Unimolecular micelles of camptothecin-bonded hyperbranched star copolymers via β-thiopropionate linkage: synthesis and drug delivery†
Received
12th September 2015
, Accepted 19th November 2015
First published on 2nd December 2015
Abstract
In order to develop pH- and redox-responsive unimolecular micelles composed of camptothecin (CPT)-conjugated hyperbranched star copolymers via acid-labile β-thiopropionate linkage, a new monomer, methacryloyloxy-3-thiohexanoyl–CPT, is synthesized through conjugation of CPT with methacrylate via β-thiopropionate linkage, and then used in synthesis of the CPT-conjugated hyperbranched star copolymers by two steps of atom transfer radical polymerization (ATRP): self-condensation vinyl polymerization of the CPT-based monomer, 2-hydroxypropyl methacrylate and inimer, and subsequent ATRP of oligo(ethylene glycol) methacrylate using the obtained hyperbranched polymers as the macroinitiator. The obtained polymers dissolve in water to form unimolecular micelles, and their release of CPT in water at various pHs and their anticancer efficacy are studied. The CPT-loaded unimolecular micelles with diameters of 3.56–6.08 nm are quite stable under neutral environment, and are easily triggered by mild acidic pH, such as 6.0 and 5.0. They can be easily internalized by the tumor cells, releasing the CPT. The CPT-conjugated unimolecular micelles via acid-labile β-thiopropionate linkage have potential for application as tumor-targeted drug release systems.
1. Introduction
Camptothecin (CPT), which is a cytotoxic quinoline alkaloid, shows remarkable anticancer activity in preliminary clinical trials because it is an inhibitor of the DNA-replicating enzyme topoisomerase I.1 CPT is isolated from the bark and stem of Camptotheca acuminate, a Chinese native tree used as a cancer treatment in Traditional Chinese Medicine.2 Nevertheless, the low solubility of CPT in water has forced medicinal chemists to develop various derivatives for improving the performance of the drug.3–5 Polymeric carriers for CPT have been widely investigated, and there are two general methods for the preparation of CPT-loaded microspheres. One is encapsulation of CPT within the hydrophobic core of micelles or nanoparticles;6–8 the resultant microspheres enhance the stability of CPT in the active lactone form, as well as improving the water solubility. Another method is to graft the CPT onto the hydrophobic cores of micelles via a labile chemical bond; under external stimuli conditions, such as redox state of the medium or light, the labile bond can be cleaved and the CPT is released.9–13 Controlled release of the free CPT from the nanostructured polymer materials was achieved via the breaking of a photo-cleavable linker, such as 2-nitrobenzyl ester, under irradiation of 365 nm light,9 or via breaking of a reducible disulfylbutyrate linker in the presence of glutathione.10 pH-Responsive aggregates have attracted extensive attention because of their particular relevance in biological applications and the significantly lower pH of tumor cells than that of the normal cells.14,15 Thus, research on grafting CPT onto nanostructured polymer aggregates via acid-labile bonds is particularly significant.
There are already many studies on pH-sensitive polymeric microspheres, and the acid-labile covalent linkages utilized to chemically link drugs onto the polymers or assemblies include hydrazones,16–18 acetal,19–22 ketal,23 and hydrazide.24 The CPT can be linked onto the polymers by reaction of the hydroxyl group in CPT with a CHO group; however, besides the synthetic difficulty, the acetal linkage is extremely sensitive to acidic pH, and can be cleaved rapidly,25 thus, controlled release of the drug in a sustained manner is difficult. β-Thiopropionate can hydrolyze in acidic solution, which is driven by the generation of a partial positive charge on the ester carbonyl carbon owing to the inductive effect of sulfur atom (Scheme 1a),26,27 and this linkage undergoes acidic hydrolysis at a relatively slow rate compare to the acetal linkage, so controlled drug release in a sustained manner is expected. This acid-labile β-thiopropionate linkage has been utilized in pH-responsive polymer micelles,28–30 and the pH-responsive polyion complex micelles were constructed by conjugation of siRNA to the lactosylated poly(ethylene glycol) through β-thiopropionate linkage.31–33 However, studies on drug release triggered by pH through β-thiopropionate are rare; we found only one article in the literature. The micelles are formed by dissolving the paclitaxel-conjugated linear block copolymers via β-thiopropionate linkage in an aqueous solution, and showed pH-triggered drug release property.34 All the polymers used in these studies are linear, and they self-assemble to form microspheres. However, the results reported demonstrate that the branched polymeric architectures, such as dendrimers, hyperbranched polymers and dendronized polymers, possess features such as a number of surface groups and nanoscopic size, which make them attractive in drug delivery applications,35,36 and the branched structures of sufficient size display extended in vivo circulation times in comparison with their linear counterparts.37
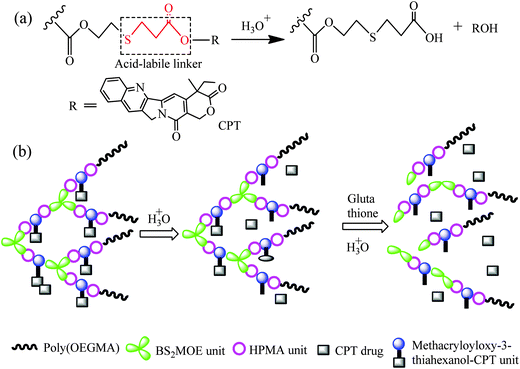 |
| Scheme 1 (a) Structure of poly(methacryloyloxy-3-thiahexanoyl–CPT) and its acid-induced cleavage; (b) schematic representation of the drug release in acidic solution and decomposition of the hyperbranched star copolymer in the presence of glutathione. | |
Herein, we introduce a novel CPT-based hyperbranched star copolymer for application in drug delivery. Scheme 1b depicts the controlled release in acidic solution and decomposition of the copolymers in the presence of glutathione. The core polymer is composed of BS2MOE, HPMA and MACPT. The disulfide is a well-known reduction-responsive linkage,38–40 thus, the BS2MOE units in the core can ensure complete renal clearance after drug delivery.9 By utilization of highly reactive bromine groups on the surface of hyperbranched polymers, a variety of targeting groups or bioactive compounds can be linked to their surface, forming various targeting drug delivery systems or improving blood compatibility. In this study, highly hydrophilic poly[oligo(ethylene glycol) methacrylate] (POEGMA) chains were attached to the surface of hyperbranched polymers through ATRP method and the resultant hyperbranched star copolymers can form unimolecular micelles in aqueous solution, and the highly hydrophilic POEGMA chains are extended into aqueous solution, shielding the hydrophobic hyperbranched polymer core. Such polymers have potential applications in tumor-targeted drug release systems.
2. Experimental section
2.1 Materials
CPT and SYTO-9 were purchased from Aladdin and Invitrogen Corporation, respectively, and both were used as received. Methacryloyl chloride, acryloyl chloride, triethylamine (Et3N) and 2-mercaptoethanol were distilled under reduced pressure. Hydroxypropyl methacrylate (HPMA, 97%, Aladdin) and oligo(ethylene glycol) methacrylate (OEGMA, Mn = 475 g mol−1, Aldrich) were purified by passing through a neutral Al2O3 column to remove the inhibitor. The HPMA is an isomer mixture of 2-hydroxypropyl methacrylate (75 mol%) and 2-hydroxyisopropyl methacrylate (25 mol%). CuBr (98%, Aldrich) was purified as reported.41N,N,N′,N′′,N′′′-Pentamethyldiethylenetriamine (PMDETA, 99%, Aladdin) was deoxygenated before use by nitrogen bubbling for 2–3 h. 2-(2′-Bromoisobutyryloxy)ethyl-2′′-methacryloyl oxyethyl disulfide (BS2MOE) was prepared according to our previous report.421H NMR (300 MHz, CDCl3, δ, ppm): 6.15 and 5.60 (s, 2H,
CH2), 4.42 (dt, 4H, 2-O–CH2), 3.02–2.94 (m, 4H, 2-S–CH2), 1.95 (dd, 3H,–CH3), and 1.94 (s, 6H, (CH3)2CBr). All other reagents were of analytical grade and were purchased from Shanghai Chemical Reagent and used without further purification.
2.2 Characterization
1H NMR measurements were carried out on a Bruker AV300 NMR spectrometer using CDCl3 or D2O or DMSO-d6 as the solvent. High-performance liquid chromatography-mass spectrometry (LC-MS) analysis was conducted on a two-dimensional liquid chromatograph-tandem mass spectrometer (2DLC-MS) from Thermo Fisher Scientific. Molecular weight and molecular weight distribution (Mw/Mn) were determined on a Waters 150C gel permeation chromatograph (GPC) equipped with two microstyragel column and an RI 2414 detector at 30 °C, monodispersed polystyrene standards were used in the calibration of Mn, Mw, and Mw/Mn, and N,N′-dimethyl formamide (DMF) was used as the eluent at a flow rate of 0.9 mL min−1. UV/Vis measurement was performed on a Unico UV/vis2802PCS spectrophotometer. Cell imaging observations were conducted on a confocal laser scanning microscope (LSM510, Zeiss and Coherent) with a 63× objective. High-performance liquid chromatography (HPLC) measurement was conducted on a liquid chromatograph (Agilent 1260). Dynamic light scattering (DLS) measurements were carried out on a DynaPro light scattering instrument (DynaPro-99E) at 25 °C with an 824.3 nm laser, and the data was analyzed with DYNAMICS V6 software.
2.3 Synthesis of methacryloyloxy-3-thiohexanoyl–CPT (MACPT)
MACPT was synthesized through three steps, including syntheses of acryloyl–CPT, 6-hydroxy-3-thiohexanoyl–CPT and MACPT (Scheme 2).
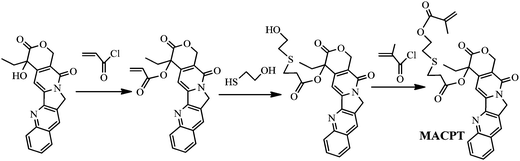 |
| Scheme 2 Synthetic route of MACPT. | |
2.3.1 Acryloyl–CPT.
Acryloyl chloride (0.447 g, 0.005 mol) was added dropwise into a suspension of CPT (0.348 g, 0.001 mol) and triethylamine (0.505 g, 0.005 mol) in dichloromethane (200 mL) in an ice bath while stirring. The reaction mixture was then stirred at room temperature for 24 h. The solvent was removed under reduced pressure to yield a yellow solid. Dilute ammonia water was added to dissolve the surplus acidoid while the acryloyl–CPT was insoluble in water and collected by filtration. The resultant product was further purified via silica column chromatography (ethyl acetate), and 0.35 g of a light yellow solid was obtained in a yield of 87%. 1H NMR (300 MHz, CDCl3, δ, ppm): 8.40 (m, 1H, ArH), 8.21 (m, 1H, ArH), 7.94 (m, 1H, ArH), 7.82 (m, 1H, ArH), 7.67 (m, 1H, ArH), 7.23 (m, 1H, ArH), 6.52 and 5.98 (d, 2H,
CH2), 6.27 (dd, 1H,
CH), 5.71 and 5.43 (m, 2H, –O–CH2), 5.29 (m, 2H, –N–CH2), 2.41–2.16 (m, 2H, –CH2), and 1.01 (t, 3H, –CH3); MS: [M − H+] = 403.12, found at 403.1286.
2.3.2 6-Hydroxy-3-thiohexanoyl–CPT.
2-Mercaptoethanol (0.340 g, 4.36 mmol) and acryloyl–CPT (0.350 g, 0.87 mmol) were dissolved in THF (40 mL). The reaction mixture was stirred at 40 °C for 12 h. The solvent was removed under reduced pressure to yield a yellow solid, and water was added to dissolve the surplus 2-mercaptoethanol while the 6-hydroxy-3-thiohexanoyl–CPT was insoluble in water and was collected by filtration (0.376 g, yield: 90%). 1H NMR (300 MHz, CDCl3, δ, ppm): 8.40 (m, 1H, ArH), 8.21 (m, 1H, ArH), 7.94 (m, 1H, ArH), 7.82 (m, 1H, ArH), 7.67 (m, 1H, ArH), 7.23 (m, 1H, ArH), 5.71 and 5.43 (m, 2H, –O–CH2), 5.29 (m, 2H, –N–CH2), 4.58 (s, 1H, –OH), 3.89 (dt, 2H, HO–CH2–), 3.01–2.83 (m, 6H, –CH2–), 2.41–2.16 (m, 2H, –CH2), and 1.01 (t, 3H, –CH3). MS: [M − H+] = 481.14, found at 481.1420.
2.3.3 Methacryloyloxy-3-thiohexanoyl–CPT (MACPT).
Methacryloyl chloride (0.409 g, 3.9 mmol) was added dropwise to a stirred suspension of 6-hydroxy-3-thiohexanoyl–CPT (0.376 g, 0.78 mmol) and triethylamine (0.395 g, 3.9 mmol) in dichloromethane (50 mL) in an ice bath. The reaction mixture was then stirred at room temperature for 24 h. The solvent was removed under reduced pressure to yield a dark yellow solid. Dilute ammonia water was added to dissolve the surplus acidoid while methacryloyl-3-thiohexanoyl–CPT was insoluble in water and could be collected by filtration. The resulting product was purified via silica column chromatography (ethyl acetate), and a dark yellow solid was obtained (0.365 mg, yield: 85%). 1H NMR (300 MHz, CDCl3, δ, ppm): 8.40 (m, 1H, ArH), 8.21 (m, 1H, ArH), 7.94 (m, 1H, ArH), 7.82 (m, 1H, ArH), 7.67 (m, 1H, ArH), 7.23 (m, 1H, ArH), 6.18 and 5.55 (d, 2H,
CH2), 5.71 and 5.43 (m, 2H, –O–CH2), 5.29 (m, 2H, –N-CH2), 4.28 (m, 1H, –O–CH2), 3.01–2.77 (m, 6H, –CH2–), 2.41–2.16 (m, 2H, –CH2), and 1.91 (m, 3H, CH3C
), 1.01 (t, 3H, –CH3). MS: [M − H+] = 549.16, found at 549.1686. Melting point = 196–198 °C.
1H NMR spectra of acryloyl–CPT, 6-hydroxy-3-thiohextyoyl–CPT and MACPT are shown in Fig. S1–S3 of the ESI,† respectively, and the characteristic signals are marked in these figures.
2.4 Synthesis of HP(HPMA-co-MACPT-co-BS2MOE)
Into a 2 mL polymerization tube, MACPT (75 mg, 0.137 mmol), HPMA (78 mg, 0.547 mmol), BS2MOE (25.2 mg, 0.0679 mmol), CuBr (0.98 mg, 0.00679 mmol), PMDETA (2.35 mg, 0.0136 mmol) and THF (0.6 mL) were added. The mixture was degassed through three freeze–pump–thaw cycles, the polymerization tube was then sealed by flame under vacuum and the sealed tube was immersed into an oil bath thermostated at 50 °C. After 48 h, the polymerization tube was cooled to room temperature rapidly, and the mixture was then dissolved in 50 mL of THF, then passed through a short neutral alumina column for removal of metal salts. The final polymer (0.157 g, yield: 88%) was obtained by evaporating most of the solvent and precipitating into diethyl ether.
2.5 Synthesis of HP(HPMA-co-MACPT-co-BS2MOE)(POEGMA)n
Into a 5 mL polymerization tube, HP(HPMA-co-MACPT-co-BS2MOE) (0.100 g, 0.0381 mmol Br), OEGMA (0.362 g, 0.762 mmol), CuBr (0.55 mg, 0.00381 mmol), PMDETA (1.32 mg, 0.00762 mmol) and THF (2 mL) were added. The mixture was degassed through three freeze–pump–thaw cycles. The polymerization tube was then sealed by flame under vacuum, and the sealed tube was immersed into an oil bath at 50 °C. After 3 h, the polymerization tube was cooled to room temperature rapidly, and the mixture was dissolved into 50 mL of THF, and then passed through a short neutral alumina column for removal of the metal salt. The final polymer (0.195 g, yield: 26.3%) was obtained by evaporating most of the solvent and precipitating in n-hexane.
2.6 Preparation of unimolecular micellar solution in water
Hyperbranched star copolymer, HP(HPMA-co-MACPT-co-BS2MOE)(POEGMA)n (10 mg), was dissolved in THF (1 mL). Under vigorous stirring, deionized water (9 mL) was added via a syringe pump at a rate of 3 mL h−1. After the addition was completed, the dispersion was stirred for another 5 h. Subsequently, THF was removed by dialysis [molecular weight (Mw) cutoff: 35 kDa] against deionized water for 24 h, and the dialysis medium was changed regularly.
The drug loading capacity (DLC) of the micelles was measured by 1H NMR spectra and UV quantitative analysis. For the UV method, a series of CPT solutions (0.005, 0.01, 0.05, 0.1, 0.25 mg mL−1) in DMSO were prepared, and then the UV absorbance of each solution was measured. The standard curve was obtained as shown in Fig. S7 (ESI†). HP(HPMA-co-MACPT-co-BS2MOE)(POEGMA)n (1 mg) was dissolved in DMSO (1 mL) and the CPT concentration was obtained based on the standard curve. The DLC was calculated according to the following formula:
DLC = (weight of loaded drug/weight of polymer) × 100% |
2.7 Drug release
The concentration of the HP(HPMA-co-MACPT-co-BS2MOE)(POEGMA)n micelle solution was adjusted to 1.0 mg mL−1 for subsequent in vitro drug release experiments. The drug release was performed under both reduction-insensitive (without DTT) and reduction-sensitive (with DTT) conditions. For each condition, the drug release was performed at pH 5.0, 6.0 and 7.4. A typical procedure is as follows. An aqueous dispersion (3.0 mL) of CPT-loaded micelles (1.0 mg mL−1) was transferred to a dialysis bag with a molecular weight cutoff of 3.5 kDa and then immersed in 60 mL of buffer solution with 10 mM DTT at pH 5.0 or 6.0 or 7.4, which was purged with pure nitrogen for 30 min. Samples (3.0 mL) were taken at predetermined time intervals (2 h) to determine the amount of drug released by ultraviolet quantitative analysis, and the solution out of the dialysis bag was refilled with 3.0 mL of the fresh buffer solution. This procedure continued for 3 days, and the buffer solutions were collected. Finally, the hydrolysis product was obtained by lyophilization for verification of its structure. The same method was employed for the reduction-insensitive experiment. UV absorbance of the dialysis solution was measured at 365 nm.
2.8 Cell viability assay
In vitro cytotoxicity was examined by MTT assay. HepG2 (or HeLa) cells were seeded in a 96-well plate with an initial density of 5000 cells per well in 100 μL of complete Dulbecco’s modified Eagle’s medium (DMEM) supplemented with 10% fetal bovine serum (FBS) at 37 °C under an atmosphere with 5% CO2. A micellar solution of the HP(HPMA-co-MACPT-co-BS2MOE)(POEGMA)n in phosphate buffered saline (PBS, 50 mM, pH 7.4) was added to achieve various polymer concentrations, while the plate with different concentrations of the unimolecular micelles was used and the plate without the polymers was used as the control. After incubation for 24 h, MTT reagent (in 20 μL of PBS buffer, 5 mg mL−1) was added to each well, and the cells were further incubated with 5% CO2 for 4 h at 37 °C. The culture medium in each well was removed and replaced by 100 μL of DMSO. The plate was gently agitated for 15 min and the optical densities (OD) were recorded at a wavelength of 490 nm using a Thermo Electron MK3 spectrophotometer. The cell viability was calculated according to the following equation:
Cell viability% = (ODsample/ODcontrol) × 100% |
where ODsample represents an OD value from a well treated with samples and ODcontrol from a well treated with PBS buffer only. Each experiment was carried out in sextuplet. Mean values and the corresponding standard deviations (mean ± SD) were obtained. The same experiment was done using HeLa cells.
2.9 Cellular uptake and imaging
A cellular uptake and imaging assay of the CPT-loaded unimolecular micelles prepared from HP(HPMA-co-MACPT-co-BS2MOE)(POEGMA)n was carried out on the human hepatoma cells (HepG2). The HepG2 cells (5 × 104) were seeded on a coverslip and then incubated in 500 μL of DMEM containing 100 μL of CPT-loaded HP(HPMA-co-MACPT-co-BS2MOE)(POEGMA)n micellar solution (3 mg mL−1 in deionized water) at 37 °C. After being treated for 2 h, the culture medium was removed, and after being rinsed with PBS twice the cells were fixed with formaldehyde, and the cells were stained with STYO 9 (green). The cells were rinsed with PBS buffer and were then observed using a confocal laser scanning microscope (LSM510, Zeiss and Coherent) at 430 nm (Ex = 405 nm).
3. Results and discussion
As mentioned in the introduction, the β-thiopropionate linker is pH-sensitive and undergoes hydrolysis in acidic solution, which is essential for application in controlled drug release. Thus, we designed a new monomer, MACPT, in which CPT is linked to the methacrylate via acid-labile β-thiopropionate, for synthesis of pH-sensitive CPT-loaded hyperbranched star copolymers, HP(HPMA-co-MACPT-co-BS2MOE)(POEGMA)n. As shown in Scheme 2, the monomer MACPT was synthesized through three steps: reaction of acryloyl chloride with the hydroxyl group of CPT; Michael addition reaction between acryloyl–CPT and mercaptoethanol; and esterification reaction of 6-hydroxyl-3-thiohexanoyl–CPT with methacryloyl chloride. The structures of the two intermediates are confirmed by 1H NMR spectra (Fig. S1 and S2, ESI†). The proton signals of the final product in Fig. S3 (ESI†) prove the formation of MACPT: vinyl proton signals of the methacrylate at δ = 6.18 and 5.55 ppm (n), methylene protons of the β-thiopropionate linker next to sulfur at δ = 2.9 ppm (k) and aromatic protons of CPT at δ = 7.2–8.8 ppm. Mass spectra also support successful synthesis of the intermediates and MACPT. However, the MACPT is hardly homo-polymerized, probably due to its steric hindrance. So, HPMA was selected as a comonomer owing to its low toxicity. In order to prepare the unimolecular polymeric micelles in aqueous solution, a strongly hydrophilic shell, which can stabilize the micelles in water, is highly required. OEGMA has been widely used in preparation of the amphiphilic block copolymers due to its favorable hydrophilicity and very low cytotoxicity. Therefore, the hyperbranched star copolymer HP(HPMA-co-MACPT-co-BS2MOE)(POEGMA)ns was synthesized according to Scheme 3. The hyperbranched star copolymer HP(HPMA-co-BS2MOE)(POEGMA)ns without the CPT was used as contrast.
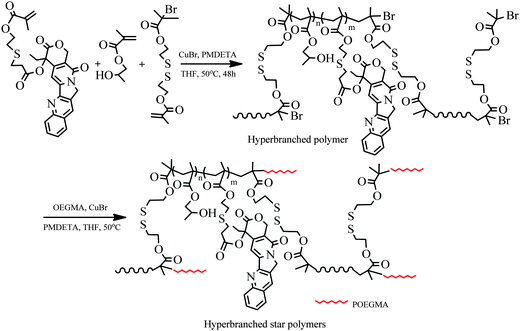 |
| Scheme 3 Synthesis of hyperbranched copolymer HP(HPMA-co-MACPT-co-BS2MOE) and hyperbranched star copolymer HP(HPMA-co-MACPT-co-BS2MOE)(POEGMA)n. | |
3.1 Synthesis and characterization of HP(HPMA-co-MACPT-co-BS2MOE)(POEGMA)n
The first step for synthesis of the hyperbranched star copolymers is preparation of the macroinitiator, HP(HPMA-co-MACPT-co-BS2MOE), which was synthesized by ATRP of HPMA, MACPT and the inimer BS2MOE; the detailed polymerization conditions and the results are listed in Table 1. The molecular weights and molecular weight distributions were measured by GPC, and the GPC curves are shown in Fig. S5 and S6 (ESI†). Similar to the hyperbranched polymers prepared by self-condensing vinyl polymerization (SCVP), all the obtained polymers reveal broad molecular weight distributions (2.61–3.12).
Table 1 Preparation and results of the HP(HPMA-co-MACPT-co-BS2MOE)s
Samplea |
Inimer |
Feed molar ratio of [M]/[H]/[B]/[CuBr]/[PMDETA]b |
Yieldc (%) |
M
n
(g mol−1) |
M
w/Mnd |
Molar ratio of [M]/[H]/[B] in polymere |
NBUf |
H0, H1, H2 and H3 represent HP(HPMA-co-BS2MOE) and HP(HPMA-co-MACPT-co-BS2MOE) with various feed molar ratios. Polymerization temperature: 50 °C; time: 48 h.
M, H and B represent MACPT, HPMA and BS2MOE, respectively.
Measured by gravimetric method.
Determined by GPC method.
Molar ratios of MACPT : HPMA : BS2MOE were calculated according to the following equation: [MACPT] : [HPMA] : [BS2MOE] = Ih/10 : Id/2.75 : [(2Ia − 3Ib)/12], where Ia, Ib, Id and Ih are the integral values of the signals at δ = 0.5–1.3 ppm (a), 1.6–2.1 ppm (b), 3.2–4.0 ppm (d) and 5.3–8.8 ppm (h), respectively.
NBU: average number of branching units per macromolecule is calculated according to the following equation: NBU = [(2Ia − 3Ib)/6]/Iq, where Iq is the integral value of the signals at δ = 5.65 and 6.22 ppm (q).
|
H0 |
BS2MOE |
0 : 1 : 0.1 : 0.01 : 0.02 |
90 |
16 200 |
2.72 |
0 : 1 : 0.096 |
30 |
H1 |
BS2MOE |
1 : 4 : 0.5 : 0.05 : 0.1 |
88 |
11 000 |
3.12 |
1 : 5.2 : 0.61 |
23 |
H2 |
BS2MOE |
1 : 8 : 0.9 : 0.09 : 0.18 |
85 |
14 400 |
2.75 |
1 : 10.7 : 1.12 |
24 |
H3 |
BS2MOE |
1 : 20 : 2.1 : 0.21 : 0.42 |
87 |
15 800 |
2.61 |
1 : 31 : 3.1 |
28 |
To verify the structure of the obtained products, 1H NMR spectra of the polymers were measured and a typical spectrum of the hyperbranched polymer H1 is shown in Fig. 1a. HPMA is composed of two isomers, 2-hydroxypropyl methacrylate and 2-hydroxyisopropyl methacrylate (HiPMA). The proton signal of ester methine in the HiPMA unit appears at δ = 4.49 ppm (f); the proton signals of ester methylene, methylene and methine next to the hydroxyl group appear at δ = 3.2–4.0 ppm (d); the signals of the aromatic protons and ester methylene in the MACPT units appear at δ = 7.2–8.8 and 5.6–5.9 ppm, respectively (h); the proton signals of the methylene next to the sulfur of the BS2MOE units appear at δ = 2.6–3.1 ppm (c). The compositions of the hyperbranched copolymers were calculated based on integral values of the proton signals at δ = 5.3–8.8 (h), δ = 3.2–4.0 ppm (d) and the methyl and methylene proton signals in the main chains at δ = 0.5–1.3 (a) and 1.6–2.1 ppm (b); the results are listed in Table 1. The contents of MACPT in the polymers were lower than those of the corresponding recipe, indicating that the polymerization activity of MACPT is lower than that of HPMA, probably due to steric hindrance of the bulky side group CPT. Theoretically, every hyperbranched polymer prepared by SCVP of the inimers should contain one vinyl group, thus, the number of branched units in every macromolecule (NBU) can be estimated based on the integral values of vinyl proton signals at δ = 5.65 and 6.22 ppm (Iq), and the methyl proton signal in the reacted initiating site of BS2MOE [Ia − (3/2)Ib]; the results are listed in Table 1. The NBU ranging from 23 to 30 supports the hyperbranched structure of the resultant polymers. The methyl proton signal of bromoisobutyrate at δ = 1.95 ppm is almost disappeared in Fig. 1a, indicating its high initiation efficiency, which is consistent with the reported result.43 Therefore, the NBU is almost the same as the number of initiating sites per macromolecule, although probably the latter is slightly underestimated, and the hyperbranched polymers listed in Table 1 were used as macroinitiators in the subsequent preparation of hyperbranched star polymers.
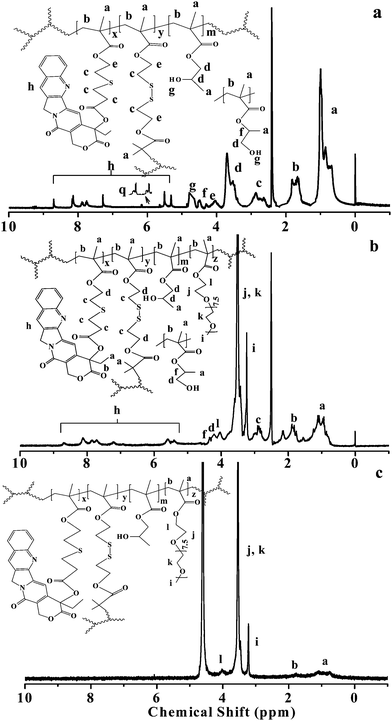 |
| Fig. 1
1H NMR spectra of (a) the hyperbranched copolymer H1, HP(HPMA-co-MACPT-co- BS2MOE) recorded in DMSO-d6; (b) the hyperbranched star copolymer H4, HP(HPMA-co- MACPT-co-BS2MOE)(POEGMA)n recorded in DMSO-d6; and (c) the same H4 recorded in D2O. | |
Since the obtained hyperbranched polymers have a large quantity of reactive bromine groups on their surface, they can be conveniently modified based on the requirements of their applications. High solubility of the hyperbranched copolymers in water is a requisite for their application as drug carriers, owing to improving their water solubility. POEGMA was selected as the surface polymer of the hyperbranched star copolymers because it is water-soluble, nontoxic, and biocompatible.44 The HP(HPMA-co-MACPT-co-BS2MOE)(POEGMA)ns were prepared by ATRP of OEGMA using HP(HPMA-co-MACPT-co-BS2MOE) as the macroinitiator. Generally, increasing the molecular weight of the POEGMA chains will decrease the content of CPT in the resulting polymers; thus, the polymerization with a feed molar ratio of OEGMA/Br = 20/1 was stopped at low conversion, and the detailed polymerization conditions and the results are listed in Table 2. To confirm the structures of products, and to estimate their composition, 1H NMR measurements were conducted, and the resulting spectra are shown in Fig. 1b and Fig. S4 (ESI†). Fig. 1b clearly shows the proton signals of the OEGMA unit besides protons signals of HP(HPMA-co-MACPT-co-BS2MOE). The proton signals of ester methylene and ether methyl of the OEGMA units appear at δ = 4.0 (l) and δ = 3.2 (i) ppm, respectively, and the signal at δ = 3.3–4.0 ppm (j, k) is significantly strengthened, indicating successful synthesis of the target polymers. Based on the integral value of the proton signals at δ = 3.2 (i) and 5.3–8.8 (h) ppm, as well as the composition of their corresponding precursors, the compositions of the resultant hyperbranched star copolymers, H00, H4, H5 and H6, which were prepared by ATRP of OEGMA using H0, H1, H2 and H3 as macroinitiators, respectively, were calculated and the results are listed in Table 2. The Mw, Mn and Mw/Mn of the hyperbranched star copolymers were measured by GPC, and their GPC traces are shown in Fig. S6 (ESI†). Compared to the GPC traces of H0, H1, H2 and H3 in Fig. S5 (ESI†), all GPC traces of the H00, H4, H5 and H6 are shifted to the higher molecular weight region. Thus, the hyperbranched star copolymers, HP(HPMA-co-MACPT-co-BS2MOE)(POEGMA)ns, were successfully synthesized and were used in the following studies.
Table 2 Preparation and results of HP(HPMA-co-MACPT-co-BS2MOE)(POEGMA)ns
Samplea |
Macro-initiator |
M
n
(g mol−1) |
M
w/Mnb |
Molar ratio of [M] : [H] : [B] : [O] in polymerc |
DLCUVd (wt%) |
DLCNMRe (wt%) |
D
(nm) |
H00, H4, H5 and H6 represent HP(HPMA-co-BS2MOE)(POEGMA)n, and HP(HPMA-co-MACPT-co-BS2MOE) (POEGMA)n 1–3; All polymerizations with feed molar ratio of [Macroinitiator] : [OEGMA] : [CuBr] : [PMDETA] = 1 : 20 : 0.1 : 0.2 were performed at 50 °C for 3 h.
Determined by GPC, the Mn and Mw/Mn were calculated based on the polystyrene standards.
M, H, B and O refer to MACPT, HPMA, BS2MOE and OEGMA, respectively. The molar ratios of [M] : [O] were calculated according to the equation: [MACPT] : [OEGMA] = (Ih/10) : (Ii/3), where Ii and Ih are the integral values of the signals at δ = 3.2 ppm (i) and at δ = 5.3–8.8 ppm (h), respectively. Subsequently, assuming that the composition of HP(HPMA-co-MACPT-co-BS2MOE)s does not change during the ATRP of OEGMA, the molar ratios of [M] : [H] : [B] : [O] were calculated.
DLC refers to the drug loading capacity, that is, wt% of the CPT in the polymers determined by ultraviolet quantitative analysis.
Calculated based on the molar ratio of MACPT in HP(HPMA-co-MACPT-co-BS2MOE).
D refers to average diameter of the micelles dissolved in water, which was determined by DLS.
|
H00 |
H0 |
36 300 |
1.89 |
0 : 1 : 0.096 : 1.2 |
0 |
0 |
6.32 |
H4 |
H1 |
20 300 |
1.98 |
1 : 5.2 : 0.61 : 2.0 |
16.7 |
14.1 |
3.56 |
H5 |
H2 |
26 700 |
1.80 |
1 : 10.7 : 1.12 : 6.0 |
7.8 |
6.5 |
4.24 |
H6 |
H3 |
36 000 |
1.87 |
1 : 31 : 3.1 : 46 |
1.3 |
1.2 |
6.08 |
3.2 Dispersion behavior of hyperbranched star copolymers in water
The hyperbranched copolymers, HP(HPMA-co-MACPT-co-BS2MOE)s, are insoluble in water due to highly hydrophilic POEGMA. The HP(HPMA-co-MACPT-co-BS2MOE)(POEGMA)ns are dissolved in water forming unimolecular micelles with a HP(HPMA-co-MACPT-co-BS2MOE) core and a POEGMA shell. To validate the formation of core-shell micelles, 1H NMR measurements of the H4 in Table 2 were conducted in DMSO-d6 and in D2O. When DMSO-d6 was used as the solvent, the characteristic proton signals of HP(HPMA-co-MACPT-co-BS2MOE)(POEGMA)n appear in its 1H NMR spectrum of Fig. 1b. However, in D2O, only the characteristic proton signals of the POEGMA chain appear and the proton signals of HP(HPMA-co-MACPT-co-BS2MOE) are absent, as shown in Fig. 1c, demonstrating the formation of micelles with the hydrophobic HP(HPMA-co-MACPT-co-BS2MOE) core and the hydrophilic POEGMA shell. To further characterize the formed micelles, the DLS was used to detect size of the micelles; the DLS curves are shown in Fig. 2 and their sizes are listed in Table 2. The results reveal that average diameters (Ds) of the resultant H4, H5, H6 and H00 micelles are 3.56, 4.24, 6.08 and 6.32 nm, respectively, which are consistent with the order of their molecular weights. Thus, the hyperbranched star copolymers can form unimolecular micelles in water.
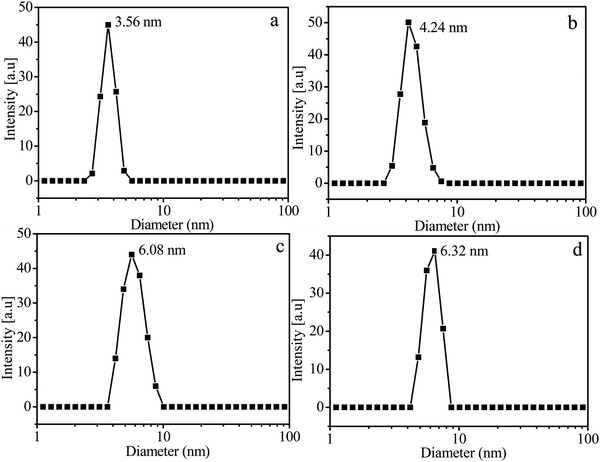 |
| Fig. 2 DLS curves of the micelles prepared from the hyperbranched star copolymers, H4 (a), H5 (b), H6 (c) and H00 (d). | |
The DLC of micelles is a very important factor for drug release. Since CPT has characteristic ultraviolet absorption at v = 365 cm−1, the ultraviolet quantitative analysis was used to measure DLC of the resultant unimolecular micelles, and the standard curve in Fig. S7 (ESI†) was used in estimation of DLC. The DLCs of H4, H5 and H6 unimolecular micelles are 16.7 wt%, 7.8 wt% and 1.3 wt%, respectively (Table 2), which is consistent with the decrease of the MACPT content in the hyperbranched star copolymers from H4 to H6 (Fig. S4, ESI†). The DLC was also estimated by 1H NMR data and the results listed in Table 2 reveal that the DLCs obtained by both methods are close to each other. Therefore, unimolecular micelles with various DLCs have been successfully prepared, and their DLCs can be easily adjusted by varying the recipe. All these micellar samples in Table 2 were used in the following study of drug release.
3.3 Release of CPT from unimolecular micelles
Since CPT is linked to the hyperbranched star copolymers via an acid-labile β-thiopropionate (Scheme 3), the unimolecular micelles are expected to be pH-sensitive. In addition, the hyperbranched star copolymers may also be redox-responsive due to the disulfide linkage in the BS2MOE branching units. Reducing agents, such as glutathione and DTT, can degrade the disulfide-containing polymers,45,46 and this type of redox-sensitive polymer has been extensively used in drug delivery systems.40,47 The hyperbranched star copolymer (H4) was treated with an aqueous solution containing 10 mM DTT for 6 h, then the degradation products were analyzed by GPC. For comparison, GPC traces of both the H4 and its degradation products are shown in Fig. 3, and the molecular weight (Mn) of the products obtained after treatment with DTT is significantly decreased from 20
300 g mol−1 to 2100 g mol−1 due to cleavage of the disulfide linkage in the branching units. Therefore, the unimolecular micelles containing anticancer drug CPT are redox-sensitive, and the degraded products may be easily cleaned after drug delivery based on the previous results.9
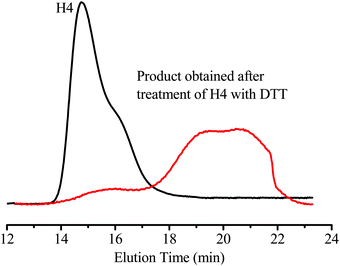 |
| Fig. 3 GPC traces of HP(HPMA-co-MACPT-co-BS2MOE)(POEGMA)n (H4) with Mn = 20 300 g mol−1 and Mw/Mn = 1.98 and the degradation products obtained after treatment of H4 in an aqueous solution containing 10 mM DTT for 6 h (Mn = 2100 g mol−1, Mw/Mn = 8.15). | |
To understand the drug release behaviors of the unimolecular micelles, we investigated the release of CPT from the micelles at different conditions, and the typical release profiles of the H4 micelles are shown in Fig. 4. When the release was conducted in an aqueous solution at pH 7.4 with or without DTT, a few percent of the CPT in the drug carriers (9 wt% with 10 mM DTT and 8 wt% without DTT) was released, and the obvious contribution of the reducing agent DTT to the drug release is not observed (Fig. 4a and b) because the CPT is covalently bonded to the polymer chains via β-thiopropionate linkage, which is stable in the neutral solution. However, for controlled release of the drug in acidic solutions (pH 5 or 6) with and without 10 mM DTT, the drug release curves in Fig. 4 exhibit similar behavior; at the beginning, the drug release rate is relatively fast, and after ca. 29 h, the drug is released at a relatively slow rate. Different from the drug-conjugated micelles via other acid-labile linkages, such as acetal linkage, which is extremely sensitive to acid and can be rapidly cleaved to release the drug,19,26 the CPT-conjugated unimoleclar micelles via β-thiopropionate linkage display controlled release of the drug in a sustained manner because this bond is hydrolyzed in acidic pH at a slow and sustained rate. As shown in Fig. 4, when the release is conducted in a solution at pH 6.0 without and with 10 mM DTT, the initial release rates are 3.27 and 3.69 μg h−1; after ca. 29 h of drug release, the release rates decrease to 0.88 μg h−1 in the absence of DTT and 1.13 μg h−1 in the presence of DTT, as shown in Fig. 4c and d. When the pH values of the solutions decreases to 5.0, the drug release rates significantly increase, and we also see an inflexion at approximately 29 h of the drug release in the curves e and f of Fig. 4. The release rates before and after 29 h of the CPT release are 6.39 and 1.06 μg h−1, respectively, under reduction-insensitive conditions, and 7.67 and 1.43 μg h−1 under reduction-sensitive conditions, respectively. The relatively high drug release rates in the initial 29 h are probably due to the relatively easy attack of the H3+O on the surface β-thiopropionate linkages of the core, and slow hydrolysis of the β-thiopropionate linkages inside the core may lead to slow release of the CPT. After controlled release without and with 10 mM DTT was conducted for 5 days, approximately 58% and 65% of the CPT in the micelles was released, respectively, and the reason for the slight increase of the released drug in the presence of DTT is mainly that degradation of the polymer chains benefits attack of the H3+O to β-thiopropionate linkage.
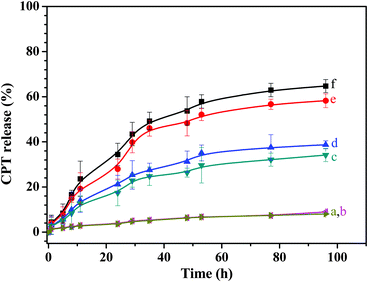 |
| Fig. 4 CPT release profiles of the hyperbranched star copolymer H4 micelles in the aqueous buffer solution under different conditions: at pH = 7.4 without DTT (a); pH = 7.4 with 10 mM DTT (b); pH = 6.0 without DTT (c); pH = 6.0 with 10 mM DTT (d); pH = 5.0 without DTT (e); pH = 5.0 with 10 mM DTT (f). | |
Another problem concerning us is whether the structure of the released CPT is the same as the original drug because any structural change will diminish, or even lose antitumor activity, for example, an intact hydroxyl lactone is essential for antitumor activity.3 Besides 1H NMR spectra of the released and the original CPT, HPLC was used to characterize the released CPT, and the HPLC curves of the pure CPT and the released molecules are shown in Fig. 5. Both the pure CPT and the released CPT from H4 micelles have the same retention time, demonstrating that the drug CPT is not changed during the release process, which is consistent with the 1H NMR results. Therefore, the drug vector obtained can unload the original drug strictly according to the reaction shown in Scheme 1a without any superfluous reaction.
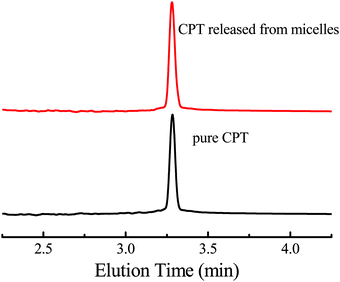 |
| Fig. 5 HPLC curves of the pure CPT and the CPT released from H4 micelles at pH 5.0. | |
3.4 Cytotoxicity of unimolecular micelles
The cytotoxicity of H00 micelles was evaluated by MTT assay using HepG2 and HeLa cells. The cells were incubated with various concentrations of micellar solutions at 37 °C for 24 h, while the cells treated with PBS solution without the micelles were used as a control. The results in Fig. 6 demonstrate that the micelles of HP(HPMA-co-BS2MOE)(POEGMA)n without CPT display low toxicity up to 2 mg mL−1 of the hyperbranched star copolymer. Fig. 7 shows the relationship of the free CPT and the CPT-loaded H4 micelles with cell viability, and the results reveal that, in the solution at pH 7.4, the free CPT displays similar dose-response curves to the HepG2 and HeLa cells, and the CPT concentrations for 50% cell viability (IC50) of the HepG2 and the HeLa cells are ∼1.36 μg mL−1 (HepG2) and ∼1.24 μg mL−1 (HeLa), respectively. The CPT-loaded H4 unimolecular micelles have almost the same dose-response curves as the HepG2 and HeLa cells, and their IC50 values are ∼2.62 μg mL−1 (HepG2) and ∼2.68 μg mL−1 (HeLa), which are very close. Thus, the CPT-loaded micelles show 1.93- (HepG2) or 2.16-fold (HeLa) lower cytotoxicity than the free CPT drug, which is ascribed to controlled release of the CPT from the unimolecular micelles, and this explanation is confirmed by the cell imaging in the following section.
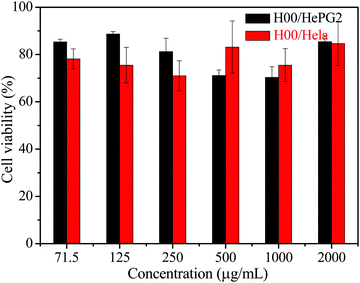 |
| Fig. 6 Relative cell viability of the HeLa and HePG2 cells evaluated by MTT assay after incubation with various concentrations of HP(HPMA-co-BS2MOE)(POEGMA)n (H00) micellar solution at pH 7.4. | |
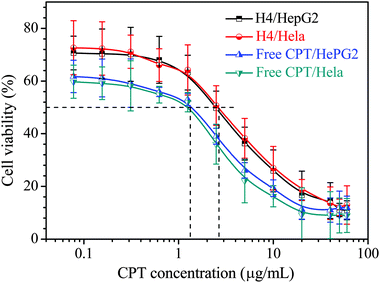 |
| Fig. 7 Relative cell viability of HePG2 cells and HeLa cells evaluated by MTT assay after incubation with micelle solution of CPT-loaded HP(HPMA-co-MACPT-co-BS2MOE) (POEGMA)n (CPT-loaded H4) at pH 7.4; and free DOX at pH 7.4. Incubating temperature: 37 °C; time: 24 h. | |
3.5 Cellular uptake of unimolecular micelles and cell imaging
In order to investigate whether the unimolecular micelles can be successfully internalized by the HepG2 cell through endocytosis, we used a confocal laser scanning microscope (CLSM) to study cellular uptake assay of the CPT solution or the CPT-loaded H4 micelles. After the HepG2 cells were incubated with DMEM medium containing the CPT-loaded H4 micelles or containing free CPT solution for 2 h, and then stained with STYO 9 (green), the fluorescence images of the HepG2 cells were acquired under UV irradiation at 405 nm or 483 nm. Because CPT emits blue fluorescence, the STYO 9 stain, which has a high affinity for DNA, was selected for staining the cell nuclei. The CLSM images in Fig. 8 reveal that the CPT blue fluorescence appears mainly at the perinuclear region of the HepG2 cells in both cases, but the cells including nuclei exhibit the STYO 9 green fluorescence. When we carefully analyzed the CLSM images, the cell nuclei incubated with the CPT-loaded H4 micelles displayed relatively weaker CPT blue fluorescence, as shown in Fig. 8B, compared to the images of the HepG2 cells treated with free CPT in Fig. 8A. The probable reason is controlled release of the CPT from the unimolecular micelles. The results demonstrate that the CPT-loaded H4 micelles have been successfully internalized by the HepG2 cells and the CPT is released from the micelles at a controlled rate, which is consistent with the results of cytotoxicity.
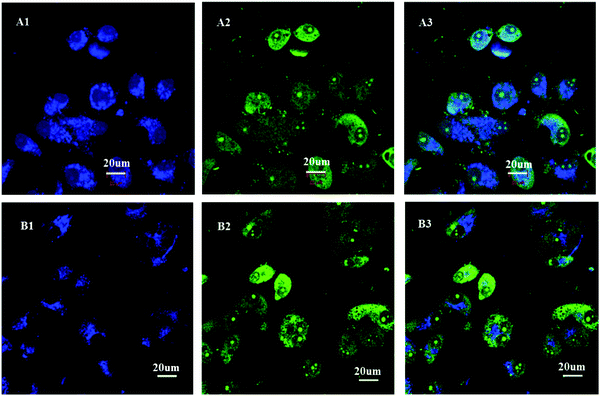 |
| Fig. 8 Confocal laser scanning microscope images of the HepG2 cells treated with the free CPT solution (A) and the H4 unimolecular micellar solution (B) at 37 °C for 2 h. The images from left to right show CPT (blue), SYTO 9 (green) and a merge of the two preceding images. | |
4. Conclusions
A new monomer, MACPT, in which the methacrylate group and the anticancer drug CPT are linked via acidic-labile β-thiopropionate linkage, has been successfully synthesized. Since the disulfide bond in the BS2MOE monomer is redox-responsive, the hyperbranched star copolymers (HSPs), HP(HPMA-co-MACPT-co-BS2MOE)(POEGMA)ns possess pH- and redox-responsive properties. Owing to the highly hydrophilic property of the POEGMA, the HP(HPMA-co-MACPT-co-BS2MOE) (POEGMA)ns are well dissolved in water to form unimolecular micelles with diameters of 3.56–6.08 nm based on their molecular weights. Controlled release of CPT from the micelles is conveniently triggered by mild acidic pH (6.0 or 5.0), and the released CPT remains its anticancer ability because no change of the CPT structure occurs during the release process. Due to hydrolysis of the β-thiopropionate linkage in acidic pH at a sustained rate, the CPT is released from the micelles in a sustained manner, and because the controlled release of CPT is pH dependent, the release rate is much faster in an acidic buffer solution of pH 5.0 than that in a neutral solution. The HSPs micelles are of low cytotoxicity, and the CPT-loaded micelles display lower cytotoxicity (IC50 = ∼2.62 μg mL−1 for HepG2 and ∼2.68 μg mL−1 for HeLa) than the free CPT (IC50 = ∼1.36 μg mL−1 for HepG2 and ∼1.24 μg mL−1 for HeLa) owing to controlled release of the CPT. The CPT-loaded micelles can be successfully internalized by the HepG2 cells. Since extracellular pH at a solid tumor site is usually weak acidic, the micelles we prepared have potential application in tumor-targeted drug release systems.
Acknowledgements
The authors are grateful for the financial support from the National Natural Science Foundation of China under contract No. 21090354 and 21374107, and the Fundamental Research Funds for the Central Universities (WK 2060200012).
References
- T. Efferth, Y. J. Fu, Y. G. Zu, G. Schwarz, V. S. B. Konkimalla and M. Wink, Curr. Med. Chem., 2007, 14, 2024–2032 CrossRef CAS PubMed.
- M. E. Wall, M. C. Wani, C. E. Cook, K. H. Palmer, A. T. Mcphail and G. A. Sim, J. Am. Chem. Soc., 1966, 88, 3888–3890 CrossRef CAS.
- W. D. Kingsbury, J. C. Boehm, D. R. Jakas, K. G. Holden, S. M. Hecht, G. Gallagher, M. J. Caranfa, F. L. McCabe, L. F Faucette, R. K. Johnson and R. P. Hertzberg, J. Med. Chem., 1991, 34, 98–107 CrossRef CAS PubMed.
- M. Berrada, A. Serreqi, F. Dabbarh, A. Owusu, A. Gupta and S. Lehnert, Biomaterials, 2005, 26, 2115–2120 CrossRef CAS PubMed.
- Z. H. Mi and T. G. Burke, Biochemistry, 1994, 33, 10325–10336 CrossRef CAS PubMed.
- M. Dai, X. Xu, J. Song, S. Z. Fu, M. L. Gou, F. Luo and Z. Y. Qian, Cancer Lett., 2011, 312, 189–196 CrossRef CAS PubMed.
- S. D. Kong, M. Sartor, C. M. Hu, W. Zhang, L. Zhang and S. Jin, Acta Biomater., 2013, 9, 5447–5452 CrossRef PubMed.
- K. Juntapram, N. Praphairaksit, K. Siraleartmukul and N. Muangsin, Carbohydr. Polym., 2012, 90, 1469–1479 CrossRef CAS PubMed.
- J. A. Johnson, Y. Y. Lu, A. O. Burts, Y. Xia, A. C. Durrell, D. A. Tirrell and R. H. Grubbs, Macromolecules, 2010, 43, 10326–10335 CrossRef CAS PubMed.
- A. G. Cheetham, P. Zhang, Y. A Lin, L. L. Lock and H. Cui, J. Am. Chem. Soc., 2013, 135, 2907–2910 CrossRef CAS PubMed.
- J. K. Cho, C. Chun, H. J. Kuh and S. C. Song, Eur. J. Pharm. Biopharm., 2012, 81, 582–590 CrossRef CAS PubMed.
- X. Chen, S. Mcrae, S. Parelkar and T. Emrick, Bioconjugate Chem., 2009, 20, 2331–2341 CrossRef CAS PubMed.
- Z. Zhou, X. Ma, E. Jin, J. Tang, M. Sui, Y. Shen, E. A. Van Kirk, W. J. Murdoch and M. Radosz, Biomaterials, 2013, 34, 5722–5735 CrossRef CAS PubMed.
- J. Ding, W. Xu, Y. Zhang, D. Sun, C. Xiao, D. Liu, X. Zhu and X. Chen, J. Controlled Release, 2013, 172, 444–455 CrossRef CAS PubMed.
- F. Shi, J. Ding, C. Xiao, X. Zhuang, C. He, L. Chen and X. Chen, J. Mater. Chem., 2012, 22, 14168–14179 RSC.
- T. Etrych, M. JelíNková, B. ŘíHová and K. Ulbrich, J. Controlled Release, 2001, 73, 89–102 CrossRef CAS PubMed.
- Y. Bae, S. Fukushima, A. Harada and K. Kataoka, Angew. Chem., Int. Ed., 2003, 42, 4640–4643 CrossRef CAS PubMed.
- M. Hruby, C. Konak and K. Ulbrich, J. Controlled Release, 2005, 103, 137–148 CrossRef CAS PubMed.
- X. Tang and C. Y. Pan, J. Biomed. Mater. Res., Part A, 2008, 86, 428–438 CrossRef PubMed.
- E. R. Gillies and J. M. J. Fréchet, Chem. Commun., 2003, 1640–1641 RSC.
- Y. J. Rui, S. Wang, P. S. Low and D. H. Thompson, J. Am. Chem. Soc., 1998, 120, 11213–11218 CrossRef CAS.
- E. R. Gillies and J. M. j. Frechet, Bioconjugate Chem., 2005, 16, 361–368 CrossRef CAS PubMed.
- D. A. Jaeger and Y. M. Sayed, J. Org. Chem., 1993, 58, 2619–2627 CrossRef CAS.
- Y. Bae, W. D. Jang, N. Nishiyama, S. Fukushima and K. Kataoka, Mol. BioSyst., 2005, 1, 242–250 RSC.
- T. H. Fife and L. K. Jao, J. Org. Chem., 1965, 30, 1492–1495 CrossRef CAS.
- K. Dan, R. Pan and S. Ghosh, Langmuir, 2011, 27, 612–617 CrossRef CAS PubMed.
- R. G. Schoenmakers, P. Van De Wetering, D. L. Elbert and J. A. Hubbell, J. Controlled Release, 2004, 95, 291–300 CrossRef CAS PubMed.
- X. Wang, L. Liu, Y. Luo, H. Shi, J. Li and H. Zhao, Macromol. Biosci., 2012, 12, 1575–1582 CrossRef CAS PubMed.
- M. Oishi, S. Sasaki, Y. Nagasaki and K. Kataoka, Biomacromolecules, 2003, 4, 1426–1432 CrossRef CAS PubMed.
- U. Hasegawa, S. Sawada, T. Shimizu, T. Kishida, E. Otsuji, O. Mazda and K. Akiyoshi, J. Controlled Release, 2009, 140, 312–317 CrossRef CAS PubMed.
- M. Oishi, Y. Nagasaki, K. Itaka, N. Nishiyama and K. Kataoka, J. Am. Chem. Soc., 2005, 127, 1624–1625 CrossRef CAS PubMed.
- K. Kataoka, K. Itaka, N. Nishiyama, Y. Yamasaki, M. Oishi and Y. Nagasaki, Nucleic Acids Symp. Ser., 2005, 49, 17–18 CrossRef PubMed.
- J. H. Jeong, T. G. Park and S. H. Kim, Pharm. Res., 2011, 28, 2072–2085 CrossRef CAS PubMed.
- J. Zou, F. W. Zhang, S. Y. Zhang, S. F. Pollack, M. Elsabahy, J. Fan and K. L. Wooley, Adv. Healthcare Mater., 2014, 3, 441–448 CrossRef CAS PubMed.
- D. Peer, J. M. Karp, S. Hong, O. C. Farokhzad, R. Margalit and R. Langer, Nat. Nanotechnol., 2007, 2, 751–760 CrossRef CAS PubMed.
- Y. Wang, C. Y. Hong and C. Y. Pan, Biomacromolecules, 2012, 13, 2585–2593 CrossRef CAS PubMed.
- M. E. Fox, F. C. Szoka and J. M. J. Frechet, Acc. Chem. Res., 2009, 42, 1141–1151 CrossRef CAS PubMed.
- F. Meng, W. E. Hennink and Z. Zhong, Biomaterials, 2009, 30, 2180–2198 CrossRef CAS PubMed.
- W. Yang, C. Y. Pan, X. Q. Liu and J. Wang, Biomacromolecules, 2011, 12, 1523–1531 CrossRef CAS PubMed.
- Y. Wang, C. Y. Hong and C. Y. Pan, Biomacromolecules, 2013, 14, 144–1451 Search PubMed.
- N. V. Tsarevsky, J. Huang and K. Matyjaszewski, J. Polym. Sci., Part A: Polym. Chem., 2009, 47, 6839–6851 CrossRef CAS.
- W. J. Zhang, W. Fan, M. Li, C. Y. Hong and C. Y. Pan, Acta Chim. Sin., 2012, 70, 1690–1696 CrossRef CAS.
- M. Sun and C. Y. Pan, Sci. China: Chem., 2010, 53, 2440–2451 CrossRef CAS.
- J.-F. Lutz, J. Andrieu, S. Zgün, C. Rudolph and S. Agarwal, Macromolecules, 2007, 40, 8540–8543 CrossRef CAS.
- G. K. Balendiran, R. Dabur and D. Fraser, Cell Biochem. Funct., 2004, 22, 343–352 CrossRef CAS PubMed.
- Y. Lee, H. Koo, G.-W. Jin, H. Mo, M. Y. Cho, J.-Y. Park, J. S. Choi and J. S. Park, Biomacromolecules, 2005, 6, 24–26 CrossRef CAS PubMed.
- L.-L. Xie, W.-J. Tong, J.-Q. Xu and C.-Y. Gao, Chin. J. Polym. Sci., 2012, 30, 719–726 CrossRef CAS.
Footnote |
† Electronic supplementary information (ESI) available: 1H NMR spectra of methacryloyloxy-3-thiohexanoyl–CPT and its intermediates, three hyperbranched star polymers; GPC curves of the hyperbranched polymers and the hyperbranched star polymers; as well as standard curve of CPT. See DOI: 10.1039/c5tb01905b |
|
This journal is © The Royal Society of Chemistry 2016 |
Click here to see how this site uses Cookies. View our privacy policy here.