DOI:
10.1039/C6RA24397E
(Paper)
RSC Adv., 2016,
6, 112166-112174
Preparation of magnetic activated carbon from waste rice husk for the determination of tetracycline antibiotics in water samples
Received
30th September 2016
, Accepted 4th November 2016
First published on 4th November 2016
Abstract
In this work, the silica in rice husk was removed to create a natural template to prepare porous activated carbon. The preparation process of the activated carbon (AC) from waste rice husk consisted of carbonization, silica removal and activation. Factors affecting the preparation of AC, including the mass ratio of ZnCl2 and rice husk carbon (RHC), activation temperature and time were investigated. Based on the Brunauer–Emmett–Teller (BET) results, the specific surface area of AC prepared under optimized conditions was as high as 1673.25 m2 g−1. Then, magnetic activated carbon (mag-AC) was synthesized by in situ co-precipitation of rice husk-based activated carbon and Fe3O4. The specific surface area and average pore diameter of mag-AC were respectively 986.97 m2 g−1 and 3.62 nm, respectively. It was shown that mag-AC was a viable adsorbent for magnetic extraction of tetracycline antibiotics in water samples followed by quantification by HPLC-MS/MS. The results indicated satisfactory correlation coefficients (R2) ranging from 0.9955 to 0.9998. Limits of detection (LOD) and quantization (LOQ) were achieved in the range of 0.10 to 0.95 and 0.35 to 2.85 μg L−1 in water samples, respectively.
1. Introduction
The widespread use of antibiotics has become a serious problem because it has a variety of potential adverse effects, including acute and chronic toxicity, impact on aquatic photosynthetic organisms, disruption of indigenous microbial populations, and development of antibiotic-resistant genes among microorganisms.1 Tetracycline antibiotics (TCs), including oxytetracycline, chlortetracycline and tetracycline, are widely applied in human therapy and the farming industry due to their low cost, high quality and desirable antimicrobial activity.2,3 Most of the TCs used in the livestock industry are excreted through feces and urine as unmodified parent compounds.4 As a result, TCs are directly or indirectly discharged into water, which has caused serious pollution. Contamination of TCs in water is not only detrimental to human health, but also causes damage to other individual species, populations and natural biological communities. Therefore, it is necessary to explore efficient and cost-effective treatment technologies to determine TCs in water.
In order to detect the content of TCs in water samples, the TCs must be concentrated to meet the detection limits of the instruments. In the process of concentration, adsorbents play an important role. To date, scientists have investigated the adsorption of tetracycline antibiotics by several materials, such as graphene oxide,5 single-walled carbon nanotubes and multi-walled carbon nanotubes,6,7 activated carbon (AC),8 smectite clay,9 chitosan particles,10 aluminum oxide,11 and rectorite.12 Among these, carbon-based adsorbents possess large surface areas with abundant pore structures and have shown great potential in the adsorption of TCs from aqueous solutions.13 Although these adsorbents are effective in the adsorption of TCs, they have a common shortcoming in that the materials are not easily separated from the aqueous solutions after the TCs are adsorbed. Because these adsorbents are granular or powdered, long centrifuge, precipitation or filtering times are generally required. To solve this problem, magnetic carbon composites have been introduced. Magnetic materials have the following advantage compared with traditional adsorbents: the separation process can be performed directly in crude samples containing suspended solid materials using a magnetic field without the need for additional centrifugation or filtration; therefore, separation is easier and faster.14 Thus, there is increasing demand for the development of cost-effective and efficient magnetic carbon materials for the adsorption of pollutants in water samples.
AC has a high surface area, numerous active sites and a porous structure; therefore, it offers an attractive and inexpensive option for the adsorption of TCs from water.15 Fe3O4, a phase of iron oxide, has numerous applications due to its extraordinary magnetic and optical properties. The introduction of a magnetic medium (such as Fe3O4) to carbon-based adsorbents by a chemical co-precipitation reaction is an efficient method to enable the adsorbent to be efficiently separated with an external magnetic field.16 Fu et al. prepared amino-functionalized magnetic activated carbon (AC/Fe3O4@SiO2–NH2) by a classical co-precipitation method.17 It is expected that composite materials containing magnetic activated carbon (mag-AC) will not only be high-efficiency adsorbents for the adsorption of TCs from aqueous solutions, but can also be easily collected magnetically after adsorption.
Many studies have been performed with the aim of obtaining low cost AC from renewable sources, mainly from agricultural wastes. Thus, a wide variety of agricultural and industrial wastes are frequently used as AC precursors, such as olive husks,18 coconut shells,19 coffee endocarp,20 buriti shells,21 flamboyant pods,22 macadamia nut shells23 and rice husk.24–26 AC from rice husk is obtained by activation with physical or chemical methods.26,27 Generally, AC with a high specific surface area can be acquired at low temperature by chemical methods. Activation agents, including KOH, H3PO4, K2CO3, and ZnCl2, are widely employed to activate carbon.26 The use of suitable activation agents to create microporous structures can result in AC with a wide pore size distribution during the activation process. Among these, ZnCl2 is a widely used activation agent for obtaining porous carbon with high specific surface area and developed porosity.28 Liu et al. used rice husk as a precursor for the production of activated carbon with high specific surface area by a ZnCl2–CuCl2 composite activator.28
In the present study, mag-AC was prepared from rice husk. Rice husk is a common agricultural byproduct with the characteristics of hard surface, high silica content, and poor decomposition in nature.24 The contents of xylan and amorphous silica in rice husk are each around 20% (wt%). In our work, the abundant silica in rice husk was used as a natural template. The silica was removed during the preparation of activated carbon and the obtained products were porous, with high specific surface areas. For these reasons, rice husk is an ideal carbon-based precursor for integrating magnetic porous carbon. Nitrogen (N2) BET (Brunauer–Emmett–Teller) surface area, scanning electron microscopy (SEM), X-ray diffraction (XRD) and energy dispersive spectrometry (EDS) were applied to characterize the materials. Then, mag-AC was used to adsorb TCs from water; the TCs were then determined by HPLC-MS/MS. To the best of our knowledge, little is known about the preparation of mag-AC based on rice husk and its application for TCs absorption in water samples.
2. Experimental
2.1 Materials and reagents
Rice husk was obtained from local markets in Hangzhou (China); FeCl3·6H2O (analytically pure) and FeCl2·4H2O (analytically pure) were purchased from Aladdin Reagent; NH3·H2O (analytically pure) was purchased from Hangzhou Longshan Fine chemical engineering company; HNO3 (analytically pure) was purchased from Zhejiang Zhongxing chemistry engineering company; deionized water was obtained from Hangzhou Wahaha Corporation; HF (analytically pure), methanol (HPLC grade), acetonitrile (HPLC grade), acetone (HPLC grade) and ethyl acetate (HPLC grade) were purchased from Shanghai Lingfeng chemical reagent company; the 3 tetracycline antibiotics (oxytetracycline, chlortetracycline and tetracycline) were purchased from Dr Ehrenstorfer GmbH; standard solutions of the 3 tetracycline antibiotics were prepared in deionized water at a concentration of 1 mg L−1. Intermediate solutions used for spiking water samples were prepared via serial dilution of the stock solution to the necessary concentrations.
2.2 Carbonization of rice husk and silica removal
In this work, rice husk was carbonized at 400 °C for 2 h in a crucible. After grinding, the powdered materials were soaked in hydrofluoric acid (10%, wt%) for 24 h to remove silica. After the process of filtration, the filtrate was washed with distilled water until neutral and dried at 80 °C overnight to prepare the activated carbon, marked as RHC (rice husk carbon). The hydrofluoric acid was collected and recycled.
2.3 Preparation of activated carbon
The RHC was impregnated with ZnCl2 solution in a crucible, and the weight ratio of ZnCl2 to RHC was varied from 2
:
1 to 5
:
1. After stirring for 1 h at room temperature, the mixture was heated to 400 °C for 40 min to remove water. Then, the mixture was heated to the activation temperature (500 °C, 650 °C, 750 °C, or 850 °C) for 0.5 to 2 h. Finally, the product was washed and dried in an oven at 80 °C overnight to obtain the final product, marked as AC.
2.4 Preparation of mag-AC
3 g AC was refluxed with 30 mL nitric acid (63%) for 5 h at 60 °C to generate carboxyl and hydroxyl groups. Mag-AC was synthesized by the in situ chemical co-precipitation of Fe3+ and Fe2+ (the molar ratio of Fe3+ and Fe2+ was 2
:
1) in an alkaline solution containing acid-treated AC.17 Briefly, 2 g acid-treated AC was dispersed in 80 mL distilled water by ultrasonic treatment. Then, 2.37 g FeCl3·6H2O and 0.87 g FeCl2·4H2O were added to the above mixture and maintained with vigorous stirring under N2 atmosphere for 0.5 h. 30 mL concentrated NH3·H2O was added into the mixture dropwise, and then the mixture was heated to 60 °C and reacted for 2 h. After the reaction, the precipitate was separated with a magnet. Finally, the precipitate was washed with distilled water and dried at 60 °C for 24 h to obtain the product, marked as mag-AC.
2.5 Extraction of tetracycline antibiotics from water samples by mag-AC
The procedure to extract tetracycline antibiotics is demonstrated in Fig. 1. In brief, 10 mL of aqueous sample was added to a 20 mL vial; then, 20 mg mag-AC was dispersed into the above solution with ultrasonic treatment to form a homogeneous solution. During the extraction process, the mag-AC tumbled freely in the 10 mL solution. After 5 min of extraction, a magnet was attached outside the vial wall, and mag-AC was quickly gathered at the side of the vial. After about 1 min, the suspension became limpid and the supernatant was decanted. Then, the pre-concentrated target analytes were eluted from the mag-AC with 2.0 mL acetone. After placing a magnet at the bottom of the vial, the supernatant solution was collected using a pipette and evaporated to dryness under a mild nitrogen stream. The residue was dissolved in 1 mL of mixture solution (acetonitrile–water, 1
:
1, v/v), 5 μL of which was analyzed by HPLC-MS/MS.
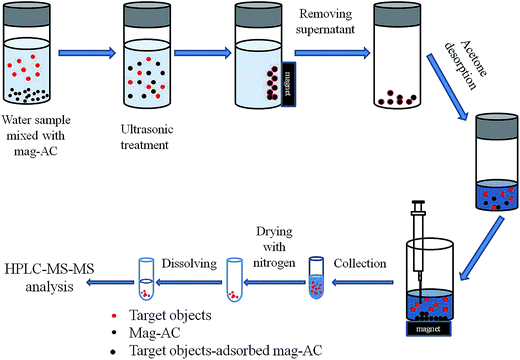 |
| Fig. 1 Extraction procedure of tetracycline antibiotics from water samples. | |
3. Results and discussion
3.1 Characterization of materials
3.1.1 X-ray diffraction (XRD). The phases and structures of AC and mag-AC were analyzed by XRD (PANalytical X'Pert Pro X-ray diffraction, employing Cu Kα radiation at a scanning rate of 6° min−1 with 2θ ranging from 10 to 80°). AC-2, AC-3, AC-4, and AC-5 respectively correspond to mass ratios of ZnCl2
:
RHC = 2
:
1, 3
:
1, 4
:
1, and 5
:
1. As shown in Fig. 2, the XRD pattern of AC presents carbon peaks, including large, broad amorphous carbon peaks from 20° to 30° and from 40° to 47°. Among these, localized graphitization peaks appeared at about 27° in the XRD patterns of AC-3 and AC-4; the localized graphitization peak of AC-3 was much sharper than that of AC-4. Meanwhile, there was no silica peak in the AC patterns, which suggests that the silica was removed completely. In the XRD pattern of mag-AC, new and significant diffraction peaks at 2θ = 30.21°, 35.54°, 43.24°, 53.71°, 57.21°, and 62.77° matched well with the data of JCPDS card no. 19-0629, which can be indexed as the face-centered cubic structure of Fe3O4 with corresponding diffraction peaks of the (220), (311), (400), (422), (511) and (440) planes. This confirmed that Fe3O4 was loaded on the AC.
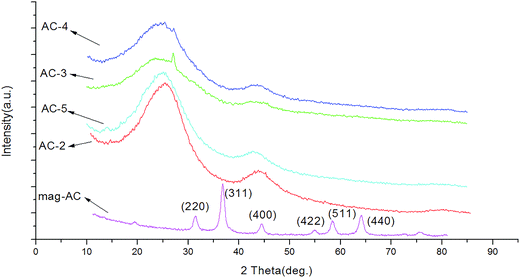 |
| Fig. 2 XRD patterns of AC and mag-AC. | |
3.1.2 FT-IR analysis. The organic functional groups on the surface of AC, acid-treated AC and mag-AC were characterized by FT-IR spectra (Nicolet 7700 FT-IR spectrometer), as shown in Fig. 3. The peaks that appeared at 3424 cm−1 for all the samples were attributed to the hydrogen bonded O–H (carboxyl or hydroxyl) stretching vibration, and the peaks at 2922 cm−1 represented the stretching vibration of –CH2.31,32 The peaks at 1606 and 1452 cm−1 can be ascribed to C
C vibrations. With nitric acid treatment, the peak at 1701 cm−1 can be assigned to C
O vibration, and the peak appearing at 2515 cm−1 corresponds to the shift of O–H (carboxyl),32 which reveals the existence of carboxyl groups. The peak at 1795 cm−1 was ascribed to the heterocyclic ring structure.33 In the infrared spectrum of mag-AC, the carboxyl peaks have almost disappeared because of the involvement of carboxyl groups in the sorption of iron ions during the preparation process.17
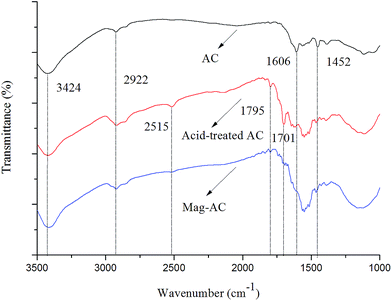 |
| Fig. 3 FT-IR spectra of AC, acid-treated AC and mag-AC. | |
3.1.3 Scanning electron microscopy (SEM) and energy dispersive spectrometry (EDS). The morphologies of RHC (Fig. 4), AC (Fig. 4), and mag-AC (Fig. 5) were characterized by scanning electron microscopy (SEM) using a Hitachi S-4700 instrument. Fig. 6 shows the EDS spectrum and element content of mag-AC.
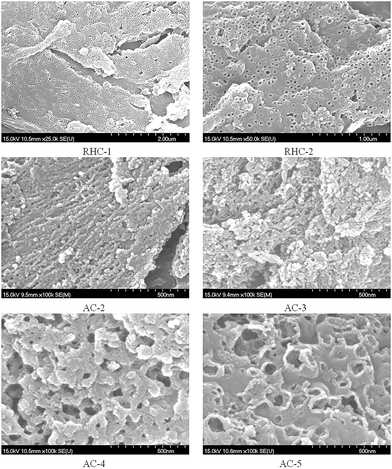 |
| Fig. 4 Scanning electron micrographs of RHC and AC. | |
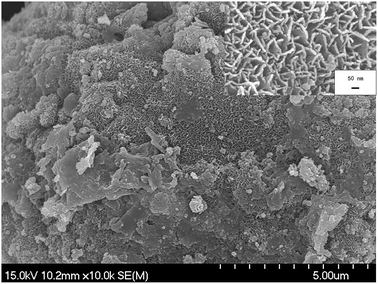 |
| Fig. 5 Scanning electron micrographs of mag-AC. | |
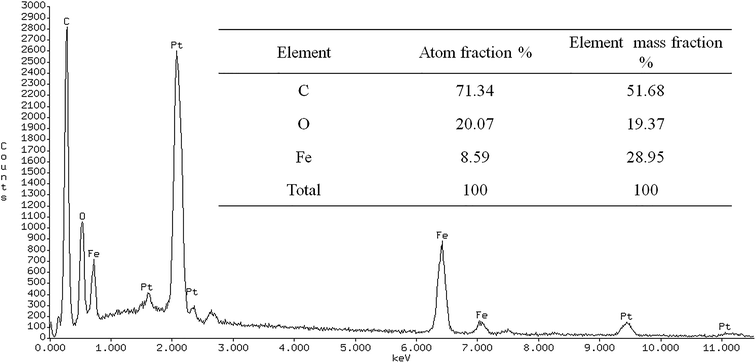 |
| Fig. 6 EDS spectrum and element content of mag-AC. Platinum was splashed on the surface of mag-AC in order to obtain clear scanning electron micrographs. | |
RHC-1 and RHC-2 are the microstructures of rice husk carbon which was treated with hydrofluoric acid. Rice husk is viewed as a natural template material due to the presence of a silica template. After HF treatment, the silica in rice husk carbon was removed, exposing abundant pores. This pore structure is conducive to the activation process of RHC by ZnCl2.
With increasing ZnCl2
:
RHC ratio, the structure of AC became increasingly porous. Fig. 4(AC-4) shows that a well-developed pore structure emerged in the AC, and the diameters of the pores were distributed between 10 and 70 nm. However, when the ratio reached 5
:
1, the pore diameters of AC became larger, and pores greater than 100 nm appeared in the AC. The pores expanded due to the expansion and corrosion effects of excess ZnCl2 during activation, which led to a decline in the specific surface area and the adsorption capacity of AC. Therefore, the mass ratio of ZnCl2
:
RHC = 4
:
1 was selected as an optimal condition in the preparation of AC.
Fig. 5 shows that tile-like Fe3O4 is embedded on the AC surface, and numerous pores formed between the sheets. During the preparation of mag-AC, AC was oxidized with HNO3 to generate carboxyl and hydroxyl groups. On the one hand, these groups increase the dispersion of AC in water. On the other hand, the groups can coordinate Fe3+ and Fe2+ under alkaline conditions, which leads to uniform distribution of Fe3O4 and prevents its agglomeration. As can be seen from Fig. 6, the EDS shows that mag-AC contains the elements C, O, and Fe, which further proves the existence of Fe3O4 in the activated carbon. Additionally, during the experiment, an external magnet was used to separate the mag-AC and the liquid phase. The procedure was finished in a very short time. As shown in Fig. 7, the solution became clarified in 60 s, which proves that mag-AC has excellent magnetic properties before and after adsorption.
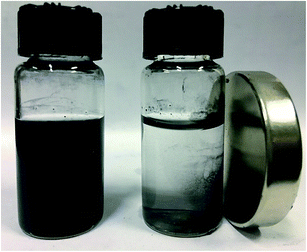 |
| Fig. 7 Image of mag-AC dispersed in water and mag-AC held in place by an external magnet. | |
3.1.4 N2 adsorption/desorption analysis. The specific surface areas of the samples were measured at 77 K by N2 adsorption/desorption isotherms using an ASAP 2020 Micromeritics instrument and by the Brunauer–Emmett–Teller (BET) method. The pore size distribution was obtained from the desorption branches of the isotherms by the density functional theory (DFT) method. The single factor method was used to research the effects of different activation temperatures and activation times on the specific surface area of AC (Fig. 8), and the optimal conditions were obtained.
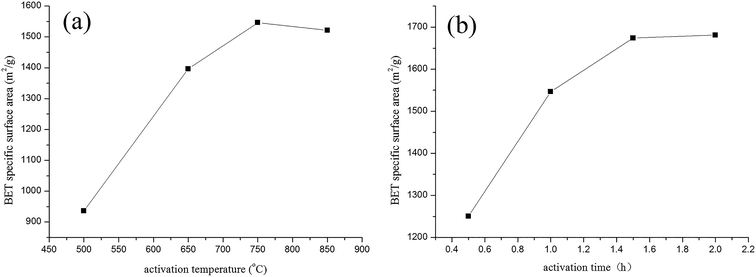 |
| Fig. 8 Effects of (a) activation temperature and (b) activation time on the BET specific surface area of activated carbon. | |
When the ratio of ZnCl2
:
RHC was 4
:
1 and the activation time was 1 h, the surface areas of AC were investigated at 500 °C, 650 °C, 750 °C, and 850 °C. Fig. 8(a) shows the specific surface area of AC at different activation temperatures. When the activation temperature was increased from 500 °C to 850 °C, the specific surface area of AC increased initially and then decreased. As shown in Fig. 8(a), the optimal activation temperature was clearly 750 °C.
When the ratio of ZnCl2
:
RHC was 4
:
1 and the activation temperature was 750 °C, the surface areas of AC were investigated at different times. Fig. 8(b) shows that the specific surface area of AC increased with activation time from 0.5 to 1.5 h and then displayed a slight increase with further extension of the activation time.
In summary, the optimal conditions for preparing activated carbon from rice husk are proposed as follows: the ratio of ZnCl2
:
RHC is 4
:
1; the activation time is 1.5 h; and the activation temperature is 750 °C. Under optimum conditions, the specific surface area of AC was as high as 1673.25 m2 g−1. Although the specific surface area of mag-AC decreased to 986.97 m2 g−1 after Fe3O4 was loaded on AC, the composites retained a satisfactory specific surface area. Mag-AC was expected to have excellent adsorption properties for the target substances.
Fig. 9(a) and (b) respectively present nitrogen adsorption isotherms of AC and mag-AC. According to IUPAC classification, AC can be defined as a typical type I isotherm, and mag-AC exhibited a type II curve; this reveals that the materials had a narrow pore size distribution. Meanwhile, the pore size distribution curves (Fig. 9(c) and (d)) further confirmed these results. It can be clearly observed that the specific surface area of the material decreased (1673.25 to 986.97 m2 g−1) after loading. From the pore size distribution curves of AC (Fig. 9(c)), it can be seen that the pores are smaller than 30 Å, which is in accordance with the average pore diameter of 2.27 nm. However, in Fig. 9(d), most of the pores are smaller than 40 Å, and the average pore diameter of mag-AC is 3.62 nm; this may be related to the coverage of Fe3O4 on AC, leading to a decrease in the number of micropores. Materials with this pore diameter facilitate the penetration of TC molecules.34
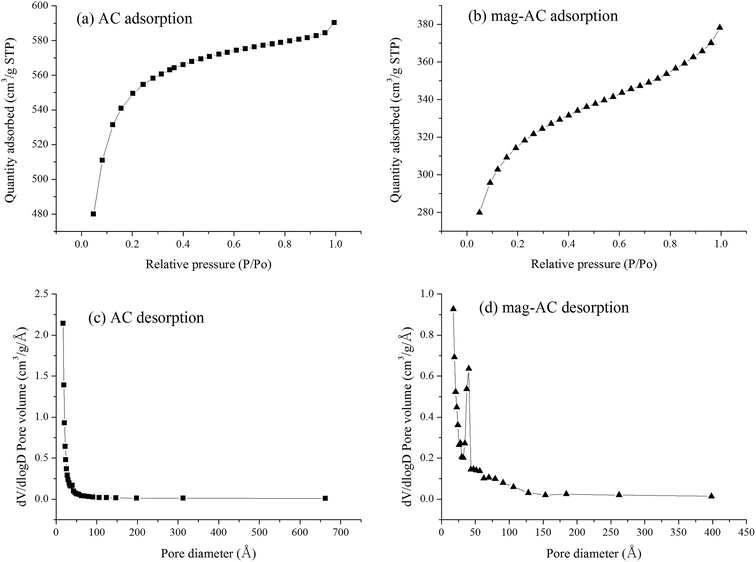 |
| Fig. 9 (a) N2 adsorption isotherm of AC. (b) N2 adsorption isotherm of mag-AC. (c) Pore size distribution of AC. (d) Pore size distribution of mag-AC. AC was prepared under the optimal conditions. | |
3.2 Extraction of tetracycline antibiotics in water samples by mag-AC
3.2.1 Optimization of extraction conditions. Standard solutions of tetracycline antibiotics were added to distilled water at a concentration of 20 μg L−1, and mag-AC was used for the extraction of 3 residual tetracycline antibiotics in water samples. The following parameters were optimized: (a) sample pH value; (b) amount of mag-AC; (c) extraction time; (d) type and amount of elution. The respective data and figures are given in Fig. 10.
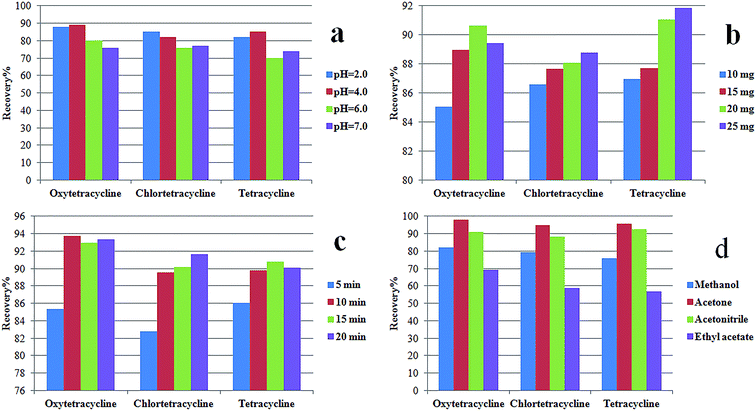 |
| Fig. 10 The effects of different influencing factors on the extraction efficiencies. (a) Effects of the pH value of the water samples. (b) Effects of the amount of sorbent in the water samples. (c) Investigation of the extraction time. (d) Optimization of the desorption solvent. | |
During the extraction procedure, tetracycline antibiotic molecules are readily adsorbed by mag-AC; however, the pH value has an effect on the molecular state of the target objects. Herein, 0.1 mol L−1 phosphate buffer was chosen to optimize the pH value of the solution. The optimal pH value was chosen according to the recoveries of 3 tetracycline antibiotics. The results are shown in Fig. 10(a); pH = 4.0 was chosen as the optimal value because of the high recoveries of the 3 antibiotics.
The pH value of the buffer system was set at 4.0, and 2 mL methanol was used as the desorption solvent. Amounts of mag-AC ranging from 5 to 25 mg were tested to adsorb antibiotics from water samples. As shown in Fig. 10(b), when the amounts of mag-AC were increased from 5 to 25 mg, the recovery of AC initially increased and then remained constant. Consequently, the optimal amount of mag-AC was fixed at 20 mg.
In order to study the effects of extraction time on extraction efficiency, experiments with different desorption times from 5 to 20 min were carried out. As displayed in Fig. 10(c), when the extraction time was varied from 5 to 10 minutes, the recovery of antibiotics increased apparently; however, the recovery changed only slightly over the next 10 minutes. Therefore, the best results were achieved in 10 minutes.
4 solvents, methanol, acetonitrile, acetone and ethyl acetate, were studied as desorption solvents to examine their effects on the extraction. The results showed that the best extraction efficiency was obtained using acetone as the desorption solvent (Fig. 10(d)).
The following experimental conditions were found to give the best results: (a) pH = 4.0; (b) extraction time = 10 min; (c) amount of mag-AC = 20 mg; (d) desorption solvent = acetone.
3.2.2 Linear range, detection limit, recoveries and repeatability. Standard solutions of tetracycline antibiotics were used to draw standard curves with the method proposed in this paper. The abscissa was the mass concentration of the compounds, and the ordinate was the peak area of instrument response. The limits of detection (LOD) and quantization (LOQ) were calculated based on S/N = 3 and 10, respectively (S stands for signal, N stands for noise). The linear regression data are shown in Table 1. The satisfactory correlation coefficients (R2) ranged from 0.9955 to 0.9998, and the LOD and LOQ ranged from 0.10 to 0.95 μg L−1 and 0.35 to 2.85 μg L−1 in water samples, respectively.
Table 1 R2, LOD and LOQ values of 3 tetracycline antibiotics for matrix standard linearity equations
Compound |
Linear range (μg L−1) |
R2 |
LOD (μg L−1) |
LOQ (μg L−1) |
Oxytetracycline |
1.0–100 |
0.9955 |
0.95 |
2.85 |
Chlortetracycline |
0.5–100 |
0.9998 |
0.32 |
0.98 |
Tetracycline |
0.5–100 |
0.9989 |
0.10 |
0.35 |
In order to ensure the accuracy of the quantitative analysis, data of the proposed method were obtained by analyzing 3 series of standard solutions with concentrations of 10 μg L−1, 20 μg L−1 and 50 μg L−1, respectively. For each concentration, five replicate extractions and determinations were performed under the optimized experimental conditions. The repeatability of the proposed method was determined by evaluating the relative standard deviations (RSD). As can be seen in Table 2, the method has good repeatability and high recoveries.
Table 2 Recoveries and relative standard deviations of 3 tetracycline antibiotics at specific concentrations
Compound |
10 μg L−1 |
20 μg L−1 |
50 μg L−1 |
Recovery/% |
RSD/% |
Recovery/% |
RSD/% |
Recovery/% |
RSD/% |
Oxytetracycline |
93 |
4.5 |
98 |
1.5 |
91 |
7.5 |
Chlortetracycline |
102 |
2.1 |
91 |
8.4 |
99 |
3.5 |
Tetracycline |
96 |
9.4 |
95 |
6.6 |
96 |
5.0 |
3.2.3 Comparison of this work with other similar work for determination of antibiotics. The performance of mag-AC and similar materials for the extraction and determination of antibiotics is shown in Table 3. Compared with other high quality adsorbents, the present work has the same advantages, such as wide linear range, excellent correlation coefficient, and low LOD and RSD. More importantly, the mag-AC was prepared from waste rice husk, which is extremely inexpensive compared to graphene or carbon nanotubes. Considering these results, mag-AC is a cost-effective, efficient and environmentally friendly material for the pre-concentration of tetracycline antibiotics in water.
Table 3 Comparison of the current work with other work for the determination of antibiotics
Adsorbent |
Antibiotics |
Sample |
Linearity (μg L−1) |
LOD (μg L−1) |
R2 |
RSD (%) |
Ref. |
Graphene oxide/polyethylene glycol (GO/PEG) |
Pefloxacin |
Water |
0.02 to 500 |
0.0045 to 0.0079 |
0.9974 to 0.9999 |
4.6 to 12.1 |
29 |
Norfloxacin |
Ciprofloxacin |
Enrofloxacin |
Lomefloxacin |
Magnetic graphene/carbon nanotubes (M-G/CNTs) |
Oxytetracycline |
Sewage water |
20 to 800 |
3.6 |
— |
5.8 |
30 |
Magnetic activated carbon (Mag-AC) |
Oxytetracycline |
Water |
1.0 to 100 |
0.10 to 0.95 |
0.9955 to 0.9998 |
1.5 to 9.4 |
This work |
Chlortetracycline |
0.5 to 100 |
Tetracycline |
0.5 to 100 |
4. Conclusions
In this study, the silica in rice husk was successfully removed to prepare activated carbon with a high specific surface area (1673.25 m2 g−1) and abundant pores under optimal conditions. Then, mag-AC was synthesized based on AC and dispersed Fe3O4 by a chemical co-precipitation method. The BET results showed that mag-AC retained a satisfactory specific surface area (986.97 m2 g−1). In order to evaluate the adsorption properties of mag-AC, a method was developed for rapid pre-concentration, separation and detection of TCs in water samples with mag-AC as a magnetic adsorbent. The results indicated that this method has the advantages of satisfactory recoveries, low detection limit, simplicity, high efficiency and low cost. Thus, mag-AC has great potential as an adsorbent for pre-concentration of other organic pollutants in environmental samples.
Acknowledgements
Support of this work by the National Key Technology Support Program of China (2014BAC03B03), the Nature Science Foundation of Zhejiang Province (LY16B050008), the Science and Technology Department of Zhejiang Province (2015C32006), and Hangzhou Qianjiang Distinguished Experts Project (2014) is gratefully acknowledged.
References
- L. L. Ji, W. Chen, L. Duan and D. Q. Zhu, Mechanisms for strong adsorption of tetracycline to carbon nanotubes: a comparative study using activated carbon and graphite as adsorbents, Environ. Sci. Technol., 2009, 43, 2322 CrossRef CAS PubMed.
- S. P. Kim, P. Eichhorn, J. N. Jensen, A. S. Weber and D. S. Aga, Removal of Antibiotics in Wastewater: Effect of Hydraulic and Solid Retention Times on the Fate of Tetracycline in the Activated Sludge Process, Environ. Sci. Technol., 2005, 39, 5816 CrossRef CAS PubMed.
- L. Zhang, X. Y. Song, X. Y. Liu, L. J. Yang, F. Pan and J. N. Lv, Studies on the removal of tetracycline by multi-walled carbon nanotubes, Chem. Eng. J., 2011, 178, 26 CrossRef CAS.
- L. N. Shao, Z. M. Ren, G. S. Zhang and L. L. Chen, Facile synthesis, characterization of a MnFe2O4/activated carbon magnetic composite and its effectiveness in tetracycline removal, Mater. Chem. Phys., 2012, 135, 16 CrossRef CAS.
- Y. Gao, Y. Li, L. Zhang, H. Huang, J. J. Hu, S. M. Shah and X. G. Su, Adsorption and removal of tetracycline antibiotics from aqueous solution by graphene oxide, J. Colloid Interface Sci., 2012, 368, 540 CrossRef CAS PubMed.
- P. Oleszczuk, B. Pan and B. S. Xing, Adsorption and Desorption of Oxytetracycline and Carbamazepine by Multiwalled Carbon Nanotubes, Environ. Sci. Technol., 2009, 43, 9167 CrossRef CAS PubMed.
- L. L. Ji, W. Chen, J. Bi, S. R. Zheng, Z. Y. Xu, D. Q. Zhu and P. J. Alvarez, Adsorption of tetracycline on single-walled and multi-walled carbon nanotubes as affected by aqueous solution chemistry, Environ. Toxicol. Chem., 2010, 29, 2713 CrossRef CAS PubMed.
- R. Ocampo-Perez, R. Leyua-Ramos, J. Rivera-Utrilla, J. V. Flores-Cano and M. Sanchez-Polo, Modeling adsorption rate of tetracyclines on activated carbons from aqueous phase, Chem. Eng. Res. Des., 2015, 104, 579 CrossRef CAS.
- L. Aristilde, C. Marichal, J. Miéhé-Brendlé, B. Lanson and L. Charlet, Interactions of Oxytetracycline With a Smectite Clay: A Spectroscopic Study With Molecular Simulations, Environ. Sci. Technol., 2010, 44, 7839 CrossRef CAS PubMed.
- A. L. P. F. Caroni, C. R. M. Lima, M. R. de Pereira and J. L. C. Fonseca, Tetracycline adsorption on chitosan: a mechanistic description based on mass uptake and zeta potential measurements, Colloids Surf., B, 2012, 100, 222 CrossRef CAS PubMed.
- W. R. Chen and C. H. Huang, Adsorption and transformation of tetracycline antibiotics with aluminum oxide, Chemosphere, 2010, 79, 779 CrossRef CAS PubMed.
- P. H. Chang, J. S. Jean, W. T. Jiang and Z. H. Li, Mechanism of tetracycline sorption on rectorite, Colloids Surf., A, 2009, 339, 94 CrossRef CAS.
- Q. Zhou, Z. Li, C. Shuang, A. Li, M. Zhang and M. Wang, Efficient removal of tetracycline by reusable magnetic microspheres with a high surface area, Chem. Eng. J., 2012, 210, 350 CrossRef CAS.
- Q. H. Wu, G. Y. Zhao, C. Feng, C. Wang and Z. Wang, Preparation of a graphene-based magnetic nanocomposite for the extraction of carbamate pesticides from environmental water samples, J. Chromatogr. A, 2011, 1218, 7936 CrossRef CAS PubMed.
- K. Gadkaree, Carbon honeycomb structures for adsorption applications, Carbon, 1998, 36, 981 CrossRef CAS.
- O. Duman, S. Tunc, T. G. Polat and B. K. Bozoglan, Synthesis of magnetic oxidized multiwalled carbon nanotube–κ-carrageenan–Fe3O4 nanocomposite adsorbent and its application in cationic methylene blue dye adsorption, Carbohydr. Polym., 2016, 147, 79 CrossRef CAS PubMed.
- R. Q. Fu, Y. Liu, Z. M. Lou, Z. X. Wang, S. A. Baig and X. H. Xu, Adsorptive removal of Pb(II) by magnetic activated carbon incorporated with amino groups from aqueous solutions, J. Taiwan Inst. Chem. Eng., 2016, 62, 247 CrossRef CAS.
- C. Michailof, G. G. Stavropoulos and C. Panayiotou, Enhanced adsorption of phenolic compounds, commonly encountered in olive mill wastewaters, on olive husk derived activated carbons, Bioresour. Technol., 2008, 99, 6400 CrossRef CAS PubMed.
- I. A. W. Tan, A. L. Ahmad and B. H. Hameed, Adsorption of basic dye on high-surface-area activated carbon prepared from coconut husk: equilibrium, kinetic and thermodynamic studies, J. Hazard. Mater., 2008, 154, 337 CrossRef CAS PubMed.
- J. V. Nabais, P. Carrott, M. M. L. Ribeiro Carrott, V. Luz and A. L. Ortiz, Influence of preparation conditions in the textural and chemical properties of activated carbons from a novel biomass precursor: the coffee endocarp, Bioresour. Technol., 2008, 99, 7224 CrossRef CAS PubMed.
- O. Pezoti Jr, A. L. Cazetta, I. P. A. F. Souza, K. C. Bedin, A. C. Martins, T. L. Silva and V. C. Almeida, Adsorption studies of methylene blue onto ZnCl2-activated carbon produced from buriti shells (Mauritia flexuosa L.), J. Ind. Eng. Chem., 2014, 20, 4401 CrossRef.
- A. M. M. Vargas, C. A. Garcia, E. M. Reis, E. Lenzi, W. F. Costa and V. C. Almeida, NaOH-activated carbon from flamboyant (Delonix regia) pods: optimization of preparation conditions using central composite rotatable design, Chem. Eng. J., 2010, 162, 43 CrossRef CAS.
- G. E. J. Poinern, G. Senanayake, N. Shah, X. Thi-Le, G. M. Parkinson and D. Fawcett, Adsorption of the aurocyanide, Au(CN)2− complex on granular activated carbons derived from macadamia nut shells – a preliminary study, Miner. Eng., 2011, 24, 1694 CrossRef CAS.
- Y. Li, X. F. Ding, Y. P. Guo, C. G. Rong, L. L. Wang, Y. N. Qu, X. Y. Ma and Z. C. Wang, A new method of comprehensive utilization of rice husk, J. Hazard. Mater., 2011, 186, 2151 CrossRef CAS PubMed.
- L. L. Ding, B. Zou, L. Shen, C. Zhao, Z. C. Wang, Y. P. Guo, X. F. Wang and Y. H. Liu, Adsorption of rhodamine-B from aqueous solution using treated rice husk-based activated carbon, Colloids Surf., A, 2014, 446, 90 CrossRef CAS.
- L. L. Wang, Z. B. Zhang, Y. N. Qu, Y. P. Guo, Z. C. Wang and X. F. Wang, A novel route for preparation of high-performance porous carbons from hydrochars by KOH activation, Colloids Surf., A, 2014, 447, 183 CrossRef CAS.
- Y. F. Shen, P. T. Zhao and Q. F. Shao, Porous silica and carbon derived materials from rice husk pyrolysis char, Microporous Mesoporous Mater., 2014, 188, 46 CrossRef CAS.
- B. Liu, J. Gu and J. B. Zhou, High surface area rice husk-based activated carbon prepared by chemical activation with ZnCl2–CuCl2 composite activator, Environ. Prog. Sustainable Energy, 2015, 35, 1 CrossRef.
- W. Y. Fan, M. He, X. R. Wu, B. B. Chen and B. Hu, Graphene oxide/polyethylene glycol composite coated stir bar for sorptive extraction of fluoroquinolones from chicken muscle and liver, J. Chromatogr. A, 2015, 1418, 36 CrossRef CAS PubMed.
- Y. Y. Sun, J. Tian, L. Wang, H. Y. Yan, F. X. Qiao and X. Q. Qiao, One pot synthesis of magnetic graphene/carbon nanotube composites as magnetic dispersive solid-phase extraction adsorbent for rapid determination of oxytetracycline in sewage water, J. Chromatogr. A, 2015, 1422, 53 CrossRef CAS PubMed.
- M. Uchimiya, L. H. Wartelle, K. T. Klasson, C. A. Fortier and I. M. Lima, Influence of pyrolysis temperature on biochar property and function as a heavy metal sorbent in soil, J. Agric. Food Chem., 2011, 59, 2501 CrossRef CAS PubMed.
- X. D. Zhu, Y. C. Liu, F. Qian, C. Zhou, S. C. Zhang and J. M. Chen, Preparation of magnetic porous carbon from waste hydrochar by simultaneous activation and magnetization for tetracycline removal, Bioresour. Technol., 2014, 154, 209 CrossRef CAS PubMed.
- Y. J. Ma, X. Zhao, X. Chen and Z. C. Wang, An approach to improve the application of acid-insoluble lignin from rice hull in phenol–formaldehyde resin, Colloids Surf., A, 2011, 377, 284 CrossRef CAS.
- A. C. Martins, O. Pezoti, A. L. Cazetta, K. C. Bedin, D. A. S. Yamazaki, G. F. G. Bandoch, T. Asefa, J. V. Visentainer and V. C. Almeida, Removal of tetracycline by NaOH-activated carbon produced from macadamia nut shells: kinetic and equilibrium studies, Chem. Eng. J., 2015, 260, 291 CrossRef CAS.
|
This journal is © The Royal Society of Chemistry 2016 |