DOI:
10.1039/C6RA24509A
(Paper)
RSC Adv., 2016,
6, 114086-114095
In vitro evaluation of an yttria-stabilized zirconia reinforced nano-hydroxyapatite/polyamide 66 ternary biomaterial: biomechanics, biocompatibility and bioactivity
Received
2nd October 2016
, Accepted 1st December 2016
First published on 2nd December 2016
Abstract
Nano-hydroxyapatite/polyamide 66 (nHA/PA66) is a bioactive composite that is widely applied in orthopedics. However, imperfect mechanical behavior is the main drawback of the nHA/PA66 composite, and this is a limitation for further applications. Meanwhile, zirconia has been attracting increasing attention in recent years, owing to its excellent mechanical behavior and biocompatibility. A novel ternary biomaterial composed of nHA/PA66/yttria-stabilized tetragonal zirconia (YTZ) was prepared in the present research. The material was analyzed by X-ray diffraction and Fourier transform infrared spectrometry; mechanical behavior was also tested. The results of the mechanical-behavior testing showed that the mechanical behaviors of nHA/PA66/YTZ were significantly better than those of nHA/PA66. Besides, the cross-section of this biocomposite was characterized by scanning electron microscopy. Furthermore, the biocompatibility and bioactivity were evaluated in vitro using MC3T3-E1 cells. From the results of the biocompatibility and bioactivity experiments (cell counting kit-8 tests, cell immunofluorescence analysis, alizarin red S staining and western blotting), it was observed that nHA/PA66/YTZ could facilitate the biological function of MC3T3-E1 cells. The results showed that the attachment, proliferation, spreading, calcium nodule formation, and expression of osteogenic-related proteins (collagen 1, osteopontin, osteocalcin) of cells cultured with the nHA/PA66/YTZ composite were better than for control groups. The ternary biomaterial has promising mechanical properties, biocompatibility and bioactivity. Thus, it deserves more attention and is worthy of further research.
Introduction
Biocompatibility, osteoconductivity, and osseointegration are the key elements for biomaterials applied in orthopedics.1,2 Nowadays, biomaterials with biocompatibility and bioactivity attract tremendous fascination from researchers. Consequently, a variety of biomaterials have been successfully used in clinics. In the fields of bone tissue substitution and repair, biomaterials composed of a biologically active inorganic compound (such as hydroxyapatite; HA) and a macromolecular compound can provide both bioactivity and ductility.3–5 Hence, it has been demonstrated that the choice of bioactive element (including inorganic component and polymer) is paramount to devise a usable inorganic/organic biocomposite with favorable mechanical properties, biocompatibility and bioactivity. The outstanding biocompatibility, osteoconductivity, and bioactivity of nHA/PA66, owing to its composition, structure, and mechanical behaviors, give it an extremely good resemblance to natural bone.4 Moreover, a large number of studies have demonstrated that as a bioactive nondegradable material nHA/PA66 not only can achieve the requirements for simulating bone, but also has parallel mechanical behaviors compared with human bone.6,7 Accordingly, this biomaterial has been widely applied for bone-repair engineering, and many kinds of derivative products (such as cage and bone filling material) made from nHA/PA66 have exhibited a good applied value as well.8–11 In addition, it is worth noting that for several clinical applications it was confirmed that nHA/PA66 manifested its bioactivity by directly integrating with host bone and did not appear to have adverse effects after the long-term implantation.12,13 Meanwhile, some other implants, such as metal, may cause adverse reactions (stress shielding or metal allergy) in the human body.14,15
Nonetheless, for fixation surgeries, metal fixation devices are still the first choice owing to their eximious mechanical properties. Unfortunately, the high elastic modulus of metal devices is their fatal shortcoming. It is well known that the high elastic modulus caused by metal fixation devices can induce stress shielding, which can result in bone atrophy and osteopenia.16,17 It is worth noting that the adverse effects on the bone under the metal fixation devices are more serious, and even refractures may occur. Therefore, it is necessary to explore new materials with both favorable mechanical behaviors and bioactivity. The successes of the application of nHA/PA66 incited us to expand its scope of application to internal fixation. However, the nHA/PA66 composite has insufficient mechanical behaviors, which means it is not suitable for use in clinical fixation. In view of these imperfections, it was necessary to develop a new type of biomaterial that had the characteristics of good biomechanics, biocompatibility, and biological activity.
Recently, in order to reinforce the mechanical behaviors of biomaterials, some fibers (such as glass fiber, carbon and polylactic acid) have been used to fortify the mechanical properties of biomaterials.18–20 Simultaneously, an inorganic material, zirconia, has gained increasing attention due to its good mechanical properties and low toxicity. In a plentiful number of experiments, it has been reported that zirconia has osteoconductive properties.21–24 Also, compared with titanium, zirconia will not cause inflammation. In addition, yttria-stabilized tetragonal zirconia (YTZ) has more superior characteristics compared to other ceramics, including high fracture toughness and flexural strength, and has been widely used in many clinical fields, such as hip prostheses, tooth crowns, dental implants.25,26 Some researchers have suggested that YTZ is expected to become the new bone-repair material.27 Thus, YTZ seems to be a satisfactory additive material owing to its high biocompatibility and excellent mechanical properties. However, research about YTZ-reinforced composite is rare. In addition, the studies of YTZ-reinforced composite are one-sided in the research, especially in orthopedic research. It was found that the research studies only involved binary composite and the functional assessment was not all-inclusive.28–30
Thus, with the aim of reinforcing the mechanical behaviors of nHA/PA66 composite and comprehensively evaluating the composite-related functional indexes, we made a ternary composite through incorporation of YTZ into nHA/PA66 for the first time. Subsequently, we investigated the biomechanical behaviors and the interface of the nHA/PA66/YTZ composite. Meanwhile, MC3T3-E1 cells were used to test the biocompatibility and bioactivity of the compound of nHA/PA66 and YTZ.
Materials and methods
Preparation of nHA/YTZ
The preparation method for nHA has been described before in the published literature,31 and the particle size is from 80 nm to 100 nm. Yttria-stabilized zirconia powder, which contained 3% Y2O3 had a particle size from 70 nm to 100 nm was purchased from Tosoh (Tokyo, Japan). Firstly, the powders of the two materials were stirred and mixed at different mixing ratios. nHA and YTZ are ground and mixed by Planetary Ball Mill (PM100; Retsch, Düsseldorf, Germany). The mixing ratios used for nHA and YTZ were 100
:
0, 90
:
10, 80
:
20 and 60
:
40. Secondly, the mixture was compressed in a crucible and sintered for 5 h at 1500 °C to obtain the nHA/YTZ compound.
Preparation of nHA/PA66/YTZ composite
PA66 was obtained from Sichuan National Nanotechnology Co, Ltd (Chengdu, China). The molecular weight of PA66 is about 30
000, and relative viscosity number is 3.2. The nHA/PA66/YTZ composite was prepared by following three steps, comprising compounding, injection, and molding. PA66 grains were mixed with nHA/YTZ and the weight ratio used for nHA/YTZ and PA66 was 3
:
7. PA66/nHA/YTZ was molded in an injection molding machine (BL 160/120C; Shuangma, Zhejiang, China) with a processing temperature ranging from 240 °C to 290 °C (heating temperature of injection molding process is divided into five interval sections, i.e. the first section of 240 °C, the second section of 260 °C, the third section of 265 °C, the fourth section of 272 °C and the fifth section of 290 °C). The nHA/PA66/YTZ composite was prepared into different specification to be used for subsequent experiments.
In consideration that nHA/PA66 is known to be biocompatible with MC3T3-E1 cells and has been widely applied in clinics, in this study, we used nHA/PA66 as the control. The nHA/PA66 was also provided by Sichuan National Nanotechnology Co, Ltd. The nHA/PA66/YTZ and nHA/PA66 composites were cut into different sizes for investigation of their biocompatibility and bioactivity.
Characterization of nHA/PA66/YTZ composite
To observe the surface morphology of nHA, YTZ and nHA/YTZ, scanning electron microscopy (SEM; JSM-7500; JEOL Ltd, Tokyo, Japan) was employed. The nHA/YTZ distribution in the PA66 matrix was also detected by SEM. X-ray diffraction (XRD; X'Pert pro-MPD; Philips, Eindhoven, The Netherlands) and Fourier transform infrared spectroscopy (FTIR; 170SX; Nicolet, Madison, WI, USA) were carried out to identify the phase composition and structure of the nHA/PA66/YTZ composite. In addition, the mechanical behaviors of nHA/PA66/YTZ were detected by using a mechanical testing machine (REGER 30–50; Shenzhen Reger Co, Ltd, Shenzhen, China). The experimental conditions for the mechanical tests: three types of testing methods of mechanical testing are as follows respectively. Compression test: sample size: 10 × 10 × 4 mm, pressing speed: 5 mm min−1, compression height ratio: 50%; bending test: sample size: 10 × 4 × 80 mm, bending span: 60 mm, pressing speed: 5 mm min−1, winding degree of bending: 15 mm; tensile test: sample size: 10 × 4 × 100 mm, gauge length: 60 mm, tensile speed: 5 mm min−1.
Cell culture
MC3T3-E1 cells, which are preosteoblasts, were used in this research. MC3T3-E1 cells were purchased from the American Type Culture Collection (Manassas, VA, USA) and Dulbecco's modified Eagle's medium/F12 (DMEM/F12; Hyclone, Logan, UT, USA) with 10% fetal bovine serum was used for cell culture. MC3T3-E1 cells at passage 3–4 were used.
Cytotoxicity assays
DMEM/F12 containing 10% FBS was used as the extraction medium. In this experiment, the extract solution of nHA/PA66 was used for comparison and the DMEM/F12 as a blank control. A cell counting kit-8 (CCK-8; Beyotime, Jiangsu, China) was employed to investigate cell viability. Two hundred microliters of cell suspension were seeded in each well of a 96-well plate. The cell density of each well was 2 × 104 viable cells per cm2. At 1, 4 and 7 days, 20 μl CCK-8 solution was added to each well and the plates were then incubated for 1 h. The plates were then read (sample absorbance) at 450 nm using a Thermo Scientific microplate reader (Thermo Fisher Scientific Co Ltd, Waltham, MA, USA).
Cell attachment and spreading
The nHA/PA66/YTZ and nHA/PA66 scaffolds were cut into 1 × 1 × 0.1 cm samples for this experiment. Cells were co-cultured with different samples in a 24-well plate. The cell density of each well was 2 × 104 viable cells per cm2. The attachment and morphology of MC3T3-E1 cells were observed by SEM. Firstly, after co-culturing with different samples for 24 hours, the cells on the samples were carefully washed with PBS three times. Secondly, the cells were fixed with 2.5% glutaraldehyde for 15 min. Thirdly, the cells were dehydrated in a series of ethanol concentrations from low to high (30%, 50%, 75%, 80%, 90%, 100%, and 100% for 15 minutes each time). Finally, all the samples were dried by desiccant, sputter-coated with gold and observed by SEM.
Cell spreading was investigated by observing the cell microfilaments of the MC3T3-E1 cells. To begin with, after co-culturing with different samples for 24 h, the cells were carefully washed with PBS three times. The cells on the samples were fixed with 3.7% paraformaldehyde for 15 min at room temperature. After this, 0.1% Triton X-100 was used to permeabilize the cells for 5 min. The cells were washed with PBS again and stained with rhodamine-phalloidin (50 μg ml−1; Sigma-Aldrich, St. Louis, MO, USA) at room temperature for 30 min. Finally, the cells on the samples were washed with PBS three times once again and stained with 4,6-diamidino-2-phenylindole (0.1 μg ml−1; Sigma-Aldrich, St. Louis, MO, USA) for 15 min. The cell microfilament cytoskeleton and nucleus were observed by confocal laser scanning microscopy using a LSM800 confocal Laser microscopy (Carl Zeiss, Jena, Germany).
Cell culture of inductive osteogenesis
Different sized samples were placed into 6-well (for Φ 32 mm samples) or 24-well (for 1 × 1 × 0.1 cm samples) plates for for western blotting and alizarin red staining. The cell density of each well was 3 × 104 viable cells per cm2. After co-culturing with different samples for 24 h, the culture medium (DMEM/F12) was changed to osteogenic induction medium, which was purchased from Cyagen Biosciences Co, Ltd (Guangzhou, China). The osteogenic induction medium was replaced every 3 days.
Alizarin red S staining
Alizarin red staining was employed for detecting calcium nodules formation. The samples, sized of 1 × 1 × 0.1 cm, were placed into a 24-well plate and co-cultured with MC3T3-E1 cells. After osteogenic induction for 21 days, the samples were harvested for alizarin red staining. Firstly, the cells in each well were carefully washed with PBS two times and fixed with 4% paraformaldehyde for 30 minutes. Secondly, after washing with PBS three times, alizarin red S (1%; Cyagen, Guangzhou, China) solution was added in each well and incubated for 5 minutes at room temperature. The staining results were observed by light microscope (BX51; Olympus, Tokyo, Japan). Meanwhile, for the quantitative analysis of calcium nodules on the composite, cetylpyridinium chloride (10%; Sigma-Aldrich, St. Louis, MO, USA) solution was used to desorb the stain on the samples. After that, the dissolving solution for each sample was collected and the absorbance was read at 620 nm using a Thermo Scientific microplate reader.
Western blot analysis
The MC3T3-E1 cells were seeded at a density of 3 × 104 viable cells per cm2 in a 6-well plate and co-cultured with nHA /PA66 or nHA/PA66/YTZ discs (Φ 32 mm). After incubation for 14, 21, and 28 days, the MC3T3-E1 cells were washed with cold PBS and lysed with RIPA lysis buffer (Beyotime, Jiangsu, China) to prepare the cell lysates. The bicinchoninic acid method (Beyotime, Jiangsu, China) was employed to assay the total protein concentration of whole cell lysates. Forty micrograms of each protein sample was loaded onto sodium dodecyl sulfate-polyacrylamide gel electrophoresis (SDS-PAGE) and transferred to polyvinylidene fluoride (PVDF) membranes. PVDF membranes were blocked with block solution (Beyotime, Jiangsu, China) for 1 h. Subsequently, the membranes were incubated overnight with primary antibodies against collagen 1 (COL-1), osteopontin (OP), osteocalcin (OC) and β-actin at a dilution of 1
:
1000. After these steps, membranes were incubated with anti-rabbit IgG secondary antibodies (Boster, WuHan, China). A chemiluminescence system was employed to detect bound antibodies.
Statistical analysis
SPSS 21.0 (SPSS Inc, Chicago, IL, USA) was employed for statistical analysis. Quantitative data were represented as means ± standard deviation (SD). One-way analysis of variance was performed. Statistically differences was determined at P < 0.05.
Results
SEM observation
Fig. 1 shows the surface morphology of nHA, YTZ and nHA/YTZ. It shows that the particles of YTZ fill in the pores among the particles of HA. It is indicative that YTZ and HA are a good combination. Fig. 1 also presents an SEM image of a cross-section of the nHA/PA66/YTZ composite. The nHA/YTZ particles were found to be homogeneously distributed on the composite's surface. It is indicative that nHA/YTZ particles remained the nanostructures in the composite and there was no obvious agglomeration of nHA/YTZ particles in the process of synthesis.
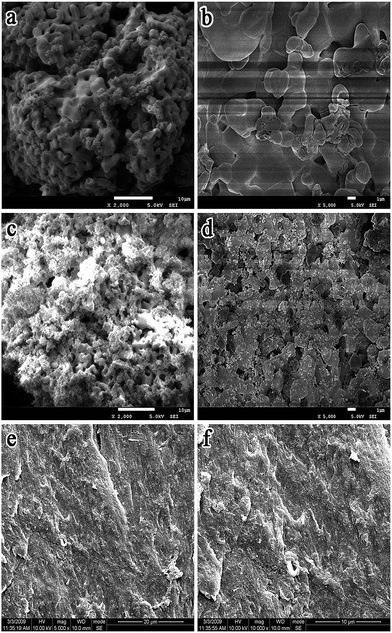 |
| Fig. 1 SEM images of nHA (a and b) and nHA/YTZ (c and d) and cross-section morphology of nHA/PA66/YTZ (e and f). The nHA/YTZ weight ratio was 60 : 40. | |
XRD and FTIR analysis
The XRD results for YTZ, nHA, nHA/YTZ, nHA/PA66 and nHA/PA66/YTZ are shown in Fig. 2. In Fig. 2(a), the peaks at 2Θ = 30.23°, 35.15° and 50.27° belong to YTZ. In Fig. 2(b), the peaks at 2Θ = 25.87°, 31.79° and 32.19° belong to nHA. Fig. 2(d) shows XRD profile of the nHA/PA66 and the peaks at 2Θ = 20.39° and 22.71° are the characteristic peaks of PA66. In Fig. 2(c) and (e), all the characteristic peaks of those materials were present in the composite. It was concluded that this composite was synthesized from nHA, PA66 and YTZ.
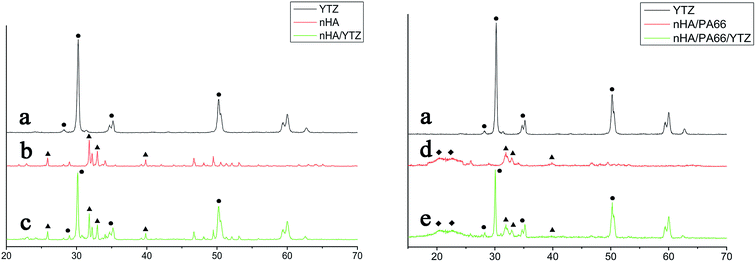 |
| Fig. 2 XRD profiles of YTZ (a), nHA (b), nHA/YTZ (c), nHA /PA66 (d) and 40% YTZ/nHA/PA66 (e). (●) YTZ, (▲) nHA, (◆) PA66. | |
Fig. 3 shows the FTIR spectrum of YTZ, nHA/PA66 and nHA/PA66/YTZ. The stretching vibration of nitrogen–hydrogen (NH) was found at 3303 cm−1, and the carbon hydrogen (CH2) vibrational peaks were found at approximately 2937 cm−1 and 2867 cm−1. The stretching vibrational peak at 1540 cm−1 was attributed to the carbon–nitrogen (CN) and the stretching vibrational peak at 1640 cm−1 was carbonyl (C
O). It was observed that nHA/PA66/YTZ has all of the characteristic stretching vibrational peaks of nHA/PA66. What is more, compared to nHA/PA66, in nHA/PA66/YTZ no new peaks nor peaks shifts were found, which is indicative that there was no effect on the composition of nHA/PA66 with the addition of YTZ.
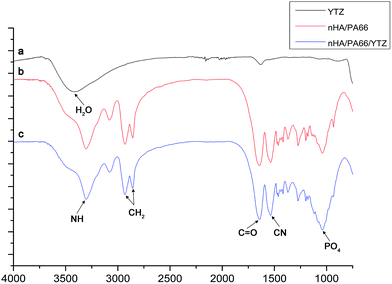 |
| Fig. 3 FTIR spectra of YTZ (a), nHA/PA66 (b) and 40% YTZ/nHA/PA66 (c). | |
Testing of mechanical behaviors of the composite
Table 1 lists the mechanical behaviors of human cortical bone, nHA/PA66 and nHA/PA66/YTZ. The compressive strength, tensile strength, bending strength, breaking elongation and elastic modulus of the composite were measured. It was observed that for human cortical bone, nHA/PA66, 10% YTZ/nHA/PA66, 20% YTZ/nHA/PA66 and 40% YTZ/nHA/PA66: the compressive strengths were 141.7 ± 5.43, 97.03 ± 4.94 MPa, 126.14 ± 6.42 MPa, 141.41 ± 10.35 MPa and 154.15 ± 12.20 MPa, respectively; the tensile strengths were 56.52 ± 3.81, 60.69 ± 2.04 MPa, 78.90 ± 2.652 MPa, 111.41 ± 2.7 MPa and 122.56 ± 2.95 MPa, respectively; the bending strengths were 117.71 ± 4.10, 72.8 ± 2.06 MPa, 94.64 ± 2.678 MPa, 113.68 ± 2.616 MPa and 119.23 ± 1.77 MPa, respectively; the breaking elongation values were 8.13 ± 0.36%, 5.28 ± 0.27%, 6.864 ± 0.351%, 10.66 ± 0.247% and 10.71 ± 0.224%, respectively; the elastic modulus values were 5.45 ± 0.21, 1.69 ± 0.37 GPa, 2.197 ± 0.481 GPa, 2.62 ± 0.51 GPa and 3.60 ± 0.237 GPa, respectively. The results showed that the mechanical behaviors of this ternary composite were improved by the addition of YTZ. Moreover, it was suggestive that the higher YTZ content, the better the mechanical strength of nHA/PA66/YTZ composite. Our results showed that 40% YTZ/nHA/PA66 exhibited the most preferable mechanical properties. Thus, 40% YTZ/nHA/PA66 was chosen for the further experiments.
Table 1 Mechanical properties of human cortical bone, nHA/PA66 and nHA/PA66/YTZ with different YTZ contents. The data are expressed as means ± standard deviation
Samples |
Compressive strength (MPa) |
Tensile strength (MPa) |
Bending strength (MPa) |
Breaking elongation (%) |
Tensile modulus (GPa) |
Human cortical bone |
141.7 ± 5.43 |
56.52 ± 3.81 |
117.71 ± 4.10 |
8.13 ± 0.36 |
5.45 ± 0.21 |
nHA/PA66 |
97.03 ± 4.94 |
60.69 ± 2.04 |
72.8 ± 2.06 |
5.28 ± 0.27 |
1.69 ± 0.37 |
10% YTZ/nHA/PA66 |
126.14 ± 6.42 |
78.90 ± 2.652 |
94.64 ± 2.678 |
6.864 ± 0.351 |
2.197 ± 0.481 |
20% YTZ/nHA/PA66 |
141.41 ± 10.35 |
111.41 ± 2.7 |
113.68 ± 2.616 |
10.66 ± 0.247 |
2.62 ± 0.51 |
40% YTZ/nHA/PA66 |
154.15 ± 12.20 |
122.56 ± 2.95 |
119.23 ± 1.77 |
10.71 ± 0.224 |
3.60 ± 0.237 |
Cytotoxicity assays
Through the CCK-8 assay, it was found that the number of MC3T3-E1 cells increased with the increasing incubation time for each group. As shown in Fig. 4, according to the statistical analyses, the cells in the experimental group exhibited a similar proliferation capacity compared to other groups. There was no significant difference between the experimental group and other groups at each observation time point. The results of the CCK-8 experiment are indicative that nHA/PA66/YTZ had no cytotoxicity, suggesting that the nHA/PA66/YTZ had favorable cell affinity and excellent biocompatibility.
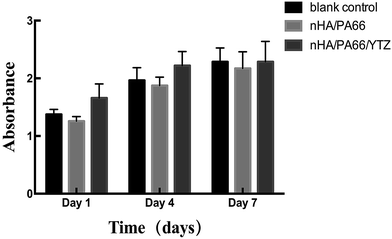 |
| Fig. 4 CCK-8 assays for proliferation of MC3T3-E1 cells co-cultured with DMEM/F12 (blank control), extracts of nHA/PA66 composite and extracts of nHA/PA66/YTZ composite for 1, 4, and 7 days. | |
Cell attachment and spreading
Fig. 5 presents the SEM graphs of MC3T3-E1 cells co-cultured with different discs after 24 h. It was observed that the spreading of MC3T3-E1 cells on the nHA/PA66/YTZ composite surface was better than for other groups. We saw that the MC3T3-E1 cells on the nHA/PA66/YTZ composite surface were spindle-shaped, and numerous pseudopods were produced to stick onto the composite's surface. Compared with nHA/PA66/YTZ, the cell spreading on nHA/PA66 appeared less efficient and the number of attached cells was fewer.
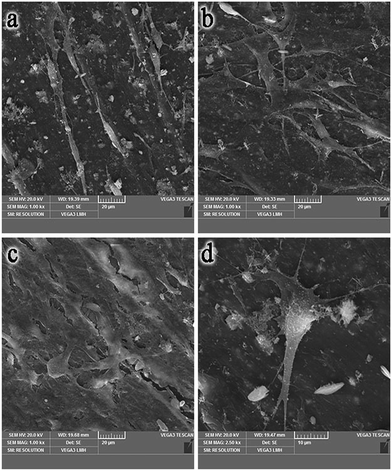 |
| Fig. 5 SEM images of M3C3T3-E1 cells on the surface of a 24-well cell culture plate (a), nHA/PA66 (b) and nHA/PA66/YTZ (c and d). | |
Fig. 6 reveals the cell microfilaments of MC3T3-E1 cells co-cultured on different samples. The images of cell immunofluorescence were observed by confocal laser scanning microscopy. As shown in the graph, the cells co-cultured with nHA/PA66/YTZ and nHA/PA66 composites exhibited a long and spindle-shaped morphology; whereas, the cells in the blank control group (6-well plate without biomaterials) exhibited a polygon shape. Moreover, a confluent, clustering and multilayer morphology was apparent in the cells cultured on the nHA/PA66/YTZ composite, and cells were linked to adjacent cells with more actin filaments; whereas a monolayer and dispersive morphology, with fewer actin filaments, was exhibited in the blank control and nHA/PA66 groups. The quantity of cells in the nHA/PA66/YTZ group was larger than for the other groups, which demonstrated that the nHA/PA66/YTZ composite had better biocompatibility and cell adhesion induction properties than the other groups.
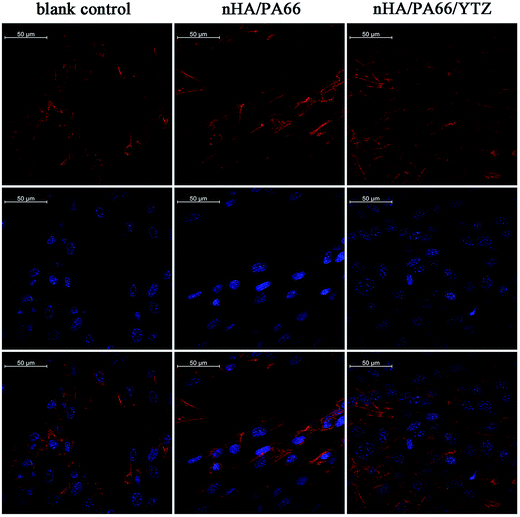 |
| Fig. 6 The spreading of MC3T3-E1 cells on the surface of a 24-well cell plate (blank control), nHA/PA66 and nHA/PA66/YTZ after 24 h. | |
Alizarin-red S staining
Fig. 7 shows the alizarin red staining results for the MC3T3-E1 cells co-cultured with different composites. After 21 days of osteogenic induction, calcium nodule formation was visualized by alizarin red S staining. The result of the staining show that there was more calcium nodule formation on nHA/PA66/YTZ composite than for the other control groups. Also, the quantitative analysis showed that the calcium nodule formation on the nHA/PA66/YTZ composite was much higher than for the other control groups, the differences were statistically significant.
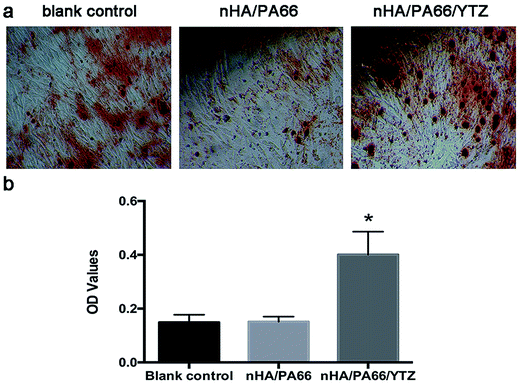 |
| Fig. 7 Optical microscopy images of alizarin red S staining (a) and quantitative analysis of calcium nodules on the composite (b). *Significant difference compared with blank control and nHA/PA66 groups. | |
Expression of osteogenic differentiation-related protein
To investigate the influence of the nHA/PA66/YTZ composite on osteoblast differentiation of MC3T3-E1 cells, western blotting was employed for detecting COL-1, OP and OC, which are indicators of osteogenic differentiation. Fig. 8 shows the expression of the osteogenic differentiation-related protein of MC3T3-E1 cells co-cultured with different samples. According to the protein expression levels of these osteogenic indicators, it was found that nHA/PA66/YTZ had no adverse affect on the osteogenic differentiation of MC3T3-E1 cells. Meanwhile, it was obvious that the expression level of these osteogenic indicators was higher in the nHA/PA66/YTZ group at each time point. It is indicative that the nHA/PA66/YTZ composite exhibited better bioactivity than the nHA/PA66 and blank control groups.
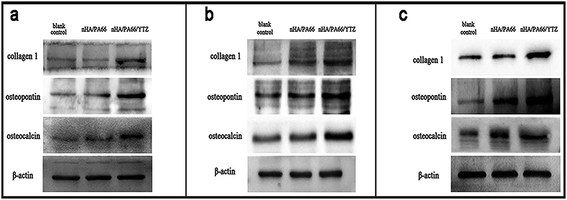 |
| Fig. 8 The expression of osteogenic differentiation-related proteins (collagen 1, osteopontin, osteocalcin) of MC3T3-E1 cells co-cultured with different composites in osteogenic inductive medium after 14 (a), 21 (b), and 28 (c) days. | |
Discussion
Bone is composed by organic and inorganic components, the same as biocomposite.32 In bone structure, collagen I and HA, respectively, play the role of organic and inorganic components.33 Therefore, in recent decades, related research about HA/polymer composites has been gaining more and more attentions due to the composite's biomimetic mechanism. Simultaneously, some research has focused on expanding the applications of HA/polymer composite to produce internal fixation devices, but these research results were not ideal.1,5,7,32,33 It was concluded that the experiments failed owing to the composite not having sufficient mechanical properties. On the contrary, because of their excellent mechanical properties and toughness, metal materials still can be considered the preferred choice for internal fixation devices. Nevertheless, the advantages and disadvantages of metal devices are equally obvious.14,34 Firstly, because of the high modulus, metal fixation can lead to stress shielding, and stress shielding is known to play a negative role in the bone healing process. Thus, it is necessary for internal metal fixation devices to be removed by a second operation. Secondly, metal devices are not ideal biomaterials due to their non-bioactive. Last, but not least, allergic responses caused by metal ion are not rare.15,35 Consequently, research and development of both the bioactivity and biomechanical behaviors of biocomposites are particularly imperative.
In the present study, to gain improved mechanical and biological properties, YTZ was added into nHA/PA66 through the above mentioned method to reinforce the nHA/PA66 composite. nHA and YTZ were first sintered at high temperature and the morphology of the nHA/YTZ composite was examined using SEM. Furthermore, a bioactive composite, nHA/PA66/YTZ, was prepared by using a procedure of mixing, injection and molding. Through the SEM observation, it was found that the particles of YTZ fill in the pores among the particles of HA, and the nHA/YTZ particles were uniformly distributed on the PA66 matrix. In Fig. 2(a), the characteristic peak of YTZ (ICDC: 49-1642), can be seen at the position of 2Θ = 30.23° (111), 35.15° (200) and 50.27° (220). From the refinement of XRD results, the YTZ crystalline of YTZ and nHA can be listed in detail. The YTZ crystal structure is tetragonal. The lattice constants are α = β = γ = 90°, a = b = 0.3754 nm, c = 0.66127 nm, and unit cell dimension is 134.65 Å3. As in Fig. 2(b), in the hydroxyapatite with high temperature sintering, the characteristic peaks of nHA (ICDC: 09-0432) can be observed at 2Θ = 25.87° (002), 31.79° (300) and 32.19° (211) respectively. nHA crystal structure is hexagon type. The lattice constants are α = β = 90°, γ = 120°, a = b = 0.94228 nm, c = 0.68871 nm, and unit cell dimension is 529.13 Å3. With sintered composite of nHA/YTZ, YTZ is changed into cubic structure. The lattice constants are α = β = γ = 90°, a = b = c = 0.54898 nm, and unit cell dimension is 136.69 Å3. nHA is still in hexagon structure, α = β = 90°, γ = 120°, a = b = 0.92584 nm, c = 0.63408 nm, and unit cell dimension is 536.17 Å3. From the above discussed results, after sintering, YTZ crystalline structure could be changed obviously. Meanwhile, nHA remain its hexagon crystalline structure except for the decreasing of dimension, which indicated that the crystallinity of nHA was increased after sintering. In Fig. 2(d), two kinds of crystal forms of PA66 can be seen at 2Θ = 20.39° and 22.71, i.e. α-crystal form and β-crystal form. Meanwhile, as in Fig. 2(c) and (f), we can see that above mentioned all characteristic peaks are seen in nHA/PA66 and nHA/PA66/YTZ composite. This was indicative that the composite was composed of nHA, PA66 and YTZ. Moreover, in the FTIR all of the peaks of nHA/PA66 were also found in nHA/PA66/YTZ. There were no new peaks in nHA/PA66/YTZ compared to nHA/PA66, demonstrating that the addition of YTZ into nHA/PA had no obvious effect on the composition of nHA/PA66. After the incorporation of YTZ into nHA/PA66, it was found that the mechanical behaviors (compressive strength, tensile strength, bending strength, breaking elongation and elastic modulus) of the nHA/PA66/YTZ composite were superior to nHA/PA66. Compared with nHA/PA66, the composite system represented a compressive strength increased by 30–88%, a tensile strength increased by 30–101% and a bending strength increased by 29–63%, with breaking elongation increased by 30–102% and a modulus increased by 29–112%. Meanwhile, it was obvious that the mechanical behaviors were enhanced according to the increased content of YTZ. Moreover, the compressive strength, tensile strength and bending strength of nHA/PA66/YTZ (YTZ
:
nHA 4
:
6) were higher than those of human bone, while its modulus was close to that of human bone. On mechanical performance selection of orthopaedic biomaterial, it is hoped that the mechanical performance of biomaterials is near or higher than the normal mechanical strength of human bone. Meanwhile, it has smaller elastic modulus, so as to reduce stress shielding after implanting biomaterials in human body and reduce the condition of nonunion condition of bone.36–38 Thus, the ternary composite could be used in orthopedic clinical fixation applications. Therefore, we chose the nHA/PA66/YTZ composite with 40% YTZ for further investigations in this study.
For the cytology experiments, the results of the CCK-8 experiment were indicative that the nHA/PA66/YTZ composite had no cytotoxicity and could support proliferation of MC3T3-E1 cells, suggestive that the nHA/PA66/YTZ had favorable cell affinity and excellent biocompatibility. The cell spreading and adhesion on biomaterials plays a vital role in influencing the subsequent performance of the implants, as well as providing evidence of the cell–material interaction.15,39,40 Cells exert their biological functions (proliferation, differentiation, maturation, etc.) only after adhering with the biomaterials. Therefore, the initial attachment and subsequent proliferation of MC3T3-E1 cells on the nHA/PA66 and nHA/PA66/YTZ were observed by using SEM and confocal laser scanning microscopy to compare the biocompatibility and cell adhesion promoting properties between those biomaterials. Cell microfilaments play a critical role in a series of cell biological functions, such as attachment, spreading, movement, endocytosis, cell division and so on.31 Increased cell microfilament formation would indicate the better cell–materials contact and cell biological function. Meanwhile, the fully stretching pseudopodia play a vital role in the connection of cells with each other, which also causes the cells to attach tightly to the surface of the scaffold. MC3T3-E1 cells in the nHA/PA66/YTZ group were found to have more microfilaments and pseudopods compared with those in the nHA/PA66 and blank control groups, suggesting cells spread more easily on the nHA/PA66/YTZ material. Moreover, it was discovered that cells were more inclined to adhere on nHA/PA66/YTZ scaffolds and the spindle-shaped cells were more common on these nHA/PA66/YTZ scaffolds. Some research proved that more cells adhesion and spindle-shaped cells are indicative of a more optimal osteogenic differential ability.41,42 In some previous studies, it was suggested that the long and spindle-shaped cells had a tendency to express higher osteogenetic ability than cells with round and polygon shapes.43,44 In this study, the cells cultured on nHA/PA66/YTZ scaffolds exhibited more adhesion and a favorable shape (long and spindle-shaped), which might be beneficial to the formation of new bone, which might increase the success rate of implantation. Thus, it was suggested that nHA/PA66/YTZ composite has better bioactivity and biocompatibility compared to nHA/PA66.
To determine the osteogenetic ability of different samples on MC3T3-E1, western blots and alizarin red staining were used for evaluate the variation of the expression levels of osteogenic differentiation-related proteins (COL-1, OP, and OC). Osteogenic differentiation of MC3T3-E1 cells is an important aspect of bone repair. Expression of osteogenesis-related proteins, including COL-1, OP, and OC, reflects osteoblastic differentiation of MC3T3-E1. Meanwhile, the evaluation of the final differentiation phase was always carried out by the detection of calcium nodules. At each observe time point observed, western blots showed that expression of osteogenesis-related proteins (COL-1, OP and OC) increased in the nHA/PA66/YTZ group compared to the other groups. In addition, alizarin red staining reflected the situation of matrix mineralization and the result also exhibited the same experimental outcome. The results were indicative that nHA/PA66/YTZ had no negative effect on either the expression of osteogenic indicators, or the calcium nodules formation of MC3T3-E1 cells. Compared with other widely applied clinical biodegradable composites, like polylactic acids, the nHA/PA66/YTZ composite has the advantage of neither releasing lactic acid with a toxic effect nor decreasing the pH, which would not inhibit osteoblastic differentiation and proliferation of MC3T3-E1 cells.45 Therefore, the nHA/PA66/YTZ composite could be implanted in the human body safely. In consideration of nHA/PA66/YTZ having good biocompatibility and biomechanical behaviors similar to human bone, we could avoid doing a second surgery to remove the implant after bone healing if the composite could be applied to the clinical situation. The limitation of this study is the lack of an in vivo study to compare the effects of biocompatibility and bioactivity between the nHA/PA66/YTZ composite and other orthopedic implants. We need to carry out further studies in vivo, especially the follow-up of the effects of long-term implantation. Moreover, the optimal mass ratio is worth further study.
Conclusion
A novel composite of YTZ-reinforced nHA/PA66 was prepared in this study. The addition of YTZ into nHA/PA66 significantly improved the mechanical and biological properties of the nHA/PA66/YTZ composite. The best mechanical properties were achieved when the YTZ
:
nHA mass ratio was 40
:
60, which were higher than those of human cortical bone. Cytology experiments in vitro showed that nHA/PA66/YTZ could facilitated cell adhesion, proliferation and differentiation, which is indicative that this ternary composite has good biocompatibility and bioactivity. In summary, the nHA/PA66/YTZ composite exhibited good mechanical and biological properties, and has broad application prospects in the future.
Acknowledgements
This study was supported by grants of National Natural Science Foundation of China (81501876), and the Key Medical Program of Science and Technology Development of Chongqing (2013-1-011).
References
- Y. Zhang, Z. Quan, Z. Zhao, X. Luo, K. Tang and J. Li, et al., Evaluation of anterior cervical reconstruction with titanium mesh cages versus nano-hydroxyapatite/polyamide66 cages after 1- or 2-level corpectomy for multilevel cervical spondylotic myelopathy: a retrospective study of 117 patients, PLoS One, 2014, 9(5), e96265 Search PubMed.
- P. Shokrollahi, H. Mirzadeh, O. A. Scherman and W. T. Huck, Biological and mechanical properties of novel composites based on supramolecular polycaprolactone and functionalized hydroxyapatite, J. Biomed. Mater. Res., Part A, 2010, 95(1), 209–221 CrossRef PubMed.
- H. Li, M. Gong, A. Yang, J. Ma, X. Li and Y. Yan, Degradable biocomposite of nano calcium-deficient hydroxyapatite-multi(amino acid) copolymer, Int. J. Nanomed., 2012, 7, 1287–1295 CrossRef CAS PubMed.
- H. Wang, Y. Li, Y. Zuo, J. Li, S. Ma and L. Cheng, Biocompatibility and osteogenesis of biomimetic nano-hydroxyapatite/polyamide composite scaffolds for bone tissue engineering, Biomaterials, 2007, 28(22), 3338–3348 CrossRef CAS PubMed.
- X. Zhang, Y. Zhang, X. Zhang, Y. Wang, J. Wang and M. Lu, et al., Mechanical properties and cytocompatibility of carbon fibre reinforced nano-hydroxyapatite/polyamide66 ternary biocomposite, J. Mech. Behav. Biomed. Mater., 2015, 42, 267–273 CrossRef CAS PubMed.
- B. Qiao, J. Li, Q. Zhu, S. Guo, X. Qi and W. Li, et al., Bone plate composed of a ternary nano-hydroxyapatite/polyamide 66/glass fiber composite: biomechanical properties and biocompatibility, Int. J. Nanomed., 2014, 9, 1423–1432 CrossRef PubMed.
- B. Su, X. Peng, D. Jiang, J. Wu, B. Qiao and W. Li, et al., In vitro and in vivo evaluations of nano-hydroxyapatite/polyamide 66/glass fibre (n-HA/PA66/GF) as a novel bioactive bone screw, PLoS One, 2013, 8(7), e68342 CAS.
- Y. Zhang, X. Deng, D. Jiang, X. Luo, K. Tang and Z. Zhao, et al., Long-term results of anterior cervical corpectomy and fusion with nano-hydroxyapatite/polyamide 66 strut for cervical spondylotic myelopathy, Sci. Rep., 2016, 6, 26751 CrossRef CAS PubMed.
- P. Yang, C. Bian, X. Huang, A. Shi, C. Wang and K. Wang, Core decompression in combination with nano-hydroxyapatite/polyamide 66 rod for the treatment of osteonecrosis of the femoral head, Archives of Orthopaedic and Traumatic Surgery, 2014, 134(1), 103–112 CrossRef PubMed.
- X. Yang, Y. Song, L. Liu, H. Liu, J. Zeng and F. Pei, Anterior reconstruction with nano-hydroxyapatite/polyamide-66 cage after thoracic and lumbar corpectomy, Orthopedics, 2012, 35(1), e66–e73 Search PubMed.
- Z. Zhao, D. Jiang, Y. Ou, K. Tang, X. Luo and Z. Quan, A hollow cylindrical nano-hydroxyapatite/polyamide composite strut for cervical reconstruction after cervical corpectomy, J. Clin. Neurosci., 2012, 19(4), 536–540 CrossRef CAS PubMed.
- J. Li, Y. Man, Y. Zuo, L. Zhang, C. Huang and M. Liu, et al., In vitro and In vivo Evaluation of a nHA/PA66 Composite Membrane for Guided Bone Regeneration, J. Biomater. Sci., Polym. Ed., 2011, 22(1–3), 263–275 CrossRef CAS PubMed.
- Y. Xiong, C. Ren, B. Zhang, H. Yang, Y. Lang and L. Min, et al., Analyzing the behavior of a porous nano-hydroxyapatite/polyamide 66 (n-HA/PA66) composite for healing of bone defects, Int. J. Nanomed., 2014, 9, 485–494 CrossRef PubMed.
- C. Knabe, F. Klar, R. Fitzner, R. J. Radlanski and U. Gross, In vitro investigation of titanium and hydroxyapatite dental implant surfaces using a rat bone marrow stromal cell culture system, Biomaterials, 2002, 23(15), 3235–3245 CrossRef CAS PubMed.
- S. Ozawa and S. Kasugai, Evaluation of implant materials (hydroxyapatite, glass-ceramics, titanium) in
rat bone marrow stromal cell culture, Biomaterials, 1996, 17(1), 23–29 CrossRef CAS PubMed.
- H. K. Uhthoff and M. Finnegan, The effects of metal plates on post-traumatic remodelling and bone mass, J. Bone Jt. Surg., Br. Vol., 1983, 65(1), 66–71 CrossRef CAS.
- N. Sumitomo, K. Noritake, T. Hattori, K. Morikawa, S. Niwa and K. Sato, et al., Experiment study on fracture fixation with low rigidity titanium alloy: plate fixation of tibia fracture model in rabbit, J. Mater. Sci.: Mater. Med., 2008, 19(4), 1581–1586 CrossRef CAS PubMed.
- A. M. Ballo, E. A. Akca, T. Ozen, L. Lassila, P. K. Vallittu and T. O. Narhi, Bone tissue responses to glass fiber-reinforced composite implants–a histomorphometric study, Clin. Oral Implants Res., 2009, 20(6), 608–615 CAS.
- E. G. Basaran, E. Ayna, P. K. Vallittu and L. V. Lassila, Load bearing capacity of fiber-reinforced and unreinforced composite resin CAD/CAM-fabricated fixed dental prostheses, J. Prosthet. Dent., 2013, 109(2), 88–94 CrossRef CAS PubMed.
- N. Saito, K. Aoki, Y. Usui, M. Shimizu, K. Hara and N. Narita, et al., Application of carbon fibers to biomaterials: a new era of nano-level control of carbon fibers after 30-years of development, Chem. Soc. Rev., 2011, 40(7), 3824–3834 RSC.
- R. Quan, D. Yang, X. Wu, H. Wang, X. Miao and W. Li, In vitro and in vivo biocompatibility of graded hydroxyapatite–zirconia composite bioceramic, J. Mater. Sci.: Mater. Med., 2008, 19(1), 183–187 CrossRef CAS PubMed.
- T. J. Matsumoto, S. H. An, T. Ishimoto, T. Nakano, T. Matsumoto and S. Imazato, Zirconia-hydroxyapatite composite material with micro porous structure, Dent. Mater., 2011, 27(11), e205–e212 CrossRef CAS PubMed.
- V. Sollazzo, F. Pezzetti, A. Scarano, A. Piattelli, C. A. Bignozzi and L. Massari, et al., Zirconium oxide coating improves implant osseointegration in vivo, Dent. Mater., 2008, 24(3), 357–361 CrossRef CAS PubMed.
- A. H. De Aza, J. Chevalier, G. Fantozzi, M. Schehl and R. Torrecillas, Crack growth resistance of alumina, zirconia and zirconia toughened alumina ceramics for joint prostheses, Biomaterials, 2002, 23(3), 937–945 CrossRef CAS PubMed.
- R. J. Kohal, M. Wolkewitz and C. Mueller, Alumina-reinforced zirconia implants: survival rate and fracture strength in a masticatory simulation trial, Clin. Oral Implants Res., 2010, 21(12), 1345–1352 CrossRef PubMed.
- M. Elpers, D. Nam, S. Boydston-White, M. P. Ast, T. M. Wright and D. E. Padgett, Zirconia phase transformation, metal transfer, and surface roughness in retrieved ceramic composite femoral heads in total hip arthroplasty, J. Arthroplasty, 2014, 29(11), 2219–2223 CrossRef PubMed.
- G. Abedi, A. Jahanshahi, M. H. Fathi, I. S. Haghdost and A. Veshkini, Study of nano-hydroxyapatite/zirconia stabilized with yttria in bone healing: histopathological study in rabbit model, Pol. J. Pathol., 2014, 1, 40–47 CrossRef.
- J. F. Bartolome, J. S. Moya, R. Couceiro, C. F. Gutierrez-Gonzalez, F. Guitian and A. Martinez-Insua, In vitro and in vivo evaluation of a new zirconia/niobium biocermet for hard tissue replacement, Biomaterials, 2016, 76, 313–320 CrossRef CAS PubMed.
- I. Mihatovic, V. Golubovic, J. Becker and F. Schwarz, Bone tissue response to experimental zirconia implants, Clin. Oral Investig., 2016 DOI:10.1007/s00784-016-1904-2.
- R. X. Shao, R. F. Quan, X. L. Huang, T. Wang, S. J. Xie and H. H. Gao, et al., Evaluation of porous gradient hydroxyapatite/zirconia composites for repair of lumbar vertebra defect in dogs, J. Biomater. Appl., 2016, 30(9), 1312–1321 CrossRef CAS PubMed.
- R. Ma, S. Tang, H. Tan, W. Lin, Y. Wang and J. Wei, et al., Preparation, characterization, and in vitro osteoblast functions of a nano-hydroxyapatite/polyetheretherketone biocomposite as orthopedic implant material, Int. J. Nanomed., 2014, 9, 3949–3961 Search PubMed.
- F. A. Barber, W. D. Dockery and S. A. Hrnack, Long-term degradation of a poly-lactide co-glycolide/beta-tricalcium phosphate biocomposite interference screw, Arthroscopy: The Journal of Arthroscopic & Related Surgery, 2011, 27(5), 637–643 Search PubMed.
- Y. Cui, Y. Liu, Y. Cui, X. Jing, P. Zhang and X. Chen, The nanocomposite scaffold of poly(lactide-co-glycolide) and hydroxyapatite surface-grafted with L-lactic acid oligomer for bone repair, Acta Biomater., 2009, 5(7), 2680–2692 CrossRef CAS PubMed.
- H. K. Uhthoff, D. Boisvert and M. Finnegan, Cortical porosis under plates. Reaction to unloading or to necrosis?, J. Bone Jt. Surg., Am. Vol., 1994, 76(10), 1507–1512 CrossRef CAS.
- F. Witte, V. Kaese, H. Haferkamp, E. Switzer, A. Meyer-Lindenberg and C. J. Wirth, et al., In vivo corrosion of four magnesium alloys and the associated bone response, Biomaterials, 2005, 26(17), 3557–3563 CrossRef CAS PubMed.
- G. M. Erickson, J. Catanese 3rd and T. M. Keaveny, Evolution of the biomechanical material properties of the femur, Anat. Rec., 2002, 268(2), 115–124 CrossRef PubMed.
- W. T. Lee, J. Y. Koak, Y. J. Lim, S. K. Kim, H. B. Kwon and M. J. Kim, Stress shielding and fatigue limits of poly-ether-ether-ketone dental implants, J. Biomed. Mater. Res., Part B, 2012, 100(4), 1044–1052 CrossRef PubMed.
- F. Sarsilmaz, N. Orhan, E. Unsaldi, A. S. Durmus and N. Colakoglu, A polyethylene-high proportion hydroxyapatite implant and its investigation in vivo, Acta Bioeng. Biomech., 2007, 9(2), 9–16 CAS.
- A. Gyorgyey, K. Ungvari, G. Kecskemeti, J. Kopniczky, B. Hopp and A. Oszko, et al., Attachment and proliferation of human osteoblast-like cells (MG-63) on laser-ablated titanium implant material, Mater. Sci. Eng., C, 2013, 33(7), 4251–4259 CrossRef CAS PubMed.
- H. Tan, S. Guo, S. Yang, X. Xu and T. Tang, Physical characterization and osteogenic activity of the quaternized chitosan-loaded PMMA bone cement, Acta Biomater., 2012, 8(6), 2166–2174 CrossRef CAS PubMed.
- U. Meyer, A. Buchter, H. P. Wiesmann, U. Joos and D. B. Jones, Basic reactions of osteoblasts on structured material surfaces, Eur. Cells Mater., 2005, 9, 39–49 CrossRef CAS PubMed.
- K. A. Kilian, B. Bugarija, B. T. Lahn and M. Mrksich, Geometric cues for directing the differentiation of mesenchymal stem cells, Proc. Natl. Acad. Sci. U. S. A., 2010, 107(11), 4872–4877 CrossRef CAS PubMed.
- T. P. Kunzler, C. Huwiler, T. Drobek, J. Voros and N. D. Spencer, Systematic study of osteoblast response to nanotopography by means of nanoparticle-density gradients, Biomaterials, 2007, 28(33), 5000–5006 CrossRef CAS PubMed.
- P. S. Mathieu and E. G. Loboa, Cytoskeletal and focal adhesion influences on mesenchymal stem cell shape, mechanical properties, and differentiation down osteogenic, adipogenic, and chondrogenic pathways, Tissue Eng., Part B, 2012, 18(6), 436–444 CrossRef CAS PubMed.
- T. Chen, Y. Zhou and W. S. Tan, Influence of lactic acid on the proliferation, metabolism, and differentiation of rabbit mesenchymal stem cells, Cell Biol. Toxicol., 2009, 25(6), 573–586 CrossRef CAS PubMed.
|
This journal is © The Royal Society of Chemistry 2016 |
Click here to see how this site uses Cookies. View our privacy policy here.