DOI:
10.1039/C7AY90097J
(AMC Technical Brief)
Anal. Methods, 2017,
9, 4338-4340
Surface-enhanced Raman spectroscopy (SERS) in cultural heritage
Received
5th July 2017
First published on 26th July 2017
Abstract
Surface-enhanced Raman spectroscopy (SERS) has been increasingly used in the last decade for the identification of organic colourants in works of art. ‘Surface-enhancement’ is the giant increase in Raman scattering intensity experienced by organic molecules adsorbed on atomically rough metallic surfaces. In the case of organic colourants, which are in general strongly luminescent under laser excitation and therefore cannot usually be identified by conventional Raman microscopy (see AMCTB no. 67), SERS yields a strong and clearly resolved spectrum, allowing for easy identification. Unlike ordinary Raman spectroscopy, SERS often requires sampling, yet the sample size is considerably smaller (from 20 to 100 micrometres) than that required for high-performance liquid chromatography (HPLC), the benchmark technique for organic colourants analysis. For this reason, SERS has seen substantial use in the analysis of works of art such as prints, drawings, paintings, and polychrome sculpture. This technical brief focuses on the practical aspects of SERS and its application to the analysis of cultural heritage material, as well as on its current limitations.
Introduction
Surface-enhanced Raman spectroscopy (SERS) is a relatively new technique, first applied to the study of cultural heritage materials in 1987, when Guineau and Guichard identified alizarin in an 8th century textile dyed with madder.1 It took, however, almost twenty years (and the widespread adoption of Raman spectroscopy in museums) for other researchers to follow. In the last decade a substantial amount of work on SERS-based identification of organic colourants in microscopic samples of dyed fibres, paints, and glazes has been carried out. New substrates and separation techniques have been developed, extraction methods have been improved, minimally invasive methods have been proposed, and database libraries of spectra have been assembled.
The enormous enhancement (as high as 1012) of Raman scattering intensity experienced by molecules adsorbed on atomically rough metallic surfaces (usually made of nanoparticles of the so-called coinage metals, i.e., silver, copper or gold) results in increased sensitivity for the identification of minor and trace components. At the same time, adsorption on metallic surfaces generally quenches fluorescence, thus enabling SERS to address the two great shortcomings of conventional Raman spectroscopy: low sensitivity and interference from luminescence. These characteristics make SERS uniquely suited to the analysis of organic colourants, both natural and synthetic (for a detailed review of SERS applications in cultural heritage studies, see ref. 2).
Of the metals used, silver is by far the most popular, giving nanoparticles that show high affinity for organic dyes, and resulting in excellent analytical sensitivity. Silver nanoparticles can be synthesized to show surface-enhancement over the blue to near infrared range of laser excitation wavelengths (488–785 nm), which is what most Raman instruments in cultural heritage institutions employ.
Silver nanoparticles, in the form of silver colloids, can be prepared easily in the laboratory with safe chemical procedures.3 SERS measurements are normally carried out with a standard Raman microspectrometer, on a microscopic sample immersed in a drop of silver colloid or decorated with a colloidal paste (a thicker slurry made by concentrating a silver colloid by centrifugation) as shown in Fig. 1.
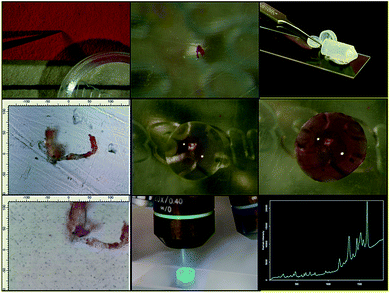 |
| Fig. 1 SERS analysis in practice: from top left, going left to right and top to bottom: sampling a coloured paper fibre with a tungsten needle; the sample; sample treatment with hydrofluoric acid (HF) to break the metal-dye molecule bond in the lake pigment; the same after treatment (scale in micrometres); silver colloid added to the sample; the colloid begins to aggregate following adsorption of the dye onto the nanoparticles; the Raman microscope is focused into the colloid drop (dark dots are large nanoparticles aggregates); the 488 nm laser beam is focused into the drop; the SERS spectrum of eosin. | |
Procedure
SERS is classified as a micro-destructive technique: it can be performed on a micro-sample taken from the object (usually a fraction of a millimetre across), or in situ, i.e., on the object itself. In the latter case, a drop of the metal colloid (usually 1 mm across or less) is deposited on the surface to be analysed. When performing SERS on a sample, some authors have found it advantageous to treat the sample with an acid to hydrolyse lake pigments or generally break the bond between dye and its substrate. This procedure allows the dye molecules more easily to diffuse into the colloid drop and adsorb on the surface of the nanoparticles. In a recent development of the technique, a gelatine nanocomposite is prepared and put on the spot to analyse; after the analysis, the gelatine can be peeled off and removed without leaving either a mark visible to the naked eye, or traces of the metal nanoparticles. However, the gelatine is slightly wet and this may be an issue with water-soluble pigments and dyes or on water-sensitive substrates.
What is SERS good for?
SERS has been used extensively in museum laboratories for the analysis of a variety of cultural heritage objects spanning a large geographical and chronological range and covering all types of materials, including textiles, prints, drawings, paintings, and polychrome sculpture.
The bulk of the published examples concerns red anthraquinone dyes such as carmine and madder. For example, SERS was used to document the oldest known so far example of a lake pigment made from madder, in an Egyptian leather fragment dating to the Middle Kingdom (Fig. 2), as well as the first occurrence of lac dye in western European art, in a Romanesque sculpture from Southern France.3
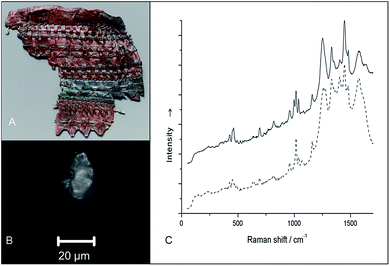 |
| Fig. 2 (A) Fragment of a quiver; accession no. 28.3.5; Middle Kingdom, ca. 2124–1981 BC (H 11 cm; W 13 cm). MMA 1911–1912, Tomb MMA830, Thebes, el-Khokha, Upper Egypt; Rogers Fund, 1928. (B) Polarized reflected light photograph of sample removed from red painted area before HF treatment (scale bar: 20 μm). (C) SERS spectrum of sample from Middle Kingdom leather quiver. Solid line, spectrum of sample from red painted area; dashed line: spectrum of a madder lake reference. The spectra were transposed vertically for clarity. | |
SERS is equally suitable to the analysis of textile fibres, watercolor and tempera paints, and lake pigments in oil (for an example of the latter, see ref. 4).
SERS has been applied, in addition to anthraquinones such as madder, cochineal, kermes, and lac dye, to all major natural colourants used as textile dyes and lake pigments, and to a good number of synthetic ones (see for instance ref. 5–7). A summary list would include weld, fustic, berberine, logwood, safflower, brazilwood, orchil, shikon, methyl violet, magenta, mauveine, and eosin.
Advantages and limitations
The chief advantage of SERS is its extremely high sensitivity, which makes it possible to identify target molecules from fibres or paint samples as small as 20 micrometres. This is particularly important given the relatively poor performance of non-invasive methods with the majority of organic dyes. Before the introduction of SERS in the cultural heritage field, definitive identification of an organic dye was a task generally accomplished using high-performance liquid chromatography (HPLC). While a few examples of the application of HPLC to paintings exist, the majority of HPLC work concerns textiles such as carpets and tapestries, from which a few millimetres of textile fibres can be obtained. SERS has enabled researchers in museums to work as routine on paintings (Fig. 3) and other objects from which sampling is more limited.
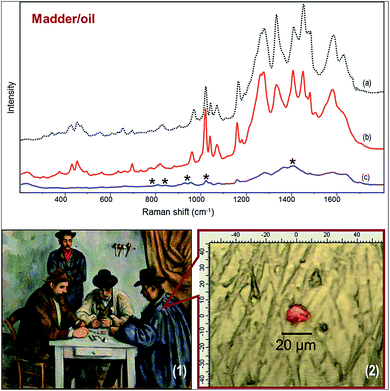 |
| Fig. 3 SERS spectra from ‘The Card Players’, Paul Cézanne, MMA 61.101.1. (a) Reference madder lake upon HF treatment, (b) sample on Ag colloid upon HF treatment; (c) sample on 5× Ag colloid without hydrolysis (peaks marked with * are due to the colloid itself). (1) The painting. (2) The sample under the stereomicroscope. | |
The key limitation of SERS is particularly evident when the technique is compared to HPLC. Unlike chromatography, where individual components of a mixture are separated and then analysed with a spectroscopic method, the spectral response in SERS is the aggregate response of all components. To complicate the picture, some dyes show a greater affinity for adsorption on silver than even closely related dyes, making it difficult to resolve dye mixtures.8
Analysis of spectra
The analysis of SERS spectra is accomplished by comparison with reference spectra. Spectral databases have been assembled by various groups.5,6 Automated or semi-automated digital spectral library search methods have been evaluated, demonstrating their reliability.9 The limitation highlighted in the previous paragraph concerning analysis of mixtures should, however, be kept in mind. Finally, assignment of spectral peaks to specific vibrations is complicated by surface-enhancement and its specific selection rules. This task generally requires computational ab initio methods to simulate the Raman spectrum.
Marco Leona (The Metropolitan Museum of Art, New York).
This Technical Brief was prepared by the Heritage Science Subcommittee and approved by the Analytical Methods Committee on 29/06/17.
References
-
B. Guineau and V. Guichard, ICOM Committee for Conservation: 8th Triennial Meeting, Preprints, The Getty Conservation Institute, Marina del Rey, CA, 1987, Vol. 2, p. 659 Search PubMed
.
- F. Pozzi and M. Leona, Surface-enhanced Raman spectroscopy in art and archaeology, J. Raman Spectrosc., 2015, 47, 67–77 CrossRef
.
- M. Leona, Proc. Natl. Acad. Sci. U. S. A., 2009, 106, 14757 CrossRef CAS PubMed
.
- F. Pozzi, K. J. van den Berg, I. Fiedler and F. Casadio, A systematic analysis of red lake pigments in French impressionist and post-impressionist paintings by surface-enhanced Raman spectroscopy (SERS), J. Raman Spectrosc., 2014, 45, 1119–1126 CrossRef CAS
.
- M. Leona, J. Stenger and E. Ferloni, Application of surface-enhanced Raman scattering techniques to the ultra-sensitive analysis of natural dyes in works of art, J. Raman Spectrosc., 2006, 37, 981–992 CrossRef CAS
.
- S. Bruni, V. Guglielmi and F. Pozzi, Historical organic dyes: a surface-enhanced Raman scattering (SERS) spectral database on Ag Lee–Meisel colloids aggregated by NaClO4, J. Raman Spectrosc., 2011, 42, 1267–1281, DOI:10.1002/jrs.2872
.
- I. Geiman, M. Leona and J. R. Lombardi, Application of Raman spectroscopy and SERS to the analysis of synthetic dyes found in ballpoint inks, J. Forensic Sci., 2009, 54, 947–952 CrossRef CAS PubMed
.
- F. Pozzi, S. Zaleski, F. Casadio and R. P. Van Duyne, SERS discrimination of closely related molecules: a systematic study of natural red dyes in binary mixtures, J. Phys. Chem. C, 2016, 120, 21017–21026 CAS
.
- F. Pozzi, S. Porcinai, J. R. Lombardi and M. Leona, Statistical methods and library search approaches for fast and reliable identification of dyes using surface-enhanced Raman spectroscopy (SERS), Anal. Methods, 2013, 5, 4205–4212 RSC
.
|
This journal is © The Royal Society of Chemistry 2017 |