. Environ. Eng., 2003, 129(1), 52–58 CrossRef CAS.
- P. Wunderlin, H. Siegrist and A. Joss, Online N2O measurement: the next standard for controlling biological ammonia oxidation?, Environ. Sci. Technol., 2013, 47(17), 9567–9568, DOI:10.1021/es402971p.
- W. Zeng, L. Li, Y. Yang, S. Wang and Y. Peng, Nitritation and denitritation of domestic wastewater using a continuous anaerobic-anoxic-aerobic (A(2)O) process at ambient temperatures, Bioresour. Technol., 2010, 101(21), 8074–8082, DOI:10.1016/j.biortech.2010.05.098.
- P. Wunderlin, J. Mohn, A. Joss, L. Emmenegger and H. Siegrist, Mechanisms of N2O production in biological wastewater treatment under nitrifying and denitrifying conditions, Water Res., 2012, 46(4), 1027–1037, DOI:10.1016/j.watres.2011.11.080.
- P. Regmi, B. Holgate, D. Fredericks, M. W. Miller, B. Wett, S. Murthy and C. B. Bott, Optimization of a mainstream nitritation–denitritation process and anammox polishing, Water Sci. Technol., 2015, 72(4), 632–642, DOI:10.2166/wst.2015.261.
- A. Joss, D. Salzgeber, J. Eugster, R. König, K. Rottermann, S. Burger, P. Fabijan, S. Leumann, J. Mohn and H. Siegrist, Full-Scale Nitrogen Removal from Digester Liquid with Partial Nitritation and Anammox in One SBR, Waste Manage., 2009, 43(14), 5301–5306, DOI:10.1021/es900107w.
- A. Joss, N. Derlon, C. Cyprien, S. Burger, I. Szivak, J. Traber, H. Siegrist and E. Morgenroth, Combined nitritation-anammox: advances in understanding process stability, Environ. Sci. Technol., 2011, 45(22), 9735–9742, DOI:10.1021/es202181v.
- S. Okabe, M. Oshiki, Y. Takahashi and H. Satoh, Development of long-term stable partial nitrification and subsequent anammox process, Bioresour. Technol., 2011, 102(13), 6801–6807, DOI:10.1016/j.biortech.2011.04.011.
- S. Okabe, M. Oshiki, Y. Takahashi and H. Satoh, N2O emission from a partial nitrification-anammox process and identification of a key biological process of N2O emission from anammox granules, Water Res., 2011, 45(19), 6461–6470, DOI:10.1016/j.watres.2011.09.040.
- A. Daverey, Y.-C. Chen, K. Dutta, Y.-T. Huang and J.-G. Lin, Start-up of simultaneous partial nitrification, anammox and denitrification (SNAD) process in sequencing batch biofilm reactor using novel biomass carriers, Bioresour. Technol., 2015, 190, 480–486, DOI:10.1016/j.biortech.2015.02.064.
- H.-B. Chen, Q. Yang, X.-M. Li, Y. Wang, K. Luo and G.-M. Zeng, Post-anoxic denitrification via nitrite driven by PHB in feast-famine sequencing batch reactor, Chemosphere, 2013, 92(10), 1349–1355, DOI:10.1016/j.chemosphere.2013.05.052.
- P. Regmi, M. W. Miller, B. Holgate, R. Bunce, H. Park, K. Chandran, B. Wett, S. Murthy and C. B. Bott, Control of aeration, aerobic SRT and COD input for mainstream nitritation/denitritation, Water Res., 2014, 57, 162–171, DOI:10.1016/j.watres.2014.03.035.
- K. Egli, C. Langer, H.-R. Siegrist, A. J. B. Zehnder, M. Wagner and J. R. van der Meer, Community analysis of ammonia and nitrite oxidizers during start-up of nitritation reactors, Appl. Environ. Microbiol., 2003, 69(6), 3213–3222 CrossRef CAS PubMed.
- W. Jianlong and Y. Ning, Partial nitrification under limited dissolved oxygen conditions, Process Biochem., 2004, 39(10), 1223–1229 CrossRef.
- S. Bagchi, R. Biswas and T. Nandy, Alkalinity and dissolved oxygen as controlling parameters for ammonia removal through partial nitritation and ANAMMOX in a single-stage bioreactor, J. Ind. Microbiol. Biotechnol., 2010, 37(8), 871–876 CrossRef CAS PubMed.
- H. Itokawa, K. Hanaki and T. Matsuo, Nitrous oxide production in high-loading biological nitrogen removal process under low COD/N ratio condition, Water Res., 2001, 35(3), 657–664 CrossRef CAS PubMed.
-
P. Jenicek, P. Svehla, J. Zabranska, R. J. LeBlanc, P. J. Laughton and R. Tyagi, Reject water treatment by nitritation/denitritation process–influence of ammonia concentration and loading rate, Query GMSC, 2007, pp. 683–690 Search PubMed.
-
K. Bilyk, R. Taylor, P. Pitt and D. Wankmuller, Process and economic benefits of sidestream treatment, Proc. Water Environ. Fed. Annu. Conf., 2012, vol. 20122, pp. 889–906 Search PubMed.
- G. T. Daigger, Oxygen and carbon requirements for biological nitrogen removal processes accomplishing nitrification, nitritation, and Anammox, Water Environ. Res., 2014, 86(3), 204–209 CrossRef CAS PubMed.
- K. Rosenwinkel and A. Cornelius, Deammonification in the moving-bed process for the treatment of wastewater with high ammonia content, Chem. Eng. Technol., 2005, 28(1), 49–52 CrossRef CAS.
- B. Wett, Development and implementation of a robust deammonification process, Water Sci. Technol., 2007, 56(7), 81–88 CrossRef CAS PubMed.
- Y. Tao, D.-W. Gao, H.-Y. Wang, M. de Kreuk and N.-Q. Ren, Ecological characteristics of seeding sludge triggering a prompt start-up of anammox, Bioresour. Technol., 2013, 133, 475–481, DOI:10.1016/j.biortech.2013.01.147.
- M. M. Jensen, B. Thamdrup and T. Dalsgaard, Effects of specific inhibitors on anammox and denitrification in marine sediments, Appl. Environ. Microbiol., 2007, 73(10), 3151–3158, DOI:10.1128/AEM.01898-06.
- M. Strous, J. G. Kuenen and M. S. M. Jetten, Key physiology of anaerobic ammonium oxidation, Appl. Environ. Microbiol., 1999, 65(7), 3248–3250 CAS.
- C. Leix, J. E. Drewes and K. Koch, The role of residual quantities of suspended sludge on nitrogen removal efficiency in a deammonifying moving bed biofilm reactor, Bioresour. Technol., 2016, 219, 212–218, DOI:10.1016/j.biortech.2016.07.134.
- B. C. Berks, D. Baratta, D. J. Richardson and S. J. Ferguson, Purification and characterization of a nitrous oxide reductase from Thiosphaera pantotropha. Implications for the mechanism of aerobic nitrous oxide reduction, Eur. J. Biochem., 1993, 212(2), 467–476 CrossRef CAS PubMed.
- H. Lu and K. Chandran, Factors promoting emissions of nitrous oxide and nitric oxide from denitrifying sequencing batch reactors operated with methanol and ethanol as electron donors, Biotechnol. Bioeng., 2010, 106(3), 390–398, DOI:10.1002/bit.22704.
-
J.-C. Bertrand, Environmental microbiology: Fundamentals and applications, Springer, Dordrecht, 2015 Search PubMed.
- D. M. Kool, N. Wrage, S. Zechmeister-Boltenstern, M. Pfeffer, D. Brus, O. Oenema and J. van Groenigen, Nitrifier denitrification can be a source of N2O from soil: a revised approach to the dual-isotope labelling method, Eur. J. Soil Sci., 2010, 61(5), 759–772 CrossRef CAS.
- X. Zhu, M. Burger, T. A. Doane and W. R. Horwath, Ammonia oxidation pathways and nitrifier denitrification are significant sources of N2O and NO under low oxygen availability, Proc. Natl. Acad. Sci. U. S. A., 2013, 110(16), 6328–6333 CrossRef CAS PubMed.
- L. Gong, M. Huo, Q. Yang, J. Li, B. Ma, R. Zhu, S. Wang and Y. Peng, Performance of heterotrophic partial denitrification under feast-famine condition of electron donor: a case study using acetate as external carbon source, Bioresour. Technol., 2013, 133, 263–269, DOI:10.1016/j.biortech.2012.12.108.
- S. Liu and H. Horn, Effects of Fe(II) and Fe(III) on the single-stage deammonification process treating high-strength reject water from sludge dewatering, Bioresour. Technol., 2012, 114, 12–19, DOI:10.1016/j.biortech.2011.11.125.
- A. Rodriguez-Caballero and M. Pijuan, N2O and NO emissions from a partial nitrification sequencing batch reactor: exploring dynamics, sources and minimization mechanisms, Water Res., 2013, 47(9), 3131–3140, DOI:10.1016/j.watres.2013.03.019.
- M. J. Kampschreur, W. R. L. van der Star, H. A. Wielders, J. W. Mulder, M. S. M. Jetten and M. C. M. van Loosdrecht, Dynamics of nitric oxide and nitrous oxide emission during full-scale reject water treatment, Water Res., 2008, 42(3), 812–826, DOI:10.1016/j.watres.2007.08.022.
- R. Ganigué, E. I. P. Volcke, S. Puig, M. D. Balaguer and J. Colprim, Impact of influent characteristics on a partial nitritation SBR treating high nitrogen loaded wastewater, Bioresour. Technol., 2012, 111, 62–69, DOI:10.1016/j.biortech.2012.01.183.
- Z. Hu, T. Lotti, M. van Loosdrecht and B. Kartal, Nitrogen removal with the anaerobic ammonium oxidation process, Biotechnol. Lett., 2013, 35(8), 1145–1154, DOI:10.1007/s10529-013-1196-4.
- T. Abzazou, R. M. Araujo, M. Auset and H. Salvadó, Tracking and quantification of nitrifying bacteria in biofilm and mixed liquor of a partial nitrification MBBR pilot plant using fluorescence in situ hybridization, Sci. Total Environ., 2016, 541, 1115–1123, DOI:10.1016/j.scitotenv.2015.10.007.
- M. Mauret, E. Paul, E. Puech-Costes, M. T. Maurette and P. Baptiste, Application of experimental research methodology to the study of nitrification in mixed culture, Water Sci. Technol., 1996, 34(1), 245–252 CrossRef CAS.
- D.-J. Kim and S.-H. Kim, Effect of nitrite concentration on the distribution and competition of nitrite-oxidizing bacteria in nitratation reactor systems and their kinetic characteristics, Water Res., 2006, 40(5), 887–894 CrossRef CAS PubMed.
- E. N. P. Courtens, N. Boon, H. de Clippeleir, K. Berckmoes, M. Mosquera, D. Seuntjens and S. E. Vlaeminck, Control of nitratation in an oxygen-limited autotrophic nitrification/denitrification rotating biological contactor through disc immersion level variation, Bioresour. Technol., 2014, 155, 182–188 CrossRef CAS PubMed.
- G. Tallec, J. Garnier, G. Billen and M. Gousailles, Nitrous oxide emissions from secondary activated sludge in nitrifying conditions of urban wastewater treatment plants: effect of oxygenation level, Water Res., 2006, 40(15), 2972–2980 CrossRef CAS PubMed.
- J. H. Ahn, S. Kim, H. Park, B. Rahm, K. Pagilla and K. Chandran, N2O emissions from activated sludge processes, 2008−2009: results of a national monitoring survey in the United States, Environ. Sci. Technol., 2010, 44(12), 4505–4511 CrossRef CAS PubMed.
- S. Bourrel, D. Dochain, J. P. Babary and I. Queinnec, Modelling, identification and control of a denitrifying biofilter, J. Process Control, 2000, 10(1), 73–91, DOI:10.1016/S0959-1524(99)00015-3.
- A. Malovanyy, J. Trela and E. Plaza, Mainstream wastewater treatment in integrated fixed film activated sludge (IFAS) reactor by partial nitritation/anammox process, Bioresour. Technol., 2015, 198, 478–487, DOI:10.1016/j.biortech.2015.08.123.
- A. Mano and F. Santana, Nitrite removal in a submerged biofilter, Environ. Technol., 2002, 23(10), 1189–1195 CrossRef CAS PubMed.
- D. Kulikowska and K. Bernat, Nitritation–denitritation in landfill leachate with glycerine as a carbon source, Bioresour. Technol., 2013, 142, 297–303 CrossRef CAS PubMed.
- C. Marina, A. Kunz, M. Bortoli, L. A. Scussiato, A. Coldebella, M. Vanotti and H. M. Soares, Kinetic models for nitrogen inhibition in ANAMMOX and nitrification process on deammonification system at room temperature, Bioresour. Technol., 2016, 202, 33–41 CrossRef PubMed.
- Y. Law, B.-J. Ni, P. Lant and Z. Yuan, N2O production rate of an enriched ammonia-oxidising bacteria culture exponentially correlates to its ammonia oxidation rate, Water Res., 2012, 46(10), 3409–3419, DOI:10.1016/j.watres.2012.03.043.
- P. Wunderlin, M. F. Lehmann, H. Siegrist, B. Tuzson, A. Joss, L. Emmenegger and J. Mohn, Isotope signatures of N2O in a mixed microbial population system: constraints on N₂O producing pathways in wastewater treatment, Environ. Sci. Technol., 2013, 47(3), 1339–1348, DOI:10.1021/es303174x.
Footnote |
† Electronic supplementary information (ESI) available. See DOI: 10.1039/c6ew00216a |
|
This journal is © The Royal Society of Chemistry 2017 |