DOI:
10.1039/C7AY01927K
(Paper)
Anal. Methods, 2018,
10, 69-75
Carrier-mediated solvent bar microextraction coupled with HPLC-DAD for the quantitative analysis of the hydrophilic antihypertensive peptide VLPVPR in human plasma
Received
8th August 2017
, Accepted 21st November 2017
First published on 22nd November 2017
Abstract
The aim of this study was to develop a method for the determination of the Val-Leu-Pro-Val-Pro-Arg (VLPVPR) antihypertensive peptide in human plasma based on a carrier-mediated three-phase solvent bar microextraction (SBME) coupled with a high performance liquid chromatography diode array detector (HPLC-DAD). For this purpose, the SBME was performed under various operating conditions to optimize the parameters including the pH of the donor and receiving phases, sodium chloride (NaCl) concentration in the receiving phase, carrier concentration, agitation rate, and extraction time. A high enrichment effect was achieved when the pH of the donor phase (i.e., sample solution) was 12, the pH of the receiving phase was 2, the NaCl concentration in the receiving phase was 2 mol L−1, the carrier concentration was 15% (w/v), the agitation rate was 700 rpm, and the extraction time was 60 min. Furthermore, the analytical characteristics of the SBME-HPLC method including the linear range (0.2–20 μg mL−1), the detection limit (68.5 ng mL−1), the precision (RSD = 1.1%, n = 5), and the enrichment factor (27.5 ± 0.65) were evaluated in human plasma. The results showed that the method was suitable for the determination of VLPVPR antihypertensive peptide in bio-fluid samples.
1. Introduction
Hypertension is a serious global health concern as it is associated with the development of cardiovascular diseases.1 The key point for the treatment of hypertension is the blockage of the enzyme angiotensin converting enzyme (ACE) involved in the renin-angiotensin system.2–4 Liu et al. demonstrated a strong ACE inhibition effect of the hydrophilic antihypertensive peptide Val-Leu-Pro-Val-Pro-Arg (VLPVPR) in in vitro experiments.5,6 However, to obtain a more quantitative understanding of the pharmacokinetics of this peptide, challenges remain in the sample preparation (extraction and concentration) of VLPVPR from blood samples for accurate quantification due to its low concentrations in test blood and significant interferences from large amount of impurities.7–10 Furthermore, the VLPVPR peptide is labile in biological samples due to its fast metabolism; this results in additional difficulties in quantitative analysis. Therefore, it is desirable to develop a reliable, sensitive, and simple analytical method to monitor the concentration of the VLPVPR peptide in biological samples. In the past few decades, HPLC has been the most widely used analytical instrument for the analyses of water-soluble peptides. However, the HPLC method suffers from low sensitivity and selectivity, especially when the analyte peptide is at very low concentrations and coexists in test samples with endogenous analogue substances. Therefore, selective concentration of the VLPVPR peptide during sample preparation would be critical for accurate HPLC analysis.
Hollow fiber-liquid phase microextraction (HF-LPME) has been demonstrated effective in sample preparation for HPLC analysis, where hollow fibers are used to host the organic solvent (the extraction phase) for the extraction of the analyte and its enrichment.11–14 Compared to the traditional organic solvent extraction, LPME uses only microliter amounts of organic solvent, thus being deemed a green chemistry approach due to its reduced environmental harmfulness. In previous biomedical studies, hollow fibers with a specific molecular weight cut-off have been widely employed in dialysis to prevent biomacromolecules from entering the dialysate.15 Similarly, HF-LPME has been proven effective in biological sample preparation.11–14,16–18 However, conventional HF-LPME involves the extraction of a hydrophobic analyte from the aqueous sample solution (the donor phase) to the organic solvent-based extraction phase using passive diffusion,17,18 which is not effective to concentrate water-soluble analytes such as VLPVPR peptide. The VLPVPR peptide is amphoteric and therefore is easy to be deprotonated and form anions in an alkaline solution. Under these conditions, in a three-phase system (Fig. 1) [i.e., aqueous sample phase (SP)⋯HF-LPME extraction phase (i.e., interphase)⋯aqueous receiving phase (RP)], the addition of anion carrier molecules to the interphase may facilitate the active transport of VLPVPR anions from the SP to the aqueous RP.19–21
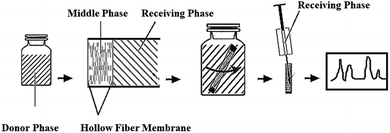 |
| Fig. 1 Schematic for the preparation of a solvent bar and its applications for extraction and analysis of the VLPVPR antihypertensive peptide. | |
Hence, we developed an effective approach by coupling novel HF-LPME with HPLC for sensitive determination of water soluble VLPVPR peptide in human plasma. Herein, a solvent bar (SB) hosted in a hollow fiber was loaded with aliquat-336 (the carrier of anionic peptides, i.e., [R3NCH3]+), which actively transported VLPVPR anions from SP to cross the hollow fiber and reach the inner RP, offering a high enrichment factor and improved sensitivity. As demonstrated in real plasma sample applications, the developed method is highly effective and efficient in the hydrophilic peptide extraction in complex biological samples.
2. Experimental
2.1 Chemicals and reagents
Methanol, trifluoroacetic acid (TFA), octanol (HPLC grade), and methyl trioctyl ammonium chloride (aliquat 336, ≥97%) were purchased from Sigma (St. Louis, MO, USA). Phosphoric acid, monopotassium phosphate, sodium chloride (NaCl), sodium hydroxide (NaOH), and hydrochloric acid (HCl) were bought from Sinopharm Chemical Reagent Co., Ltd (Shanghai, China). The human plasma samples were obtained from healthy volunteers (Shenzhen, China) and stored in a refrigerator at −20 °C for use. Deionized water was freshly obtained from a Milli-Q system (Millipore, Bedford, MA, USA). The VLPVPR antihypertensive peptide standard was synthesized by Yao biotechnology (Shanghai, China). The 10 mL sample vials were purchased from Agilent (Santa Clara, CA, USA). The polyvinylidene fluoride (PVDF) hollow fiber membrane (with a wall thickness of 200 μm, aninner diameter of 1.2 mm, a pore diameter of 0.2 μm, and a porosity of 85%) was obtained from the Tianjin University of Science and Technology (Tianjin, China).
2.2 Instrument
High performance liquid chromatography (HPLC, LC-20AT, Shimadzu, Japan) was used for analysis of the VLPVPR antihypertensive peptide using an Agilent Zorbax Eclipse XDB C18 (250 mm × 4.6 mm, 5 μm) column and a photodiode array detector.
2.3 Preparations of the solutions and pretreatment of human plasma
A stock solution (1 mg mL−1) containing the VLPVPR peptide was prepared with 10 mL of deionized water and stored at −20 °C until use. The pH values of optimization SP and RP were adjusted using the NaOH solution and HCl solution, respectively. The sample solutions were mainly prepared in phosphate buffer solutions, in which 1.36 g of monopotassium phosphate and 79 mL NaOH solution (0.1 mol L−1) were dissolved in deionized water to a final volume of 200 mL.
Since the VLPVPR peptide is degraded quickly by enzymes in human plasma, the inactivation of plasma enzymes is critical. This is carried out as follows: alcohol precipitation is performed by mixing 1.2 mL human plasma with 3.6 mL methanol. The mixtures were then centrifuged for 5 min at 12
000 rpm to obtain the supernatant liquor. Finally, the pH value of the supernatant liquor is adjusted to 12 by NaOH solutions for solvent bar extraction.
2.4 Preparation of the solvent bar and extraction and HPLC-DAD analysis procedures
The preparation of the solvent bars was similar to that reported in our previous study.22 A schematic presenting the steps of the analytical procedures (i.e., the preparation of SB and the extraction–detection procedure) used in the study is shown in Fig. 1.
Typically, the PVDF hollow fiber was cut into 30 mm portions and cleaned by methanol using an ultraphonic cleaner to remove impurities completely. The cleaned PVDF hollow fiber was air-dried for the following experiments. Then, the PVDF hollow fiber was immersed in octanol-containing aliquat 336 for 5 min to load the peptide anion carrier into the surface pores of the fiber. Subsequently, one end of the PVDF hollow fiber was heat-sealed to accommodate 25 μL RP, which was added to the PVDF hollow fiber slowly using a microsyringe. Finally, the other end of the PVDF hollow fiber was heat-sealed to obtain a solvent bar for the convenience of immersion in the sample. The prepared solvent bar was stored and used in the following extraction procedure.
The prepared solvent bar was transferred into a sample vial containing 8 mL of the donor phase to extract the VLPVPR antihypertensive peptide. The sample vial was then placed in a turbine oscillator to be agitated for a certain time to speed up the extraction procedure. After agitation, the solvent bar was extracted from the sample vial, and 20 μL of RP was withdrawn and diluted to 100 μL for the HPLC analysis. The following chromatographic conditions were used for the HPLC analysis: mobile phases: Milli-Q water/0.1% (v/v) TFA (A) and ACN/0.1% (v/v) TFA (B); isocratic elution: flow rate, 1 mL min−1; temperature, 35 °C; injection volume, and 10 μL; detection was performed with the λ of 202 nm.
3. Results and discussion
Aliquat 336, ([R3NCH3]+), a chloride ion-bound anion carrier, which is effective in active anion transportation, is critical to enhance the enrichment effect of the VLPVPR peptide. The main mechanism for the transport of the VLPVPR peptide is shown in Fig. 2. The VLPVPR peptide can be deprotonated and becomes anionic when the donor phase is alkali. Then, the VLPVPR anions form ion-pairs with the cationic functional groups of the aliquat 336 molecules on the boundary between the SP and the interphase; this results in the release of chloride ion from aliquat 336. The peptide-alquat 336 ion-pair is then transported to the boundary between the interphase and the RP, where the peptides are exchanged with chloride ions from the RP; this leads to the formation of new chloride-aliquat 336 ion-pairs and the release of VLPVPR anions into the RP. In the abovementioned transport process, the chloride concentration gradients in the different interfaces provide the driving force for the enrichment of the VLPVPR peptide in addition to the pH gradient between the SP and the RP. Overall, chloride ions serve as an effective ion pump for the peptide extraction, overcoming the limitations set by the partition coefficients and achieving high enrichment of the target analyte. Considering the presence of the non-volatile NaCl in the RP, a liquid chromatography-photodiode array detector (LC-DAD) is chosen for the quantification of the VLPVPR peptide.
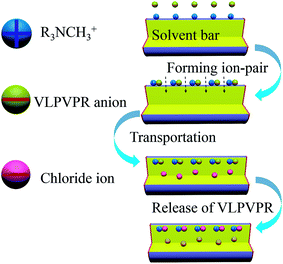 |
| Fig. 2 Schematic to explain the active transportation mechanism for VLPVPR antihypertensive peptide by aliquat 336 ([R3NCH3]+). | |
To achieve high enrichment of the VLPVPR peptide, the effects of pH (SP and RP), NaCl concentration in RP, carrier concentration, agitation rate, and extraction time were investigated, and the results have been discusses hereinafter.
3.1 Effect of pH values of SP and RP
The effect of the pH of the SP and RP on the enrichment of the VLPVPR antihypertensive peptide was studied with 10% (w/v) aliquat 336. The pH effect on the ionization of VLPVPR peptide is critical since the charge interactions form the basis for the peptide-carrier binding and dissociation. As the acid dissociation constant (pKa) of the VLPVPR peptide is close to 11, the peptide molecules become anions and then form ion-pairs with aliquat 336 in the alkali SP; this is a precondition for the carrier-mediated transport of the peptides. Similarly, in the RP, the peptides can be protonated, bearing positive charges at lower pH, and thus are released from the carrier.
The influence of the pH of the SP was evaluated at various pH values (7.4, 8.0, 9.0, 10.0, 11.0, 12.0, 13.0 and 14.0). The results in Fig. 3 indicated that the enrichment factor of the VLPVPR peptide increased rapidly until the pH value of the donor phase was 12. It proved that alkali environment was beneficial for the VLPVPR peptide to form an ion-pair with [R3NCH3]+, leading to a high enrichment factor. However, under higher pH conditions, there were more OH− ions present in the SP, which competed with the VLPVPR anions for [R3NCH3]+ cations. This is unfavorable for the transport of the VLPVPR anions and therefore a decrease in the enrichment of the VLPVPR peptide is observed when the pH of the SP is further increased. Hence, 12 is chosen as the optimal pH value of SP for the following experiments.
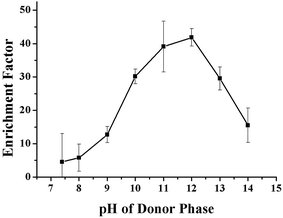 |
| Fig. 3 Effect of pH of SP on the enrichment effect of the VLPVPR antihypertensive peptide. | |
As aforementioned, the pH value of the RP is also important in determining the transport efficiency of the VLPVPR peptide, which is evaluated experimentally at various pH values (pH = 1, 2, 3, and 4). The results, as shown in Fig. 4, show that the enrichment factors are optimal at pH 2, which is used in further experiments.
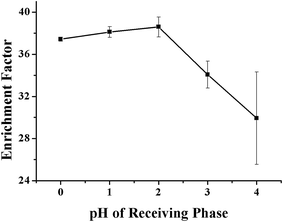 |
| Fig. 4 Effect of pH of RP on the enrichment effect of the VLPVPR antihypertensive peptide. | |
3.2 Effect of the NaCl concentration in RP
The influence of NaCl concentration on the extraction efficiency of the VLPVPR peptide was studied with regards to the hollow fiber consisting of 10% (w/v) aliquat 336 as the carrier. The concentration of NaCl varied in the range between 1, 2, 3, and 4 mol L−1. As shown in Fig. 5, an optimal enrichment effect was obtained when the concentration of NaCl was 2 mol L−1. Chloride ions in the RP could replace the VLPVPR anions in the peptide-[R3NCH3]+ ion pair, promoting the transport of VLPVPR anions from the interphase to the RP, which was important for improving the enrichment of the VLPVPR peptide and the sensitivity of the analytical methods. However, a decrease in enrichment was observed when the NaCl concentration was increased presumably due to the lower mechanical stability of the interphase (the organic solvent membrane on the hollow fiber) when the concentration of the chloride ions was too high.
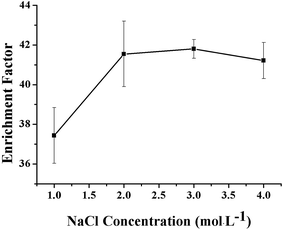 |
| Fig. 5 Effect of the NaCl concentration in RP on the enrichment effect of the VLPVPR antihypertensive peptide. | |
3.3 Effect of the carrier amount loading on the hollow fiber
The concentration of the carrier aliquat 336 was critical for the efficient enrichment of the VLPVPR peptide, which was optimized under four concentration levels: 0%, 10%, 15%, and 20% (w/v). As shown in Fig. 6, the results indicated that the enrichment factor of the VLPVPR peptide increased when the concentration of aliquat 336 increased from 0% to 15%. The enrichment factor reached its highest value (42.8) at a concentration of 15% (w/v), and thereafter, it decreased. In contrast, the enrichment factor was only 5.5 without aliquat 336; this demonstrated the important role played by the carrier in the enrichment of the VLPVPR peptide. In principle, when the concentration of aliquat 336 continues to increase, the capacity for ion transport should increase; this leads to a higher enrichment effect. However, the extraction efficiency of the VLPVPR peptide decreases when aliquat 336 is above 15% (w/v). This is mainly due to increased viscosity of the organic interphase as the concentration of aliquat 336 increases; this leads to a difficult VLPVPR release from the SP to the RP. Therefore, 15% (w/v) aliquat 336 was employed in further experiments.
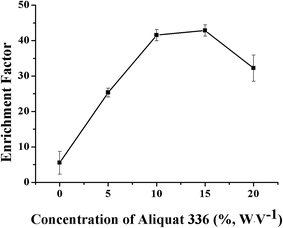 |
| Fig. 6 Effect of the aliquat 336 concentration on the enrichment effect of the VLPVPR antihypertensive peptide. | |
3.4 Effect of the agitation rate during the enrichment process
The agitation speed can influence mass transfer between the organic solvent and the sample matrix, as well as promote a fast extraction equilibrium. An increase in the agitation speed can accelerate the diffusion of the analyte from the sample solution to the extraction solvent and shorten the equilibrium time. In addition, agitation is an effective way of obtaining a suitable recovery by increasing the diffusion coefficient. Since the extraction step is the main rate-limiting step of the whole analysis procedure, a higher agitation speed is necessary to save time. The relationship between agitation speed and enrichment efficiency of the VLPVPR antihypertensive peptide was investigated with the agitation speeds of 450, 550, 650, 750, and 850 rpm (Fig. 7). The results indicate that the extraction efficiency of the VLPVPR peptide increases, and highest enrichment efficiency is obtained when the agitation speed is 650 rpm. However, the relative standard deviation (RSD) is larger when the agitation speed increases from 650 rpm to 850 rpm since: (i) higher agitation rate tends to generate bubbles attached on the surface of hollow fiber, which influences mass transfer and (ii) a higher agitation rate causes a more pounced loss of organic solvent, therefore increasing experiment errors. Thus, 650 rpm was the optimal agitation speed.
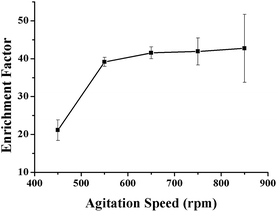 |
| Fig. 7 Effect of the agitation speed on the enrichment effect of the VLPVPR antihypertensive peptide. | |
3.5 Effect of the extraction time
The kinetics of mass transfer in the three-liquid-phase microextraction (TLPME) is highly important. For example, based on partitioning thermodynamics, the amount of analyte extracted is determined by the equilibrium time; i.e., the extraction efficiency increases as the extraction time increases until equilibrium is attained. However, the loss of the organic solvent phase within the hollow fiber becomes more severe over time; this results in decreased extraction efficiency and larger experimental error. Therefore, it is important to optimize the extraction time to realize high extraction efficiency and good reproducibility. The influence of extraction time on the enrichment factor of the VLPVPR peptide was tested by varying the time intervals from 10 to 90 min. As observed from the results (Fig. 8), the extraction of the VLPVPR peptide reaches equilibrium after 60 min. The extraction efficiency decreases, and the value of RSD increases by further increasing the extraction time. Hence, 60 min was the optimal extraction time.
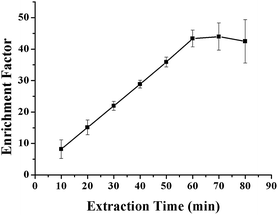 |
| Fig. 8 Effect of the extraction time on the enrichment effect of the VLPVPR antihypertensive peptide. | |
3.6 The performance of the developed methodology
An optimized methodology consists of the following parameters: linear range, detection limit (LOD, ng mL−1), precision, enrichment factor, and the percentage of free drug (Table 1). All these factors were evaluated in a standard peptide solution prepared from real plasma matrix (i.e., matrix-matched standard solutions). The chromatogram of VLPVPR in human plasma is shown in Fig. 9. A good linear correlation (R2 = 0.995) was obtained between the signal and the concentration in the range from 0.2 to 20 μg mL−1 of the VLPVPR peptide in the plasma samples. The developed method was sensitive (adsorption capacity: 10.8 mg peptide/solvent bar; LOD = 68.5 ng mL−1) and precise (RSD = 1.1% when n = 5). The enrichment factor obtained from the plasma sample was 27.5 ± 0.65, smaller than the value (43.3 ± 0.38) obtained from the buffer solution, presumably due to portion of VLPVPR antihypertensive peptide bound to the plasma proteins, thus not extractable, which was validated by the experiment; upon spiking human plasma samples with known amounts of VLPVPR peptide standard, the obtained percentage of the free drug was only 66.2 ± 0.43%, indicating significant protein binding of the VLPVPR in the plasma samples. However, the excellent linearity of the standard lines in the plasma samples demonstrates the feasibility of this technique for real sample analysis. It is noteworthy that the developed SBME method demonstrates several features of green analytical methods based on the established Analytical Eco-Scale principle 23–28. First, using a miniaturized device to integrate analyte extraction and extract clean-up in a single step, our method greatly reduces the consumption of the organic solvent as the extraction phase, thus generating less toxic waste as compared to the traditional methods, e.g., protein precipitation, organic solvent extraction, or solid-phase extraction. Second, the method is suitable for the in situ preparation of samples with minimal volumes (e.g., 100 μL), thus minimizing time and energy consumption. Furthermore, no derivatization step is required; this reduces the cost of reagents and time/energy for operation.
Table 1 Analytical characteristics of the developed method in the real sample matrix, i.e., human plasma
Linearity range (μg mL−1) |
Calibration curve (R) |
RSD (%, n = 5) |
LOD (ng mL−1) |
Enrichment factora |
Free drug percentagea (%) |
Enrichment factor and free drug (peptide) percentage were obtained in human plasma with 10 μg mL−1 of analyte.
|
0.2–20 |
y = 0.000001x + 0.3315 (0.995) |
1.1 |
68.5 |
27.5 ± 0.65 |
66.2 ± 0.43 |
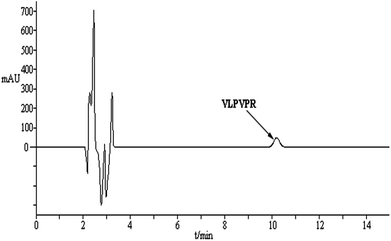 |
| Fig. 9 The chromatogram of VLPVPR in plasma obtained by the method of SBME-HPLC-DAD. | |
4. Conclusions
In this study, a loading carrier (aliquat 336)-facilitated SBME method has been developed to extract the VLPVPR antihypertensive peptide efficiently from human plasma. The feasibility of the SBME-HPLC method was validated by quantitative determination of the VLPVPR antihypertensive peptide in human plasma with sufficient specificity and reproducibility. In general, the developed SBME-HPLC method is green, effective, simple, and cost-effective and has the potential for the determination of hydrophilic peptides in various complex samples. The method is also promising for peptide-based pharmacokinetic studies, which are critical for peptide- and protein-based drug development.
Conflicts of interest
There are no conflicts of interest to declare.
Ethical statements
All experiments were performed in compliance with relevant laws or guidelines. All experiments followed institutional guidelines. We have obtained a statement of the institutional committee that approved the experiments. We have obtained consent from human subjects.
Acknowledgements
We acknowledge the financial support received from the National Natural Science Foundation of China (No. 81072615), the Project of Guangdong Province Higher Vocational College & Schools Pearl River Scholar Funded Scheme (2017), the Project of Scientific and Technological Foundation of Shenzhen (No. GJHS20120702152016267), and the Project of Scientific and Technological Foundation of Guangdong province (No. 2013B051000089).
References
- A. Chockalingam, World hypertension day and global awareness, Can. J. Cardiol., 2008, 24, 441–444 CrossRef PubMed.
- G. H. Li, G. W. Le and Y. H. Shi,
et al., Angiotensin I-converting enzyme inhibitory peptides derived from food proteins and their physiological and pharmacological effects, Nutr. Res., 2004, 24, 469–486 CrossRef CAS.
- D. Martínez-Maqueda, B. Miralles and I. Recio,
et al., Antihypertensive peptides from food proteins: a review, Food Funct., 2012, 3, 350–361 Search PubMed.
- B. Hernández-Ledesma, M. M. Contreras and I. Recio, Antihypertensive peptides: production, bioavailability and incorporation into foods, Adv. Colloid Interface Sci., 2011, 165, 23–35 CrossRef PubMed.
- H. Y. Sun, D. Liu and Y. Li,
et al., Preparation and in vitro/in vivo characterization of enteric-coated nanoparticles loaded with the antihypertensive peptide VLPVPR, Int. J. Nanomed., 2014, 9, 1709–1716 CrossRef PubMed.
- H. Y. Sun, J. Z. Luo and D. Liu, Antihypertensive effects of biodegradable microspheres loaded with recombinant antihypertensive peptide on spontaneously hypertensive rats, Chin. J. Biochem. Pharm., 2012, 33, 587–590 Search PubMed.
- X. Q. Guo, M. He and K. Nan,
et al., A dual extraction technique combined with HPLC-ICP-MS for speciation of seleno-amino acids in rice and yeast samples, J. Anal. At. Spectrom., 2016, 31, 406–414 RSC.
- P. Puchalska, M. C. García and M. L. Marina, Development of a capillary high performance liquid chromatography-ion trap-mass spectrometry method for the determination of VLIVP antihypertensive peptide in soybean crops, J. Chromatogr. A, 2014, 1338, 85–91 CrossRef CAS PubMed.
- P. Puchalska, M. L. Marina and M. C. García, Development of a reversed-phase high-performance liquid chromatography analytical methodology for the determination of antihypertensive peptides in maize crops, J. Chromatogr. A, 2012, 1234, 64–71 CrossRef CAS PubMed.
- M. M. Contreras, B. Gómez-Sala and P. J. Martín-Álvarez,
et al., Monitoring the large-scale production of the antihypertensive peptides RYLGY and AYFYPEL by HPLC-MS, Anal. Bioanal. Chem., 2010, 397, 2825–2832 CrossRef PubMed.
- J. Lee, H. K. Lee and K. E. Rasmussen,
et al., Environmental and bioanalytical applications of hollow fiber membrane liquid-phase microextraction: a review, Anal. Chim. Acta, 2008, 624, 253–268 CrossRef CAS PubMed.
- S. Pedersen-Bjergaard and K. E. Rasmussen, Liquid-phase microextraction with porous hollow fibers, a miniaturized and highly flexible format for liquid–liquid extraction, J. Chromatogr. A, 2008, 1184, 132–142 CrossRef CAS PubMed.
- A. Sarafraz-Yazdi and A. Amiri, Liquid-phase microextraction, Trends Anal. Chem., 2010, 29, 1–14 CrossRef CAS.
- L. Chimuka, M. Michel and E. Cukrowska,
et al., Advances in sample preparation using membrane-based liquid-phase microextraction techniques, Trends Anal. Chem., 2011, 11, 1781–1792 CrossRef.
- T. Kanamori, K. Sakai and T. Awaka,
et al., An improvement on the method of determining the solute permeability of hollow-fiber dialysis membranes photometrically using optical fibers and comparison of the method with ordinary techniques, J. Membr. Sci., 1994, 88, 159–165 CrossRef CAS.
- C. Yang, J. Wang and D. H. Li, Microextraction techniques for the determination of volatile and semivolatile organic compounds from plants: a review, Anal. Chim. Acta, 2013, 799, 8–22 CrossRef CAS PubMed.
- S. F. Cui, S. Tan and G. F. Ouyang,
et al., Automated polyvinylidene difluoride hollow fiber liquid-phase microextraction of flunitrazepam in plasma and urine samples for gas chromatography/tandem mass spectrometry, J. Chromatogr. A, 2009, 1216, 2241–2257 CrossRef CAS PubMed.
- S. F. Cui, G. F. Ouyang and G. J. Duan,
et al., The mass transfer dynamics of hollow fiber liquid-phase microextraction and its application for rapid analysis of biological samples, J. Chromatogr. A, 2012, 1266, 10–16 CrossRef CAS PubMed.
- S. Shariati, Y. Yamini and A. Esrafili, Carrier mediated hollow fiber liquid phase microextraction combined with HPLC-UV for preconcentration and determination of some tetracycline antibiotics, J. Chromatogr. B: Anal. Technol. Biomed. Life Sci., 2009, 877, 393–400 CrossRef CAS PubMed.
- S. Yudthavorasit, C. Chiaochan and N. Leepipatpiboon, Simultaneous determination of multi-class antibiotic residues in water using carrier-mediated
hollow-fiber liquid-phase microextraction coupled with ultra-high performance liquid chromatography tandem mass spectrometry, Microchim. Acta, 2011, 172, 39–49 CrossRef CAS.
- Y. Yamini, C. T. Reimann and A. Vatanara,
et al., Extraction and preconcentration of salbutamol and terbutaline from aqueous samples using hollow fiber supported liquid membrane containing anionic carrier, J. Chromatogr. A, 2006, 1124, 57–67 CrossRef CAS PubMed.
- J. Zhou, Y. Zhang and B. Xu,
et al., Analysis of Local Anesthetics in Biological Samples via Kinetically Calibrated Liquid-Phase Solvent Bar Micro-Extraction Combined with HPLC, Chromatographia, 2014, 77, 1213–1221 CAS.
- P. T. Anastas, Green chemistry and the role of analytical methodology development, Crit. Rev. Anal. Chem., 1999, 29, 167–175 CrossRef CAS.
- J. Namieśnik, Trends in environmental analytics and monitoring, Crit. Rev. Anal. Chem., 2000, 30, 221–269 CrossRef.
- S. Armenta, S. Garrigues and M. de la Guardia, Green analytical chemistry, Trends Anal. Chem., 2008, 27, 497–511 CrossRef CAS.
- M. Koel and M. Kaljurand, Application of the principles of green chemistry in analytical chemistry, Pure Appl. Chem., 2006, 78, 1993–2002 CrossRef CAS.
- M. Tobiszewski, A. Mechlińska and J. Namieśnik, Green analytical chemistry – theory and practice, Chem. Soc. Rev., 2010, 39, 2869–2878 RSC.
- A. Gałuszka, P. Konieczka, Z. Migaszewski and J. Namieśnik, Analytical Eco-Scale for assessing the greenness of analytical procedures, Trends Anal. Chem., 2012, 37, 61–72 CrossRef.
|
This journal is © The Royal Society of Chemistry 2018 |
Click here to see how this site uses Cookies. View our privacy policy here.