DOI:
10.1039/C6CE02222G
(Paper)
CrystEngComm, 2017,
19, 142-147
Growing single crystals of small molecules by thermal recrystallization, a viable option even for minute amounts of material?†
Received
21st October 2016
, Accepted 28th November 2016
First published on 28th November 2016
Abstract
The growth of single crystals of small molecules by thermal recrystallization of only a few milligrams of material has been studied for carbamazepine, para-aminobenzoic acid and vitamin B12. High quality single crystals could be grown for carbamazepine and para-aminobenzoic acid within just six hours. Various solvents and concentrations were tested for their influence upon the growth of crystals.
1. Introduction
In contrast to inorganic polymeric materials, for which single crystals are frequently grown by thermal recrystallization,1,2 thermal recrystallization of small molecules is described in most introductions into single crystal growth,3 however the method is rarely used in research laboratories.4–8 There is an exceptional report about the extremely slow cooling of an ethanol solution (0.5 °C per day) to achieve growth of a single crystal of an organic compound.9 Another report described a single crystal grown by cooling a 150 °C hot solution at a rate of −1.25 °C per hour.10 In many cases, it is essential that the employed crystallization methods only use few milligrams of material, as the supply of the compound of interest is limited.11
In this publication, we describe our results from attempts to grow single crystals of 3 different molecular compounds (carbamazepine, para-aminobenzoic acid and vitamin B12) by thermal recrystallization using a small volume of only 0.1 ml with the help of the CrystalBreeder. The single crystals had to have sufficient quality and size in order to allow X-ray analysis on a source diffractometer equipped with a CCD detector and Microfocus Mo and Cu X-ray tubes respectively. This publication is meant to be an extension of our previous tutorial about the growth of single crystals of small molecules, which focused on isothermal methods.11
2. Experimental section
Carbamazepine (CBZ, 97%, P-monoclinic form III,12 see ESI;† we employ the nomenclature of ref. 13) was purchased from ABCR GmbH & Co. KG. Para-Aminobenzoic acid (pABA, ≥99%, α-form, see ESI†14), 1-propanol (≥99%), 2-propanol (≥99.5%), 2-butanol (≥99%), tetrahydrofuran (THF, ≥99.9%), cyclohexane (≥99.5%), acetonitrile (≥99.9%) and ethyl acetate (≥99.9%) were purchased from Sigma Aldrich. All chemicals were used without further purification.
The crystallization experiments were performed with help of the CrystalBreeder (CB), a multi-reactor crystallization platform for medium-throughput solid state research. The CB shows real time turbidity information for 32 parallel vials with a working volume of only 0.1 ml. The vials are arranged in 8 blocks, which can be temperature controlled independently within a range of −15 °C to 145 °C.
UPLC-ESI-MS spectra were recorded on an Acquity Waters system equipped with a PDA detector and an autosampler using an ACQUITY UPLC BEH C18 Gravity 1.7 μm (2.1 mm × 50 mm) reverse phase column. The UPLC system was connected to a Bruker Daltonics HCT ESI-MS spectrometer. A total volume of 1 μL of a sample solution was analysed. A gradient (0 to 0.5 min 5% B, 0.5 to 2 min 30% B, 2 to 4 min 100% B, 4 to 5 min 100% B) of acetontitrile (solvent B) versus Millipore water containing 0.1% formic acid (solvent A) was applied using a flow rate of 0.3 ml min−1. All solvents used were of LCMS grade.
2.1 Thermal crystallization of carbamazepine
The carbamazepine single crystals were grown by thermal crystallization in various solvents and with various concentrations always with 0.1 ml of solvent (Table 1).
Table 1 Properties of solvents tested for the thermal recrystallization of CBZ
Solvent |
Solubility of CBZ in that solvent |
Melting point |
Boiling point |
Mass (in mg) of CBZ for 0.1 ml of a saturated solution |
Estimated from the solubility in 2-propanol.
|
1-Propanol |
18.1 g l−1 (25 °C)15 |
−126 °C |
97 °C |
1.8 |
2-Propanol |
9.11 g l−1 (25 °C)15 |
−88 °C |
82 °C |
0.91 |
2-Butanol |
∼8 g l−1 (25 °C)a |
−115 °C |
99 °C |
0.8 |
THF |
45.2 g l−1 (22.4 °C)16 |
−108 °C |
66 °C |
4.5 |
Cyclohexane |
0.055 g l−1 (25 °C)15 |
6.7 °C |
81 °C |
0.005 |
For every solvent, a minimum of four different concentrations were chosen. Each sample was heated at 1 °C min−1 starting from room temperature until five degrees below the boiling point of the solvent, held at this temperature for 15 minutes, cooled at −0.5 °C min−1 until −15 °C (for cyclohexane only until 0 °C because its melting point is at 7 °C and the solution should not be frozen) and held at the lowest temperature for 15 minutes (Table 2). All samples were stirred with a paddle while heating to ensure that all solids had dissolved. The stirrers were turned off during the cooling.
Table 2 Chosen amounts of CBZ and thermal recrystallization cycle
Solvent |
Vial 1 (mg) |
Vial 2 (mg) |
Vial 3 (mg) |
Vial 4 (mg) |
Heating rate (°C min−1) |
Max temp. (°C) |
Cooling rate (°C min−1) |
Lowest temp. (°C) |
1-Propanol |
1.5 |
2 |
2.5 |
3 |
1 |
92 |
0.5 |
−15 |
2-Propanol |
0.5 |
1 |
1.5 |
2 |
1 |
77 |
0.5 |
−15 |
2-Butanol |
0.5 |
1 |
1.5 |
2 |
1 |
94 |
0.5 |
−15 |
THF low conc. |
4.5 |
5 |
5.5 |
6 |
1 |
60 |
0.5 |
−15 |
THF high conc. |
6 |
6.5 |
7 |
7.5 |
1 |
60 |
0.5 |
−15 |
Cyclohexane |
0.005 |
0.01 |
0.02 |
0.05 |
1 |
76 |
0.5 |
0 |
2.2 Thermal crystallization of p-aminobenzoic acid (pABA)
Crystals of para-aminobenzoic acid were grown by the same method used for CBZ (Tables 3 and 4).
Table 3 Properties of solvents tested for the thermal recrystallization of pABA
Solvent |
Solubility of pABA at 20 °C |
Melting point |
Boiling point |
Mass (in mg) of pABA for 0.1 ml solvent |
2-Propanol |
48 g l−1 (ref. 17) |
−88 °C |
82 °C |
4.8 |
Ethyl acetate |
68 g l−1 (ref. 17) |
−83 °C |
77 °C |
6.8 |
Acetonitrile |
47 g l−1 (ref. 17) |
−45 °C |
82 °C |
4.7 |
Table 4 Chosen amounts of pABA and thermal recrystallization cycle
Solvent |
Vial 1 (mg) |
Vial 2 (mg) |
Vial 3 (mg) |
Vial 4 (mg) |
Max. temp. (°C) |
Heating rate (°C min−1) |
Lowest temp. (°C) |
Cooling rate (°C min−1) |
2-Propanol |
4.5 |
5 |
5.5 |
6 |
77 |
1 |
−15 |
0.5 |
Ethyl acetate |
6.5 |
7 |
7.5 |
8 |
72 |
1 |
−15 |
0.5 |
Acetonitrile |
4.5 |
5 |
5.5 |
6 |
77 |
1 |
−15 |
0.5 |
2.3 Thermal crystallization of vitamin B12
Crystals of vitamin B12 were grown by the same method used for CBZ. The reported solubility of vitamin B12 in water is 10.2 g l−1,18 however we employed higher concentrations of 4 to 8 mg of vitamin B12 in 0.1 ml of water. We obtained crystals by cooling down from 95 °C to −5 °C with cooling rates of either −0.1 or −0.05 °C min−1. Unfortunately, in both cases, the obtained crystal quality was not good enough for an X-ray analysis, since the crystals were clearly not single (see Fig. S1 in the ESI†). Using smaller crystals did not help, as it is our repeated experience with crystals derived from B12 that they need a much bigger size to diffract decently compared with “normal” small molecules.19–26 Furthermore, we have previously made the experience that vitamin B12 and its derivates are rather delicate molecules to crystallize, that only appreciate very few crystallization conditions. Using a concentration of 20 g l−1 (twice the reported concentration of saturation) did not yield any B12 crystals. We checked by UPLC-ESI-MS whether any degradation like hydrolysis of the amide group or removal of the cyanide of the B12 was observed during the extended thermal treatment in an aqueous solution. As can be seen in Fig. S2 in the ESI,† no deterioration of the B12 could be observed, when compared with B12 that did not undergo any thermal treatment, based upon peak purity, retention time and isotopic distribution of the mass spectra.
2.4 X-ray measurement
Crystallographic data were collected at 183(2) K on an Agilent SuperNova, dual source, single X-ray diffractometer equipped with an Atlas CCD detector with either Mo Kα radiation (λ = 0.7107 Å) for the monoclinic form of CBZ and pABA or with Cu Kα radiation (λ = 1.54184 Å) for the rhombohedral form of CBZ. Suitable crystals were covered with oil (Infineum V8512, formerly known as Paratone N), placed on a nylon loop that is mounted on a CrystalCap Magnetic™ (Hampton Research) and immediately transferred to the diffractometer. Data were corrected for Lorentz and polarisation effects as well as for absorption. The program suite CrysAlisPro was used for data collection, multi-scan absorption correction (additionally a numerical absorption correction was done for pABA) and data reduction.27 Structures were solved with direct methods using SIR97 (ref. 28) and were refined by full-matrix least-squares methods on F2 with SHELXL-2014.29 CCDC-1494536–1494538 contain the supplementary crystallographic data for this paper.
3. Results and discussion
For every solvent at least four different concentrations were chosen. The lowest concentration was at about 75% of the solubility at room temperature and the other three concentrations were from 100 to 150/200% of the RT solubility. Each sample was heated until five degrees below the boiling point of the solvent. Despite the small starting volume, no solvent loss from tube could be observed. However, in case of viscous solvents (like water), up to 18% of the solvent ended up in separated drops above the original solution between the outer wall and the paddle.
3.1 Carbamazepine crystals
Carbamazepine is known to form five polymorphs and many solvates.13,30 While there are many publications about the influence of cooling rates, concentrations, solvents, seeds and stirring rates upon the (co)formation of one of these polymorphs,5,13,15,16,30–36 the focus of this work is exclusively on the formation of single crystals suitable for X-ray analysis. We have tested five different solvents at 4–8 concentrations for the growth of carbemazepine single crystals upon cooling back to the originally (super)saturated solutions at room temperature. No crystals or even any precipitate could be obtained from 1-propanol. In the case of 2-propanol, colourless, thin needles and prismatic single crystals appeared on the bottom of the vials with 1.5 mg and 2 mg of material (Fig. 1), respectively. Only one small crystal grew on the pedal stirrer in the vial with 1 mg of CBZ. By single-crystal analysis, we could show that the needles are the CBZP-monoclinic form III and that the thick, prismatic, 3-dimensional crystals are CBZ with one sixth occupied water molecule as a solvate crystallizing in the space group R
(Fig. 3 left and Table 5). It must be noted that in several publications, an identical rhombohedral cell has been reported, however once the single crystal data were refined without any solvate molecules and named trigonal form II.37 In two other publications with a very similar cell, one partially occupied THF38 or a partially occupied water35 were found in the structure. As in 2-propanol, needle-shaped crystals of CBZ grew from 2-butanol on the bottom of the vial and on the stirrer. However, no prismatic single crystals appeared. From THF, CBZ crystallized not only as needles but also as prismatic single crystals (Fig. 2). At a concentration of 50 mg ml−1, needle-like crystals appeared on the bottom of the vial and on the stirrer (Fig. 2 left). Increasing the concentration to 55 mg ml−1 yielded crystalline needles in the vial and one small prismatic crystal on the edge of the vial (Fig. 2 middle). Finally, just one large single crystal can be seen in the vial with a concentration of 60 mg ml−1 (Fig. 2 right). Only a few small, crystalline needles grew on the stirrer from cyclohexane, as was expected due to the low solubility of CBZ in cyclohexane.
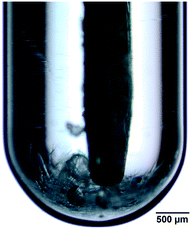 |
| Fig. 1 Crystals of CBZ grown from 2-propanol in the shape of needles and prisms. The picture shows the vial together with the paddle. | |
Table 5 Crystallographic data of the measured single crystals of CBZ and pABA
Compound |
CBZ
|
CBZ·1/6(H
2
O)
|
pABA
|
Used volume: 0.1 ml.
See text in chapter 3.1.
|
Grown froma |
2-Propanol at a concentration of 15 g l−1 |
2-Propanol at a concentration of 15 g l−1 |
Ethyl acetate at a concentration of 80 g l−1 |
Space group |
P21/n |
R![[3 with combining macron]](https://www.rsc.org/images/entities/char_0033_0304.gif) |
P21/n |
Form |
P-Monoclinic form III12 |
|
α-form39 |
a [Å] |
7.49441(10) |
35.2832(9) |
18.5413(3) |
b [Å] |
11.06430(14) |
35.2832(9) |
3.77083(7) |
c [Å] |
13.80360(15) |
5.20165(13) |
18.5858(3) |
α [°] |
90 |
90 |
90 |
β [°] |
92.9142(11) |
90 |
93.6727(17) |
γ [°] |
90 |
120 |
90 |
Volume [Å3] |
1143.12(2) |
5608.0(3) |
1296.78(4) |
Crystal size [mm3] |
0.37 × 0.33 × 0.17 |
0.17 × 0.05 × 0.05 |
0.34 × 0.09 × 0.07 |
X-ray wavelength [Å] |
0.71073 |
1.54184 |
0.71073 |
Independent reflections |
5891 [R(int) = 0.0244] |
2482 [R(int) = 0.0086] |
4512 [R(int) = 0.0201] |
Reflections observed [I > 2σ(I)] |
4768 |
2277 |
3791 |
Completeness to resolution |
99.9% to 0.60 Å |
99.9% to 0.84 Å |
99.9% to 0.70 Å |
Final R indices [I > 2σ(I)] |
R
1 = 0.0426, wR2 = 0.1200 |
R
1 = 0.0474, wR2 = 0.1566 |
R
1 = 0.0505, wR2 = 0.1313 |
Largest diff. peak and hole [e Å−3] |
0.459 and −0.219 |
0.635 and −0.233 |
0.368 and −0.190 |
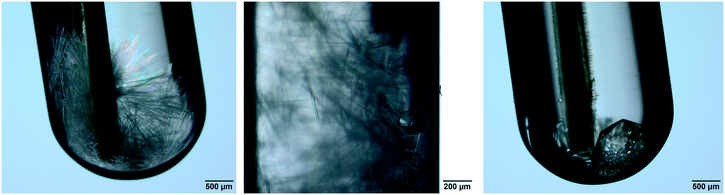 |
| Fig. 2 Crystals of CBZ grown from THF at a concentration of 50 mg ml−1 (left), of 55 mg ml−1 (middle) and of 60 mg ml−1 (right). | |
Matzger and co-workers obtained the P-monoclinic form III by slow evaporation from ethanol and the metastable trigonal form II by cooling a hot ethanol solution.13 Both forms were characterized by X-ray powder diffraction. Sefcik observed a temporary formation of form II in ethanol followed by appearance of form III.34 They noted the influence of stirring and of an unknown impurity upon the crystallization pathway.
3.2 p-Aminobenzoic acid crystals
pABA did not form any crystals in 2-propanol for all concentrations used in the experiments. In ethyl acetate, plate-like crystals of pABA formed (Fig. 4). In acetonitrile, the same result was obtained as in ethyl acetate: some needle crystals were formed on the bottom of the vial. Single crystals of excellent quality were obtained from ethyl acetate at concentration of 80 mg ml−1 (Fig. 3, right, Table 5). The exceptional quality of obtained dataset is indicated by the final difference electron density maps, where the largest peaks of residual density lie between bonded atoms and represent the bonding electrons, which are not accounted for by classical spherical atom refinement models. Sullivan obtained single crystals of the α-form by isothermal evaporation of saturated solutions of 2-propanol, ethanol, methanol, ethyl acetate, acetonitrile, nitromethane and 1
:
1 methanol
:
toluene at room temperature and the β-form by isothermal evaporation of saturated solutions of water.39 They had to wait up to 2 weeks for the completion of the experiments. Extensive concentration of the saturated solution at room temperature was obviously essential for crystal growth at least in the case of 2-propanol, as we could not form any crystals from 2-propanol with our thermal recrystallization method.
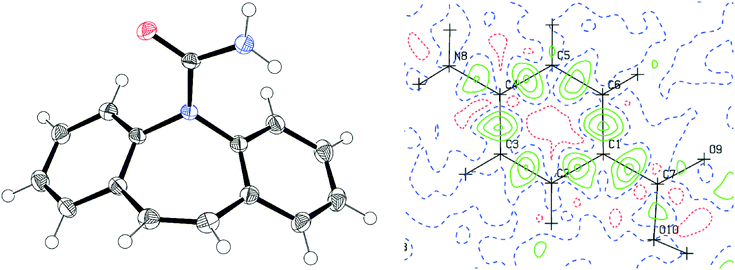 |
| Fig. 3 Left: ORTEP representation of the P-monoclinic form of CBZ at 50% probability; right: residual electron density map for the pABA structure. | |
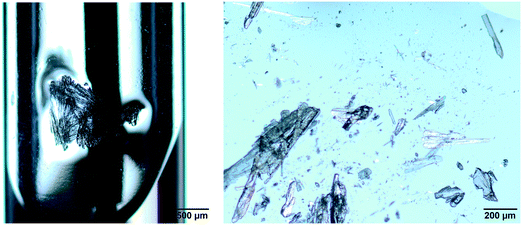 |
| Fig. 4 Left: plate-like crystals of pABA grown from ethyl acetate in the vial at a concentration of 80 mg ml−1; right: isolated crystals of pABA grown from ethyl acetate at a concentration of 80 mg ml−1. | |
4. Conclusion
This study was done in order to evaluate the growth of single crystals of small molecules from minute volumes of only 0.1 ml by thermal recrystallization. For this method, it is obviously necessary that the desired molecule must change its solubility with temperature in a steady way and by a significant amount,40 additionally the molecule must be stable over the chosen temperature range. Since some molecules only slightly change their solubility as a function of temperature, it is extremely helpful to know exactly the solubility in the chosen solvents at the desired temperature (normally around 25 °C), either from the literature or from own experimentation. In order to further minimize the needed amount of material, one could start with one single initial experiment per solvent at an concentration of 150% of the saturated solution. Within only six hours using the CrystalBreeder, we were able to grow excellent quality single crystals of carbamazepine and p-aminobenzoic acid from as little as 1.5 mg of material. However, it is clear that this method is not suitable for all molecules, as shown in the case of the vitamin B12. Additionally, by comparing our results of pABA with the work of Sullivan,39 it is clear that cooling a slightly supersaturated solution will not yield in all cases crystals, unless evaporation further increases the supersaturation.
Acknowledgements
We thank the University of Zurich for financial support. We thank Technobis for providing us with a CrystalBreeder, Michael Olah for measuring the powder X-ray spectra, Lucas Prieto for recording the UPLC-ESI-MS chromatograms and Prof. Dr. Tony Linden for a careful reading of our manuscript.
References
-
D. Elwell and H. J. Scheel, Crystal Growth from High-Temperature Solutions, Academic, 2nd edn, 2011 Search PubMed.
-
V. Glebovsky, in Recrystallization, ed. K. Sztwiertnia, InTech, 2012, pp. 59–86 Search PubMed.
-
P. D. Boyle, Growing Crystals That Will Make Your Crystallographer Happy, http://xray.chem.uwo.ca/crystal_growing/GrowXtal.html, (accessed 8th April 2016) Search PubMed.
- D. J. Watkin, J. Appl. Crystallogr., 1972, 5, 250 CrossRef CAS.
- A. J. Florence, A. Johnston, S. L. Price, H. Nowell, A. R. Kennedy and N. Shankland, J. Pharm. Sci., 2006, 95, 1918–1930 CrossRef CAS PubMed.
- D. M. Homden, C. Redshaw and D. L. Hughes, Inorg. Chem., 2007, 46, 10827–10839 CrossRef CAS PubMed.
- I. Mendoza, N. Curado, M. Carrasco, E. Alvarez, R. Peloso, A. Rodriguez and E. Carmona, Inorg. Chim. Acta, 2015, 424, 120–128 CrossRef CAS.
- D.-R. Dauer, M. Flügge, R. Herbst-Irmer and D. Stalke, Dalton Trans., 2016, 45, 6149–6158 RSC.
- T. Notake, K. Nawata, H. Kawamata, T. Matsukawa and H. Minamide, Opt. Mater. Express, 2012, 2, 119–125 CrossRef CAS.
- R. W. Seidel, J. Graf, R. Goddard and I. M. Oppel, Acta Crystallogr., Sect. E: Struct. Rep. Online, 2011, 67, m236–m237 CAS.
- B. Spingler, S. Schnidrig, T. Todorova and F. Wild, CrystEngComm, 2012, 14, 751–757 RSC.
- V. L. Himes, A. D. Mighell and W. H. De Camp, Acta Crystallogr., Sect. B: Struct. Crystallogr. Cryst. Chem., 1981, 37, 2242–2245 CrossRef.
- A. L. Grzesiak, M. Lang, K. Kim and A. J. Matzger, J. Pharm. Sci., 2003, 92, 2260–2271 CrossRef CAS PubMed.
- T. F. Lai and R. E. Marsh, Acta Crystallogr., 1967, 22, 885–893 CrossRef CAS.
- R. C. Kelly and N. Rodriguez-Hornedo, Org. Process Res. Dev., 2009, 13, 1291–1300 CrossRef CAS.
- W. Liu, L. Dang, S. Black and H. Wei, J. Chem. Eng. Data, 2008, 53, 2204–2206 CrossRef CAS.
- Gestis-Stoffdatenbank, gestis.itrust.de, (accessed 22.2.2016).
- X. Wang, L. Wei and L. P. Kotra, Bioorg. Med. Chem., 2007, 15, 1780–1787 CrossRef CAS PubMed.
- S. Kunze, F. Zobi, P. Kurz, B. Spingler and R. Alberto, Angew. Chem., Int. Ed., 2004, 43, 5025–5029 CrossRef CAS PubMed.
- S. Mundwiler, B. Spingler, P. Kurz, S. Kunze and R. Alberto, Chem. – Eur. J., 2005, 11, 4089–4095 CrossRef CAS PubMed.
- B. Spingler, S. Mundwiler, P. Ruiz-Sanchez, D. R. van Staveren and R. Alberto, Eur. J. Inorg. Chem., 2007, 2641–2647 CrossRef CAS.
- P. Ruiz-Sanchez, S. Mundwiler, A. Medina-Molner, B. Spingler and R. Alberto, J. Organomet. Chem., 2007, 692, 1358–1362 CrossRef CAS.
- P. Ruiz-Sanchez, S. Mundwiler, B. Spingler, N. R. Buan, J. C. Escalante-Semerena and R. Alberto, J. Biol. Inorg. Chem., 2008, 13, 335–347 CrossRef CAS PubMed.
- K. Zelenka, H. Brandl, B. Spingler and F. Zelder, Dalton Trans., 2011, 40, 9665–9667 RSC.
- R. M. Oetterli, L. Prieto, B. Spingler and F. Zelder, Org. Lett., 2013, 15, 4630–4633 CrossRef CAS PubMed.
- L. Prieto, M. Neuburger, B. Spingler and F. Zelder, Org. Lett., 2016, 18, 5292–5295 CrossRef CAS PubMed.
- Rigaku Oxford Diffraction, CrysAlisPro Software system, vers. 171.38, 2015 Search PubMed.
- A. Altomare, M. C. Burla, M. Camalli, G. L. Cascarano, C. Giacovazzo, A. Guagliardi, A. G. G. Moliterni, G. Polidori and R. Spagna, J. Appl. Crystallogr., 1999, 32, 115–119 CrossRef CAS.
- G. M. Sheldrick, Acta Crystallogr., Sect. C: Struct. Chem., 2015, 71, 3–8 CrossRef PubMed.
- J.-B. Arlin, L. S. Price, S. L. Price and A. J. Florence, Chem. Commun., 2011, 47, 7074–7076 RSC.
- M. Lang, A. L. Grzesiak and A. J. Matzger, J. Am. Chem. Soc., 2002, 124, 14834–14835 CrossRef CAS PubMed.
- M. Lang, J. W. Kampf and A. J. Matzger, J. Pharm. Sci., 2002, 91, 1186–1190 CrossRef CAS PubMed.
- R. Hilfiker, J. Berghausen, F. Blatter, A. Burkhard, S. M. De Paul, B. Freiermuth, A. Geoffroy, U. Hofmeier, C. Marcolli, B. Siebenhaar, M. Szelagiewicz, A. Vit and M. von Raumer, J. Therm. Anal. Calorim., 2003, 73, 429–440 CrossRef CAS.
- K. Sypek, I. S. Burns, A. J. Florence and J. Sefcik, Cryst. Growth Des., 2012, 12, 4821–4828 CAS.
- R. Prohens, M. Font-Bardia and R. Barbas, CrystEngComm, 2013, 15, 845–847 RSC.
- J. V. Parambil, S. K. Poornachary, S. J. Hinder, R. B. H. Tan and J. Y. Y. Heng, CrystEngComm, 2015, 17, 6384–6392 RSC.
- M. M. J. Lowes, M. R. Caira, A. P. Lötter and J. G. Van der Watt, J. Pharm. Sci., 1987, 76, 744–752 CrossRef CAS PubMed.
- F. P. A. Fabbiani, L. T. Byrne, J. J. McKinnon and M. A. Spackman, CrystEngComm, 2007, 9, 728–731 RSC.
- R. A. Sullivan and R. J. Davey, CrystEngComm, 2015, 17, 1015–1023 RSC.
-
B. Averill and P. Eldredge, Chemistry: Principles, Patterns and Applications, Pearson, 2005 Search PubMed.
Footnote |
† Electronic supplementary information (ESI) available: X-ray powder diffraction of commercial CBZ and pABA. Diffraction image obtained during the initial unit cell determination of a twinned vitamin B12 crystal. UPLC-ESI-MS chromatograms of vitamin B12 before and after the thermal treatment. CCDC 1494536–1494538. For ESI and crystallographic data in CIF or other electronic format see DOI: 10.1039/c6ce02222g |
|
This journal is © The Royal Society of Chemistry 2017 |