DOI:
10.1039/C5SC04130A
(Edge Article)
Chem. Sci., 2016,
7, 3427-3431
Construction of spirocarbocycles via gold-catalyzed intramolecular dearomatization of naphthols†
Received
31st October 2015
, Accepted 1st February 2016
First published on 2nd February 2016
Abstract
A highly efficient, gold-catalyzed intramolecular dearomatization reaction of naphthols via 5-endo-dig cyclization is described. This facile and direct approach furnishes spirocarbocycles in excellent yields under mild conditions.
Spirocarbocycles have captured the close attention of organic chemists due to their unique structural characteristics, including fully substituted carbon centers. Moreover, spirocarbocycles often appear in diverse natural products and biologically active molecules (Fig. 1).1 Given the distinctive properties of spirocarbocycles, especially the relatively congested quaternary carbon center, it has been a challenging task for chemists to develop a synthetically applicable methodology for a long time, until recent progress in organometallic catalysis.2 However, highly efficient, mild and streamlined synthetic routes are still in great demand.
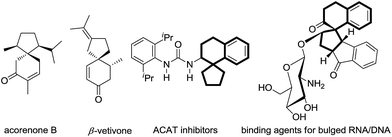 |
| Fig. 1 Examples of natural products and biologically active compounds containing spirocarbocyclic backbones. | |
Meanwhile, gold catalysis has exhibited remarkable capacity for the construction of complex molecules since the new millennium.3 The potent soft Lewis acidity of cationic gold(I) complexes enables, upon coordination, efficient attack on alkynes and alkenes by various nucleophiles, thereby leading to the formation of functional products including spirocarbocycles. Despite remarkable progress, there are relatively few reports on the application of gold catalysis in dearomatization reactions.4,5 Particularly, dearomatization reactions of phenol and derivatives have been much under-developed despite potential direct access to highly functionalized spirocarbocycles.6,7 In this regard, Hamada and coworkers recently reported an elegant gold-catalyzed 5-exo-dig carbocyclization of phenols in the presence of methanesulfonic acid and 2,6-di-tert-butylpyridine.6b Interestingly, we found 5-endo-dig cyclization products could be selectively obtained via gold-catalyzed dearomatizations of naphthols under mild conditions. Herein, we report our findings.
We began our investigation by testing 1-naphthol derivative 1a with commercially available gold complex Ph3PAuCl and various chloride scavengers. The results are summarized in Table 1. To our delight, in the presence of Ph3PAuCl (5 mol%) and NaBARF (tetrakis[3,5-bis(trifluoromethyl)phenyl]boron sodium) (5 mol%), the gold-catalyzed dearomatization reaction of 1a (0.1 mmol) in DCM (1.0 mL) at room temperature proceeded smoothly to afford the desired spirocarbocyclic product 2a in 72% yield (100% yield based on conversion) in 5 h, albeit with incomplete conversion (entry 1, Table 1). However, other chloride scavengers such as AgOTf, AgNTf2, and Cu(OTf)2 (entries 2–4, Table 1) led to little desired product despite nearly complete substrate consumption. In the presence of AgNTf2, the tricycle product 2aa was isolated in 86% yield. Interestingly, AgOMs was found to be the best chloride scavenger, and the reaction proceeded to completion in 5 h, affording 2a in excellent yield (>95% NMR yield, entry 5, Table 1). Next, solvent screening was carried out. Among the solvents evaluated, toluene and THF (entries 6 and 7, Table 1) led to slow reactions while no desired product 2a was detected in MeOH (entry 8, Table 1). As expected, Ph3PAuCl, AgOMs, or HOMs alone could not catalyze this dearomative spirocylization (entries 9–11, Table 1), suggesting that the combination of Ph3PAuCl and AgOMs is essential for this reaction. Ph3PAuOMs is most likely the catalyst. Indeed, when Ph3PAuOMs (entry 12, Table 1) was prepared in pure form and used as the catalyst, the reaction outcome was identical to that when the catalyst was prepared in situ (entry 5). Moreover, the reaction could be conducted open-flask without erosion of the yield (entry 13, Table 1).
Table 1 Optimization of reaction conditionsa
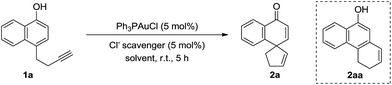
|
Entry |
Cl− scavenger |
Solvent |
Conversionb (%) |
Yieldb (%) |
Reaction conditions: 1a (0.1 mmol), Ph3PAuCl (5 mol%), Cl− scavenger (5 mol%) in 1.0 mL solvent, r.t, 5 h.
Determined by 1H NMR using CH2Br2 (0.1 mmol) as internal standard; isolated yield in parentheses.
2aa was isolated in 86% yield.
2.5 mol% of Cu(OTf)2 was added.
Reaction was performed without Ph3PAuCl.
Reaction was performed with HOMs (5 mol%) instead of Ph3PAuCl.
Reaction was performed with Ph3PAuOMs (5 mol%).
Reaction was performed open-flask.
|
1 |
NaBARF |
DCM |
72 |
72 |
2 |
AgOTf |
DCM |
>95 |
<5 |
3c |
AgNTf2 |
DCM |
>95 |
<5 |
4d |
Cu(OTf)2 |
DCM |
>95 |
<5 |
5 |
AgOMs |
DCM |
>95 |
>95 (96) |
6 |
AgOMs |
Toluene |
42 |
42 |
7 |
AgOMs |
THF |
10 |
10 |
8 |
AgOMs |
MeOH |
<5 |
<5 |
9e |
AgOMs |
DCM |
<5 |
<5 |
10 |
— |
DCM |
<5 |
<5 |
11f |
— |
DCM |
<5 |
<5 |
12g |
— |
DCM |
>95 |
>95 (92) |
13h |
AgOMs |
DCM |
>95 |
>95 (96) |
Under the above optimized reaction conditions, we then explored the substrate scope of this reaction. The results are summarized in Scheme 1. For substrates bearing terminal alkyne pendants, the dearomatization reactions all proceeded well, delivering the corresponding spirocarbocyclic products 2a, 2b and 2c in satisfactory yields (2a, 96% yield; 2b, 99% yield; 2c, 98% yield). Despite the fact that the reaction of a substrate with a 2-methyl group on the naphthyl ring was sluggish (2d, 44% yield, 44% conversion after 34 h), the yield of 2d could be improved to 79% with the addition of 10 mol% catalyst in 2 portions. Next, various substrates bearing internal alkyne tethers were examined. Pleasingly, halogen-substituted alkynes did not interfere with the gold-catalyzed cyclization and the corresponding products (Br, 2e; I, 2f) could be obtained in nearly quantitative yields within 20 minutes. In addition, when phenyl-substituted alkyne substrate 1g was subjected to the reaction conditions, the reaction was completed even faster, delivering spirocarbocyclic product 2g in 95% yield in 10 min. Moreover, different electron-donating groups (2h, MeO; 2i, Me) or electron-withdrawing groups (2j, F; 2k, Cl; 2l, Br; 2m, CN; 2n, CO2Me) on the para-position of the phenyl ring were compatible with this reaction, and the corresponding spirocarbocyclic products were formed in superb yields (97–99%). Likewise, a methyl or methoxyl group at either the ortho- or meta-position on the phenyl ring had little influence on the reaction, and the spirocarbocyclic products 2o and 2p were isolated in 99% and 96% yields, respectively. It is worth mentioning that substrates with other aromatic rings attached to the alkyne moiety also underwent the spirocyclization smoothly, affording spirocyclic products containing 1-naphthyl (2q), 5-indolyl (2r), or 2-thienyl (2s) motifs again in excellent yields (91–98%), and even pyridine-containing substrate 1t was converted into the corresponding spirocarbocyclic product 2t in 99% yield with an additional 1.5 equivalents of HOMs. Additionally, substrate 1u bearing a 3-OMe substituent was also compatible with this reaction, delivering 2u in 86% yield. When the linker was further extended, 5-exo-cyclization product 2v was observed in 99% yield. Simple phenolic substrates 1w and 1x were unreactive under the standard reaction conditions.
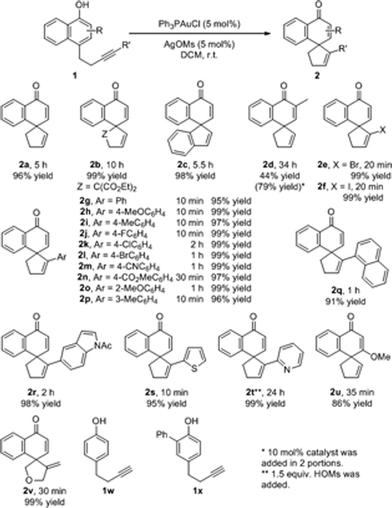 |
| Scheme 1 Substrate scope. Reaction conditions: 1 (0.2 mmol), Ph3PAuCl (5 mol%), AgOMs (5 mol%) in 2.0 mL DCM, r.t. | |
To test the practicality of this new methodology, a gram-scale reaction was conducted. As shown in Scheme 2, only 0.05 mol% of Ph3PAuOMs was required to accomplish the dearomatization reaction to give spirocarbocyclic compound 2g in 99% yield after 2 hours. This level of efficiency in gold catalysis has only been observed in limited reports.8
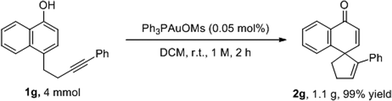 |
| Scheme 2 Gram-scale reaction. | |
To illustrate the synthetic utility of this methodology, transformations of product 2f were conducted. The vinyl iodine moiety readily underwent the Sonogashira coupling reaction with ethynyltrimethylsilane to afford enyne 3f in 99% yield. In addition, 2f smoothly participated in radical reactions.9 Treatment with phenyl vinyl sulfone in the presence of Bu3SnH and AIBN afforded the polycyclic spiro-product 4f with considerable molecular complexity in 55% yield (Scheme 3).
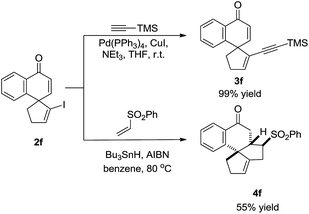 |
| Scheme 3 Transformations of product 2f. | |
A preliminary attempt at the asymmetric reaction revealed that good enantioselectivity (90% ee) could be achieved in the presence of a catalytic amount of (4-CF3C6H4)3PAuCl and chiral silver phosphate ((S)-TRIP-CPA-Ag),10 while moderate enantiocontrol (26% ee) was obtained by using (R)-BINAP(AuCl)2 (Scheme 4).
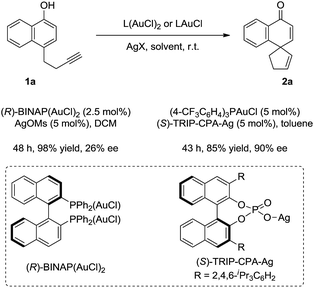 |
| Scheme 4 Preliminary results of the asymmetric reaction. | |
A catalytic cycle is proposed in Scheme 5. The in situ generated cationic gold(I) complex coordinates with and activates the C–C triple bond in 1, and the subsequent 5-endo-dig cyclization is facilitated by the concomitant deprotonation by the counter anion MsO−, directly yielding the spirocyclic gold intermediate A. Alternatively, this cyclization might follow a typical 1,5-enyne cycloisomerization route to afford a cyclopropyl gold carbene intermediate B, which in turn would undergo MsO−-promoted deprotonative fragmentation to arrive at the same intermediate. Although with relatively basic MsO− as the counter anion the direct route is more likely, the intermediacy of B in the step-wise route offers a straightforward rationale for the formation of 2aa, where in the presence of less basic NTf2− the fragmentation of the bold bond of the cyclopropane ring in B is preferred over the depicted deprotonative fragmentation. Protodemetallation of A by the in situ generated MsOH then delivers the desired spirocarbocyclic product 2 while regenerating the active gold catalyst (Scheme 5).
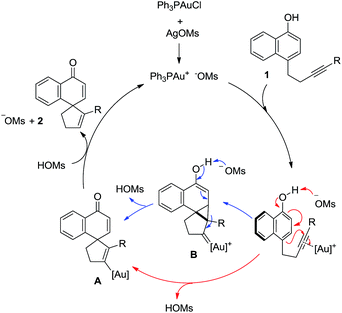 |
| Scheme 5 A proposed catalytic cycle. | |
Conclusions
In conclusion, we have realized a highly efficient and straightforward construction of spirocarbocycles via the gold-catalyzed dearomatization reaction of naphthols under mild reaction conditions. The employment of commercially available catalysts and the compatibility with reaction scale-up and low catalyst loading point to the potential synthetic application of this methodology.
Acknowledgements
This research was supported by the CAS/SAFEA International Partnership Program for Creative Research Teams, the National Basic Research Program of China (973 Program 2015CB856600) and NSFC (21332009, 21421091).
Notes and references
- For selected syntheses and biological studies, see:
(a) W. Oppolzer and K. K. Mahalanabis, Tetrahedron Lett., 1975, 16, 3411–3414 CrossRef;
(b) J. F. Ruppert, M. A. Avery and J. D. White, J. Chem. Soc., Chem. Commun., 1976, 978–978 RSC;
(c) J. A. Marshall and P. C. Johnson, Chem. Commun., 1968, 391–392 RSC;
(d) A. Nakazaki, T. Era, Y. Numada and S. Kobayashi, Tetrahedron, 2006, 62, 6264–6271 CrossRef CAS;
(e) B. K. Trivedi, A. Holmes, T. S. Purchase, A. D. Essenburg, K. L. Hamelehle, B. R. Krause, M. S. Hes and R. L. Stanfield, Bioorg. Med. Chem. Lett., 1995, 5, 2229–2234 CrossRef CAS;
(f) S. Kotha and K. Mandal, Tetrahedron Lett., 2004, 45, 1391–1394 CrossRef CAS;
(g) Z. Xi, G.-S. Hwang, I. H. Goldberg, J. L. Harris, W. T. Pennington, F. S. Fouad, G. Qabaja, J. M. Wright and G. B. Jones, Chem. Biol., 2002, 9, 925–931 CrossRef CAS PubMed;
(h) D. Ouyang, L. Yi, L. Liu, H.-T. Mu and Z. Xi, FEBS J., 2008, 275, 4510–4521 CrossRef CAS PubMed.
-
(a) A. Fürstner, Chem. Soc. Rev., 2009, 38, 3208–3221 RSC;
(b) V. A. D'yakonov, O. A. Trapeznikova, A. de Meijere and U. M. Dzhemilev, Chem. Rev., 2014, 114, 5775–5814 CrossRef PubMed;
(c) L. Fensterbank and M. Malacria, Acc. Chem. Res., 2014, 47, 953–965 CrossRef CAS PubMed.
- For recent reviews on homogenous gold catalysis, see:
(a) A. S. K. Hashmi, Acc. Chem. Res., 2014, 47, 864–876 CrossRef CAS PubMed;
(b) L. Zhang, Acc. Chem. Res., 2014, 47, 877–888 CrossRef CAS PubMed;
(c) Y.-M. Wang, A. D. Lackner and F. D. Toste, Acc. Chem. Res., 2014, 47, 889–901 CrossRef CAS PubMed;
(d) C. Obradors and A. M. Echavarren, Acc. Chem. Res., 2014, 47, 902–912 CrossRef CAS PubMed;
(e) D.-H. Zhang, X.-Y. Tang and M. Shi, Acc. Chem. Res., 2014, 47, 913–924 CrossRef CAS PubMed;
(f) A. Fürstner, Acc. Chem. Res., 2014, 47, 925–938 CrossRef PubMed;
(g) B. Alcaide and P. Almendros, Acc. Chem. Res., 2014, 47, 939–952 CrossRef CAS PubMed;
(h) A. Fürstner, Angew. Chem., Int. Ed., 2014, 53, 8587–8598 CrossRef PubMed;
(i) M. E. Muratore, A. Homs, C. Obradors and A. M. Echavarren, Chem.–Asian J., 2014, 9, 3066–3082 CrossRef CAS PubMed;
(j) C. Obradors and A. M. Echavarren, Chem. Commun., 2014, 50, 16–28 RSC;
(k) J. Xie, C. Pan, A. Abdukader and C. Zhu, Chem. Soc. Rev., 2014, 43, 5245–5256 RSC;
(l) S. Zhang, F. Wei, C. Song, J. Jia and Z. Xu, Chin. J. Chem., 2014, 32, 937–956 CrossRef CAS;
(m) Y. Zhang, T. Luo and Z. Yang, Nat. Prod. Rep., 2014, 31, 489–503 RSC;
(n) P. Gu, Q. Xu and M. Shi, Tetrahedron Lett., 2014, 55, 577–584 CrossRef CAS;
(o) M. Jia and M. Bandini, ACS Catal., 2015, 5, 1638–1652 CrossRef CAS;
(p) R. Dorel and A. M. Echavarren, Chem. Rev., 2015, 115, 9028–9072 CrossRef CAS PubMed;
(q) D. Qian and J. Zhang, Chem. Soc. Rev., 2015, 44, 677–698 RSC;
(r) Y. Wang, M. E. Muratore and A. M. Echavarren, Chem.–Eur. J., 2015, 21, 7332–7339 CrossRef CAS PubMed.
- For reviews on dearomatization reactions, see:
(a) A. R. Pape, K. P. Kaliappan and E. P. Kündig, Chem. Rev., 2000, 100, 2917–2940 CrossRef CAS PubMed;
(b) F. López-Ortiz, M. J. Iglesias, I. Fernández, C. M. Andújar-Sánchez and G. Ruiz-Gómez, Chem. Rev., 2007, 107, 1580–1691 CrossRef PubMed;
(c) C.-X. Zhuo, W. Zhang and S.-L. You, Angew. Chem., Int. Ed., 2012, 51, 12662–12686 CrossRef PubMed;
(d) Q. Ding, X. Zhou and R. Fan, Org. Biomol. Chem., 2014, 12, 4807–4815 RSC;
(e) C.-X. Zhuo, C. Zheng and S.-L. You, Acc. Chem. Res., 2014, 47, 2558–2573 CrossRef CAS PubMed;
(f) W. Zi, Z. Zuo and D. Ma, Acc. Chem. Res., 2015, 48, 702–711 CrossRef CAS PubMed;
(g) S. P. Roche, J.-J. Youte Tendoung and B. Tréguier, Tetrahedron, 2015, 71, 3549–3591 CrossRef CAS. For reviews on dearomatization reactions of phenols, see:
(h) S. P. Roche and J. A. Porco Jr, Angew. Chem., Int. Ed., 2011, 50, 4068–4093 CrossRef CAS PubMed;
(i) L. Pouységu, D. Deffieux and S. Quideau, Tetrahedron, 2010, 66, 2235–2261 CrossRef;
(j) W.-T. Wu, L. Zhang and S.-L. You, Chem. Soc. Rev., 2016 10.1039/C5CS00356C.
- For examples of gold-catalyzed dearomatization reactions of indoles, see:
(a) Y. Liu, W. Xu and X. Wang, Org. Lett., 2010, 12, 1448–1451 CrossRef CAS PubMed;
(b) G. Cera, P. Crispino, M. Monari and M. Bandini, Chem. Commun., 2011, 47, 7803–7805 RSC;
(c) Y.-Q. Zhang, D.-Y. Zhu, Z.-W. Jiao, B.-S. Li, F.-M. Zhang, Y.-Q. Tu and Z. Bi, Org. Lett., 2011, 13, 3458–3461 CrossRef CAS PubMed;
(d) G. Cera, M. Chiarucci, A. Mazzanti, M. Mancinelli and M. Bandini, Org. Lett., 2012, 14, 1350–1353 CrossRef CAS PubMed;
(e) S. G. Modha, A. Kumar, D. D. Vachhani, J. Jacobs, S. K. Sharma, V. S. Parmar, L. Van Meervelt and E. V. Van der Eycken, Angew. Chem., Int. Ed., 2012, 51, 9572–9575 CrossRef CAS PubMed;
(f) J. D. Podoll, Y. Liu, L. Chang, S. Walls, W. Wang and X. Wang, Proc. Natl. Acad. Sci. U. S. A., 2013, 110, 15573–15578 CrossRef CAS PubMed;
(g) M. Jia, M. Monari, Q.-Q. Yang and M. Bandini, Chem. Commun., 2015, 51, 2320–2323 RSC;
(h) W. Zi, H. Wu and F. D. Toste, J. Am. Chem. Soc., 2015, 137, 3225–3228 CrossRef CAS PubMed.
- For examples of gold-catalyzed dearomatization reactions of phenols, see:
(a) M. D. Aparece and P. A. Vadola, Org. Lett., 2014, 16, 6008–6011 CrossRef CAS PubMed;
(b) T. Nemoto, N. Matsuo and Y. Hamada, Adv. Synth. Catal., 2014, 356, 2417–2421 CrossRef CAS;
(c) J. Oka, R. Okamoto, K. Noguchi and K. Tanaka, Org. Lett., 2015, 17, 676–679 CrossRef CAS PubMed.
- For selected
recent examples of other transition-metal-catalyzed dearomatization reactions of phenols, see:
(a) K. Du, P. Guo, Y. Chen, Z. Cao, Z. Wang and W. Tang, Angew. Chem., Int. Ed., 2015, 54, 3033–3037 CrossRef CAS PubMed;
(b) J. Nan, J. Liu, H. Zheng, Z. Zuo, L. Hou, H. Hu, Y. Wang and X. Luan, Angew. Chem., Int. Ed., 2015, 54, 2356–2360 CrossRef CAS PubMed;
(c) S.-G. Wang, Q. Yin, C.-X. Zhuo and S.-L. You, Angew. Chem., Int. Ed., 2015, 54, 647–650 CAS;
(d) D. Yang, L. Wang, F. Han, D. Li, D. Zhao and R. Wang, Angew. Chem., Int. Ed., 2015, 54, 2185–2189 CrossRef CAS PubMed;
(e) S. J. Murray and H. Ibrahim, Chem. Commun., 2015, 51, 2376–2379 RSC;
(f) Q. Yin, S.-G. Wang, X.-W. Liang, D.-W. Gao, J. Zheng and S.-L. You, Chem. Sci., 2015, 6, 4179–4183 RSC;
(g) L. Pantaine, V. Coeffard, X. Moreau and C. Greck, Eur. J. Org. Chem., 2015, 2005–2011 CrossRef CAS;
(h) S. Huang, L. Kötzner, C. K. De and B. List, J. Am. Chem. Soc., 2015, 137, 3446–3449 CrossRef CAS PubMed;
(i) L. Yang, H. Zheng, L. Luo, J. Nan, J. Liu, Y. Wang and X. Luan, J. Am. Chem. Soc., 2015, 137, 4876–4879 CrossRef CAS PubMed;
(j) J. Zheng, S.-B. Wang, C. Zheng and S.-L. You, J. Am. Chem. Soc., 2015, 137, 4880–4883 CrossRef CAS PubMed;
(k) M. J. James, J. D. Cuthbertson, P. O'Brien, R. J. K. Taylor and W. P. Unsworth, Angew. Chem., Int. Ed., 2015, 54, 7640–7643 CrossRef CAS PubMed.
-
(a) X. Giner and C. Nájera, Org. Lett., 2008, 10, 2919–2922 CrossRef CAS PubMed;
(b) N. Marion, R. S. Ramón and S. P. Nolan, J. Am. Chem. Soc., 2009, 131, 448–449 CrossRef CAS PubMed;
(c) X. Giner, C. Nájera, G. Kovács, A. Lledós and G. Ujaque, Adv. Synth. Catal., 2011, 353, 3451–3466 CrossRef CAS;
(d) M. C. Blanco Jaimes, C. R. N. Böhling, J. M. Serrano-Becerra and A. S. K. Hashmi, Angew. Chem., Int. Ed., 2013, 52, 7963–7966 CrossRef CAS PubMed;
(e) V. Lavallo, J. H. Wright II, F. S. Tham and S. Quinlivan, Angew. Chem., Int. Ed., 2013, 52, 3172–3176 CrossRef CAS PubMed;
(f) M. Kumar, G. B. Hammond and B. Xu, Org. Lett., 2014, 16, 3452–3455 CrossRef CAS PubMed;
(g) J. Han, N. Shimizu, Z. Lu, H. Amii, G. B. Hammond and B. Xu, Org. Lett., 2014, 16, 3500–3503 CrossRef CAS PubMed;
(h) J. Ma, H. Jiang and S. Zhu, Org. Lett., 2014, 16, 4472–4475 CrossRef CAS PubMed;
(i) D. Malhotra, M. S. Mashuta, G. B. Hammond and B. Xu, Angew. Chem., Int. Ed., 2014, 53, 4456–4459 CrossRef CAS PubMed;
(j) Y. Xi, D. Wang, X. Ye, N. G. Akhmedov, J. L. Petersen and X. Shi, Org. Lett., 2014, 16, 306–309 CrossRef CAS PubMed;
(k) Y. Wang, Z. Wang, Y. Li, G. Wu, Z. Cao and L. Zhang, Nat. Commun., 2014, 5, 3470, DOI:10.1038/ncomms4470;
(l) M. C. Blanco Jaimes, F. Rominger, M. M. Pereira, R. M. B. Carrilho, S. A. C. Carabineiro and A. S. K. Hashmi, Chem. Commun., 2014, 50, 4937–4940 RSC;
(m) R. M. P. Veenboer, S. Dupuy and S. P. Nolan, ACS Catal., 2015, 5, 1330–1334 CrossRef CAS;
(n) S. M. Inamdar and N. T. Patil, Org. Chem. Front., 2015, 2, 995–998 RSC.
- For an intermolecular example, see: K.-M. Liu, C.-M. Chau and C.-K. Sha, Chem. Commun., 2008, 91–93 RSC.
- For other examples of the use of chiral silver phosphates in gold catalysis, see:
(a) G. L. Hamilton, E. J. Kang, M. Mba and F. D. Toste, Science, 2007, 317, 496–499 CrossRef CAS PubMed;
(b) K. Aikawa, M. Kojima and K. Mikami, Adv. Synth. Catal., 2010, 352, 3131–3135 CrossRef CAS;
(c) R. L. Lalonde, Z. J. Wang, M. Mba, A. D. Lackner and F. D. Toste, Angew. Chem., Int. Ed., 2010, 49, 598–601 CrossRef CAS PubMed;
(d) R. Quach, D. P. Furkert and M. A. Brimble, Tetrahedron Lett., 2013, 54, 5865–5868 CrossRef CAS;
(e) S. Handa, D. J. Lippincott, D. H. Aue and B. H. Lipshutz, Angew. Chem., Int. Ed., 2014, 53, 10658–10662 CrossRef CAS PubMed;
(f) W. Zi and F. D. Toste, Angew. Chem., Int. Ed., 2015, 54, 14447–14451 CrossRef CAS PubMed.
Footnote |
† Electronic supplementary information (ESI) available. See DOI: 10.1039/c5sc04130a |
|
This journal is © The Royal Society of Chemistry 2016 |
Click here to see how this site uses Cookies. View our privacy policy here.