DOI:
10.1039/C6RA23984F
(Paper)
RSC Adv., 2016,
6, 108180-108186
Insight into the synthesis of isosorbide diester plasticizer using immobilized lipases
Received
27th September 2016
, Accepted 5th November 2016
First published on 7th November 2016
Abstract
A bio-plasticizer isosorbide diester was synthesized using three immobilized lipases to select a suitable catalyst. These three immobilized lipases demonstrated an (R)-enantio-preference for synthesizing isosorbide monoesters with different MS
:
MR values. The S-monoester content was close to zero when the reaction was catalyzed by Yarrowia lipolytica lipase lip2 (Ylip2) or RM IM. Thus, the MS synthesis rate was the limiting step for diester production. To overcome this constraint, the catalysis condition was optimized by using mixture of Novo 435 & Ylip2, or Novo 435 & RM IM, or Ylip2 and Novo 435 sequentially, respectively. When the reaction was sequentially catalyzed by Ylip2 and Novo 435, the highest diester content was obtained (77.4%), which was 2.1- and 1.7-fold greater than the content obtained using either Ylip2 or Novo 435 alone, respectively.
I. Introduction
Poly(vinyl chloride) (PVC) is one of the most widely manufactured resins in the world. It is used in the production of a wide variety of products, including packing materials, building materials, toys, agriculture plastic films, and bags.1 Plasticizers have been compounded with PVC in order to improve the thermal and mechanical properties. As such, plasticizer has become one of the most important PVC additives.2,3 Many kinds of plasticizers have been produced and commercialized.4 For instance, phthalate plasticizers (e.g. dioctyl phthalate (DOP) and dibutyl phthalate (DBP)) are important in this regard as they have the desired combination of high performance and low cost.4 Given this, they occupy more than 90% of the plasticizers used in China.5 However, phthalate plasticizer toxicity is of considerable interest as it can leech into domestic food and waste.6,7 Moreover, the properties of PVC products and their biological effect can change when phthalate-based plasticizers migrate from PVC products to the environment.8 It should also be noted that phthalate plasticizers are petroleum-based products. Therefore, their use is becoming more and more limited, especially in products designed for using by children.9 Therefore, finding a bio-based plasticizer that is environmentally friendly and biodegradable with low toxicity is imperative, particularly as the consumption of petroleum resources and their cost increase.10
With this in mind, more and more attention has been paid to epoxidized vegetable oil, polyester, and triglyceride-based plasticizers.11–13 Recently, we explored the synthesis of isosorbide diester using Novo 435 and its potential application as a PVC plasticizer.14 In general, the epoxidized oil content in PVC products is between 5–10% of all plasticizers, leading to its status as a secondary plasticizer. It is advantageous that isosorbide diester could be used as the main plasticizer, giving isosorbide diester high prospects for its future application.
Lipases (glycerol ester hydrolase EC 3.1.1.3) are important industrial catalysts that have been widely used in hydrolysis, alcoholysis, esterification, and transfer action reactions.15,16 Lipases have excellent catalytic activities in non-aqueous media and their region- and enantio-selectivity can be used to obtain enantiopure chemicals.17,18 Previous studies have shown the enantioselective ability of many different kinds of lipases, including Candida antarctica lipase B,19 Novozym 435,20 Rhizomucor miehei lipase,21 and Lipase PS.22 Despite this, (3S,6R)-isosorbide has been shown to be a chiral compound, however there are no reports regarding its enantioselectivity in the process of synthesizing either isosorbide diester or isosorbide polymer.23 Lipases from different sources have different chiral preferences and selective activities.24 Different approaches have been used to improve and reverse enantioselectivity, such as co-solvent engineering,25 immobilization techniques,26 directed evolution,27 and regulating reaction conditions.28
Yarrowia lipolytica lipase Lip2 (Ylip2) is an extracellular lipase produced by the yeast Yarrowia lipolytica.15 It has been widely used as a catalyst for many reactions, including biodiesel production,29 modification of oils and fats, and enantioselection of ibuprofen and (R,S)-1-phenylethanol.25,30 One of its major advantages is its low cost.
This study sought to explore the synthesis of isosorbide ester from different and individually immobilized lipases obtained from Yarrowia lipolytica (Ylip2), Candida Antarctica (Novo 435), and Rhizomucor miehei (Lipozyme RM-IM), and their independent activities and selectivities. The effect of different immobilized lipases on the selectivity of isosorbide monoester and their acyl donor preferences were also examined. Finally, we investigated the catalytic efficiencies using simultaneous or sequential catalytic methods with the selected immobilized lipases.
II. Materials and methods
A. Materials
The catalyst Novozym 435 (Novo 435) and RM IM were purchased from Beijing Cliscent Technology Co., Ltd, Beijing, China. Yarrowia lipolytica lipase (Ylip2) was purchased from Beijing CTA New Century Biotechnology Co., Ltd, Beijing, China. Immobilized Ylip2 was produced according to our previously published research.31 Isosorbide (98% purity) was obtained from TCI (Shanghai) Development Chemical Industry Co., Ltd, Shanghai, China. Caprylic acid, capric acid, lauric acid, myristic acid, palmitic acid, oleic acid, and dodecanol were all 98% pure and were obtained from Fuchen Chemical Co., Ltd. (Tianjin, China). Celite was purchased from Changbai Celite Co., Ltd, Jilin, China. All other reagents and solvents used were of analytical grade.
B. Isosorbide diester synthesis using different immobilized lipases
All experiments were carried out in a 100 mL, two-neck flask. We first sought to examine the effect of three different immobilized lipases on esterification according to the following design: isosorbide (4.05 g) and oleic acid (19.56 g) were individually combined with Ylip2 (0.81 g), Novo 435 (0.19 g), or RM IM (0.24 g). All the reactions were conducted over 24 h at 50 °C, followed by a centrifugation at 300 rpm. And the reaction route is shown in Fig. 1.
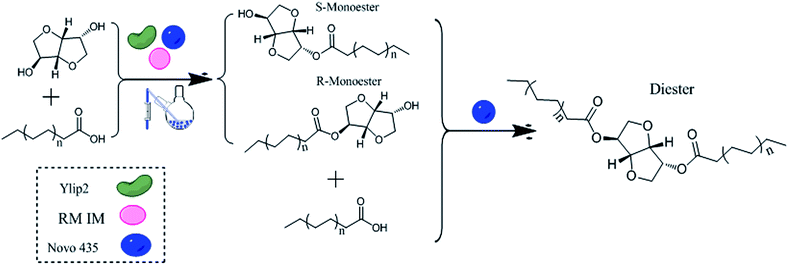 |
| Fig. 1 The isosorbide diester synthesis route using immobilized lipases. | |
The reaction conditions for the esterification of isosorbide with different acyl donors were as follows: isosorbide to acyl donor molar ratio was 1
:
2.5 (4.05 g isosorbide, 9.98 g caprylic acid, 10.95 g nonoic acid, 11.93 g capric acid, 13.87 g lauric acid, and 9.56 g oleic acid), and the immobilized lipases had equal esterification activities (0.81 g Ylip2, 0.19 g Novo 435, and 0.24 g RM IM). Reactions were conducted with bubbling dried air at 50 °C, followed by a centrifugation at 300 rpm for 12 h.
For the hydrolysis experiments, esterification products were reacted with water under optimized esterification conditions14 with diester (29.3 g), water (3 g), and Novozym 435 (1.97 g), followed by a centrifugation at 300 rpm for 105 min at 60 °C.
Reactions using immobilized lipase mixtures were carried out with different lipase ratios. The conditions were as follows: substrates molar ratio of 1
:
2.5 (isosorbide
:
acid), temperature of 55 °C, and biocatalyst esterification activity ratios of 3
:
1, 2
:
1, 1
:
1, 1
:
2, and 1
:
3. All reactions were carried out for 36 h.
The sequential catalysis reaction conditions using Ylip2 and Novo 435 were as follows: isosorbide (4.05 g) and oleic acid (19.56 g) were reacted in the presence of Ylip2 at 50 °C and centrifuged at 300 rpm for 24 h. Ylip2 was then removed by further centrifugation, after which the medium was catalyzed by Novo 435 at 60 °C and centrifuged at 300 rpm for 24 h.
The acid, monoester, and diester contents were then analyzed using gas chromatography (GC).
C. Hydrolysis and esterification activities of immobilized lipases
(i) Hydrolysis. The hydrolysis activities of free and immobilized lipases were measured via olive oil hydrolysis according to a previously published report.31 Briefly, an olive oil emulsion (200 mL) was prepared by mixing olive oil (50 mL) and 3% PVA (150 mL) under vigorous stirring for 6 min. Then, 5 mL of this emulsion and 4 mL of 0.1 M phosphate buffer (pH 8.0) were combined and warmed at 35 °C for 5 min. Immobilized lipase was then added to the mixture and incubated at 35 °C before being agitated at 100 rpm for 5 min in a water bath shaker. The reaction was terminated by addition of 15 mL of ethanol. The amount of fatty acid released was then determined by titration with 50 mM NaOH solution. Following convention, one unit of lipase hydrolytic activity was defined as the release of 1 μmol of fatty acids per min at 35 °C. The results shown are the averages of experiments conducted in triplicate.
(ii) Esterification activity. The esterification activities of different immobilized lipases were measured using lauric acid and dodecanol. The standard esterification procedure was used as follows: immobilized lipase was added into a 50 mL conical flask containing a mixture of 200 mM dodecanoic acid and 200 mM dodecanol in 5 mL of hexane. The flask was then placed in a temperature controlled shaker and shaken at 180 rpm for 15 min at 40 °C. The esterification activity was quantified using GC and one unit of lipase esterification activity was defined as the consumption of 1 μmol of fatty acid per min at 40 °C for the immobilized lipase.
D. GC analysis
(i) Analysis of isosorbide diester by GC. Aliquots were taken from the reactions at each scheduled time point and immediately heated to ensure enzyme deactivation. The levels of fatty acid, monoester (S-monoester, MS and R-monoester, MR), and di-substituted esters in the reaction mixture were quantified using a Trace 1300 gas chromatograph (Thermo scientific, USA) equipped with a DB-1ht capillary column (30 m × 0.25 mm × 0.1 mm; J&W Scientific, USA) and a flame ionizing detector (FID).14 The column temperature was held at 120 °C and then ramped to 190 °C at 30 °C min−1 and to 360 °C at 45 °C min−1, where it was held for 10 min. The temperatures of the injector and detector were both set at 360 °C. The molar fractions of fatty acid, isosorbide, monoester, and diester were then calculated.
(ii) Analysis of esterification activity by GC. During the esterification process, the initial injector temperature was 100 °C, which was then increased to 300 °C at a heating rate of 200 °C min−1 and maintained for 4.5 min. The initial column oven temperature was 120 °C, which was first increased to 132 °C at a heating rate of 12 °C min−1, then increased to 300 °C at a heating rate of 45 °C min−1, and finally maintained at the maximum temperature for 2 min. The temperature of both the injector and detector was 300 °C.
III. Results and discussions
A. Effect of different immobilized lipases on synthesizing isosorbide diester
Three immobilized lipases (Ylip2, Novo 435, and RM IM) were used to synthesize isosorbide diester (Fig. 1). In the esterification, the enzyme loading amounts have a positive influence on ester content and reaction rate. Thus, the universal standard was necessary when comparing the effect of different immobilized lipases on synthesizing isosorbide diester.
As shown in Table 1, the immobilized lipases exhibited different hydrolysis and esterification activities. Ylip2 obtained the highest hydrolysis activity (17
000 U g−1), which was 1.7- and 2.1-fold higher than either Novo 435 (10
000 U g−1) or RM IM (8000 U g−1), respectively. However, Novo 435 (949.9 U g−1) obtained the highest esterification activity, which was 3.7- and 1.2-fold higher than either Ylip2 (253.3 U g−1) or RM IM (785.2 U g−1), respectively.
Table 1 Effect of three different immobilized lipases (Ylip2, Novo 435 and RM IM) on lipase properties and isosorbide diester content. Reaction conditions: 4.05 g isosorbide, 19.56 g oleic acid, and immobilized lipases with equal esterification activities (180 U). Reactions were conducted with bubbling dried air at 50 °C, followed by a centrifugation at 300 rpm for 48 h
Immobilized lipase |
Hydrolysis activity (U g−1) |
Esterification activity (U g−1) |
Content (%) |
Acid |
MS |
MR |
Diester |
Ylip2 |
17 000 |
253.3 |
56.91 |
1.05 |
37.36 |
4.68 |
Novo 435 |
10 000 |
949.9 |
55 |
6.48 |
18.86 |
19.65 |
RM IM |
8000 |
785.2 |
57.71 |
0.25 |
40.34 |
1.69 |
Since isosorbide diester was synthesized using an esterification reaction, equal esterification activity was chosen as the loading amount of the three different immobilized lipases for isosorbide diester synthesis. As shown in Table 1, the acid contents were approximately the same at 24 h after the start of the reaction. This shows that the catalytic efficiencies of the immobilized lipases were the same when catalyzing fatty acids with equal esterification activities. However, Novo 435 (19.65%) was more effective than either Ylip2 (4.68%) or RM IM (1.69%) when synthesizing diesters. The monoester content (S- and R-monoesters) was approximately 40% for both Ylip2 and RM IM and 25.34% for Novo 435. It was also found that the R-monoester (MR) content was much higher than the S-monoester (MS) content regardless of the immobilized lipase used. The MR and MS contents were 37.36 and 1.05, 18.86 and 6.48, and 40.34 and 0.25 for Ylip2, Novo 435 and RM IM, respectively. These results illustrate that the three immobilized lipases preferentially catalyzed the R-monoester, especially Ylip2 and RM IM. Diester was produced from fatty acids and monoesters, and the higher MR/MS ratio when using Ylip2 and RM IM limited the isosorbide diester synthesis. Thus, Novo 435 obtained the highest diester content.
B. Effect of different acyl donors on monoester and diester content
In esterification reactions, the acyl donor leads to the formation of an acyl-lipase intermediate, followed by nucleophilic attack alcohol, resulting in the product formation and release of the lipase.32 Thus, the acylation process is very important for esterification reactions. Different fatty acids were chosen as the acyl donors for isosorbide diester synthesis using Novo 435, RM IM, and Ylip2. As shown in Fig. 2A, the diester content was higher when the reaction was catalyzed by Novo 435 than by RM IM or Ylip2. This occurred when the acyl donor was caprylic acid, nonoic acid, capric acid, lauric acid, or oleic acid. The diester content was close to zero when RM IM was used. The diester content was also close to zero when Ylip2 was used, with the exception of oleic acid. Given these results, it is clear that the fatty acid preferences for reaction were different. Novo 435 preferred mid-length fatty acid chains, while Ylip2 preferred long-chain fatty acids. As shown in Fig. 2B, the monoester content derived from both RM IM and Ylip2 varied greatly depending on the type of acyl donor, however, both RM IM and Ylip2 preferred lauric acid and oleic acid. The changes of the monoester content with these preferred acyl donors were consistent with those of the diester content.
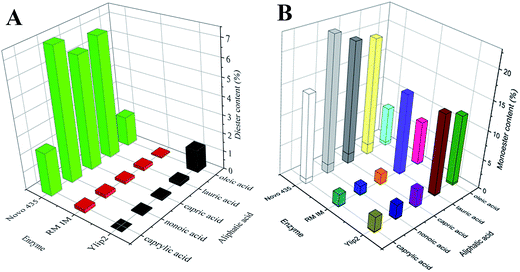 |
| Fig. 2 (A) Effects of acyl donor and immobilized lipases on diester content. (B) Effects of acyl donor and immobilized lipases on monoester content. Reaction conditions: isosorbide to acyl donor molar ratio was 1 : 2.5 (4.05 g isosorbide, 9.98 g caprylic acid, 10.95 g nonoic acid, 11.93 g capric acid, 13.87 g lauric acid, and 19.56 g oleic acid), and immobilized lipases with equal esterification activities (0.81 g Ylip2, 0.19 g 435, and 0.24 g RMIM). Reactions were conducted with bubbling dried air at 50 °C followed by a centrifugation at 300 rpm for 12 h. | |
In addition, the monoester content included both MS and MR. As shown in Table 2, the monoester and diester contents increased as the reaction time increased. However, the MS content was much lower than the MR content; thus, diester production was limited by MS synthesis. When diester was synthesized using Ylip2 or Novo 435, the MR
:
MS values were 40 and 18, respectively, which indicated that the Ylip2- and Novo 435-driven MR synthesis rates were 40- and 18-fold greater than the corresponding MS synthesis rates, respectively. Furthermore, this illustrated that the selectivity of Ylip2 and Novo 435 was maintained as the reaction time increased. However, the MR
:
MS values increased from 54.60 to 82.44 when the reaction time increased from 2 h to 8 h for RM IM diester synthesis. Collectively, these results demonstrate that RM IM, Ylip2, and Novo 435 favor the formation of (R)-isosorbide. This was especially true for RM IM lipase.
Table 2 Effect of different lipases and reaction time on the compounds contents when synthesizing isosorbide diester. Reaction conditions: 4.05 g isosorbide, 19.56 g oleic acid, and immobilized lipases with equal esterification activities (180 U). Reactions were conducted with bubbling dried air at 50 °C followed by a centrifugation at 300 rpm
Immobilized lipase |
Reaction time (h) |
Content (%) |
MR : MS |
Acid |
MS |
MR |
Diester |
Ylip2 |
2 |
97.65 |
0.06 |
2.23 |
0.06 |
37.17 |
4.5 |
94.62 |
0.11 |
5.04 |
0.23 |
45.82 |
6 |
92.82 |
0.16 |
6.68 |
0.34 |
41.75 |
8 |
91.31 |
0.19 |
8.12 |
0.38 |
42.74 |
Novo 435 |
2 |
97.64 |
0.11 |
2.13 |
0.11 |
19.37 |
4.5 |
92.98 |
0.31 |
6.04 |
0.67 |
19.48 |
6 |
89.99 |
0.48 |
8.29 |
1.24 |
17.27 |
8 |
87.1 |
0.64 |
10.56 |
1.7 |
16.50 |
RM IM |
2 |
97.23 |
0.05 |
2.73 |
0 |
54.60 |
4.5 |
91.56 |
0.11 |
8.28 |
0.05 |
75.27 |
6 |
89.24 |
0.12 |
10.59 |
0.06 |
88.25 |
8 |
86.55 |
0.16 |
13.19 |
0.09 |
82.44 |
C. Effect of diester hydrolysis and esterification
To further analyze the synthesis of diester, the esterification and hydrolysis of isosorbide diester were estimated by Novo 435. Fig. 3A shows the isosorbide diester synthesis process, where the bell-type curve of the monoester indicates that the diester was generated in a step-wise process. However, the MR curve was consistent with that of the monoester, while the MS content increased slowly before achieving equilibrium as the reaction time increased. During diester hydrolysis (Fig. 3B), the diester content decreased quickly due to the conversion to MS, and the MS content was almost equal to the monoester content. The MR content was closed to zero, illustrating that MR was quickly hydrolyzed to acid. Thus, the esterification and hydrolysis process of isosorbide diester showed that the MS synthesis rate, but not the MR synthesis rate, was the limiting step for diester production. To our knowledge, this is the first report about lipase-catalyzed isosorbide transformation.
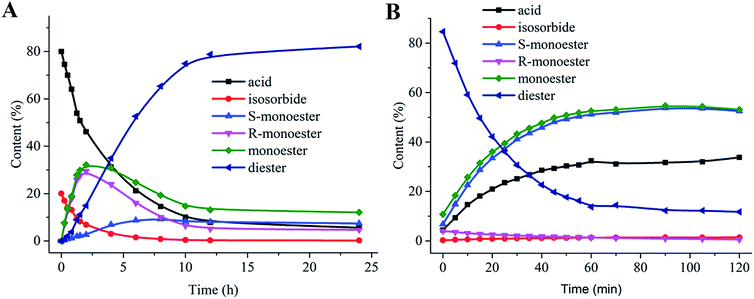 |
| Fig. 3 (A) Effects of esterification on the medium compounds using Novo 435. (B) Effects of isosorbide diester hydrolysis reaction on the medium compounds using Novo 435. Esterification reaction conditions: 4.05 g isosorbide, 19.56 g oleic acid, and 1.01 g Novo 435 at 60 °C. Hydrolysis reaction conditions: 23 g esterification medium (4.39% oleic acid, 24% isosorbide, 10.77% monoester, and 84.6% diester), 20 g water, and 1.01 g Novo 435 at 60 °C. | |
D. Effect of the mixed immobilized lipases ratios on monoester and diester content
Lipases from different sources have different chiral preferences and selectivities. In this work, Novo 435 was more suitable for synthesizing MS, while both Ylip2 and RM IM were more preferable for MR synthesis. Thus, the mixture of two lipases with different specificities may increase the diester synthesis rate as compared to a reaction containing just one.33,34 Furthermore, the mixture of two lipases could be an economically available alternative when compared to individual applications, as both RM IM and Ylip2 are cheaper than Novo 435. This difference in cost is especially salient for Ylip2. To further examine their individual advantages, mixtures of Novo 435 & Ylip2 and Novo 435 & RM IM were tested using different esterification activity ratios (3
:
1, 2
:
1, 1
:
1, 1
:
2, or 1
:
3).
As shown in Fig. 4A and B, the diester content increased from 30% to 45% while the monoester content decreased from 21% to 15%, these changes occurred as the esterification activity ratio of Novo 435 to Ylip2 was varied from 1
:
3 to 1
:
1. The ratio was further increased to 3
:
1, with the diester content maintained at 49% and the monoester content maintained at 14%. The highest monoester content was approximately 30%, which occurred even with different immobilized lipase ratios. Thus, the optimized esterification ratio for Ylip2 and Novo 435 was 1
:
1. Similar results were observed when RM IM and Novo 435 mixtures were used to synthesize isosorbide diester. There were still some differences. The monoester content decreased slowly after it peaked and the diester content increased slowly when the ratio of Novo 435 to RM IM was 1
:
2 and 1
:
3. The optimized esterification ratio of RM IM and Novo 435 was 1
:
1. Thus, the synergistic effect of mixtures of Ylip2 or RM IM and Novo 435 for esterification was 1
:
1.
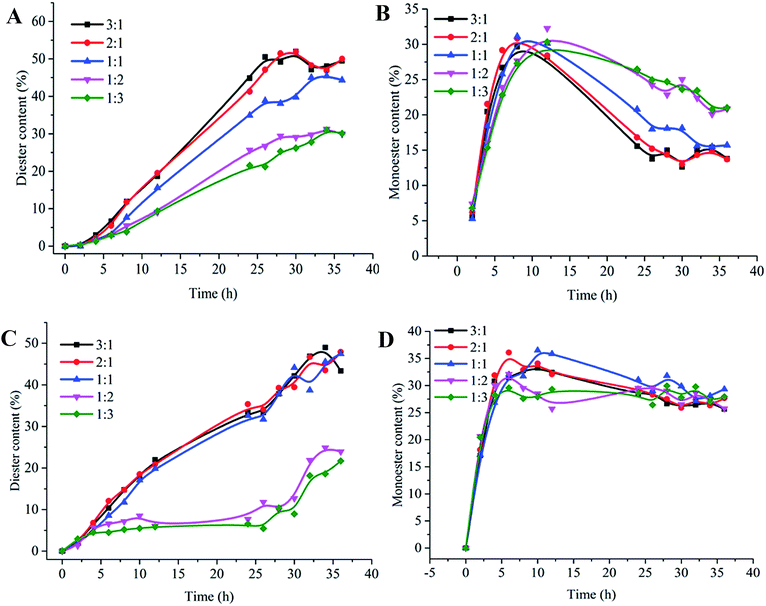 |
| Fig. 4 (A) Effects of Novo 435 and Ylip2 esterification activity ratios on diester content. (B) Effects of Novo 435 and Ylip2 esterification activity ratios on monoester content. (C) Effects of Novo 435 and RM IM esterification activity ratios on diester content. (D) Effects of Novo 435 and RM IM esterification activity ratios on monoester content. Reaction conditions: 4.05 g isosorbide, 19.56 g oleic acid, and 550 U esterification activity of the immobilized lipase at 60 °C. | |
E. Effect of the catalytic sequence of immobilized lipases ratios on monoester and diester content
Finally, we tested the effects of mixtures of immobilized lipases on isosorbide diester synthesis. Since Ylip2 is more suitable for industrial applications due to its low cost, we selected it along with Novo 435 for diester synthesis. However, there were differences in the optimized, catalyzed reactions. If Ylip2 and Novo 435 were simultaneously used to catalyze the reaction, it is possible they might not function at the most suitable conditions. To overcome this limitation, Ylip2 and Novo 435 were used sequentially for diester synthesis. This sequence was chosen because Ylip2 only catalyzed MR synthesis while Novo 435 catalyzed both MS and MR synthesis. Therefore, Ylip2 was first used to synthesize MR, followed by Novo 435 for diester synthesis.
As shown in Fig. 5A, when the reaction was first catalyzed by Ylip2, monoester content was 43.02% (MS: 1.52%, MR: 41.58%) and diester content was 5.44% after 24 h. Comparatively, continuous catalysis with Novo 435 resulted in a monoester content of 12.13% (MS: 8.07%, MR: 4.06%) and a diester content of 77.74% after 24 h. The monoester and diester contents were 37.4% and 24.74% and 20.55% and 44.58%, respectively, when the reaction was catalyzed by Ylip2 and Novo 435 for 48 h (Fig. 5B). The diester content resulted from sequential catalysis was higher than that resulted from mixtures or single immobilized lipase catalysis. This is due to the higher monoester content resulted from sequential catalysis versus mixture or single immobilized lipases. In summary, monoester content is significantly important for diester synthesis and the synthesis of high-content monoesters was beneficial for isosorbide diester synthesis.
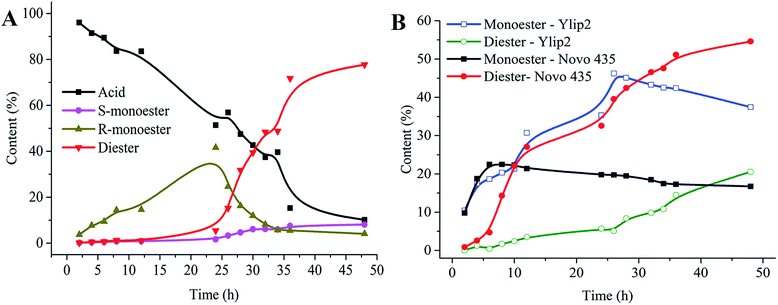 |
| Fig. 5 (A) Effects of Ylip2 and Novo 435 sequentially catalyzed the diester synthesis on the reaction compounds contents. (B) Effects of Ylip2 and Novo 435 individually catalyzed the diester synthesis on the reaction compounds contents. | |
IV. Conclusions
In this study, the bio-plasticizer isosorbide diester was synthesized using three different immobilized lipases, Ylip2, RM IM and Novo 435 to determine which one would be the most suitable catalyst. Lipase sources and acyl donors significantly affected mono- and diester synthesis efficiencies. These three lipases were preferable for MR formation, especially Ylip2 and RM IM. The synthesis rate of MS was the limiting step for diester production. We utilized two strategies to overcome these drawbacks: (1) allowing for each advantage to be highlighted and (2) maintaining a low cost. The catalysis condition was optimized by using mixture of Novo 435 & Ylip2 or Novo 435 & RM IM or Ylip2 and Novo 435 sequentially. When the reaction was sequentially catalyzed by Ylip2 and Novo 435, the highest diester content was obtained (77.4%), which was 2.1- and 1.7-fold greater than the content obtained using either Ylip2 or Novo 435 alone, respectively.
Acknowledgements
This work was supported by the National Basic Research Program of China (973 program) (2013CB733600, 2012CB725200), the National Nature Science Foundation of China (21576019, 21436002), National High-Tech R&D Program of China (863 Program) (2014AA022100), and the Higher Education and High-quality and World-class Universities (PY201614).
Notes and references
- G. V. Rybachuk, I. I. Kozlova, V. B. Mozzhukhin and V. V. Guzeev, Polym. Sci. Ser. C., 2007, 49, 6–12 CrossRef.
- J. T. van Oosterhout and M. Gilbert, Polymer, 2003, 44, 8081–8094 CrossRef CAS.
- B. L. Shah and V. V. Shertukde, J. Appl. Polym. Sci., 2003, 90, 3278–3284 CrossRef CAS.
- A. Stuart, M. M. Mccallum, D. Fan, D. J. Lecaptain, C. Y. Lee and D. K. Mohanty, Polym. Bull., 2010, 65, 589–598 CrossRef CAS.
- A. Zoller and A. Marcilla, J. Appl. Polym. Sci., 2012, 124, 2691–2701 CrossRef CAS.
- Y. Yue, J. Liu, L. Ren, Y. Sun, X. Li and F. Jing, Food Chem. Toxicol., 2014, 71, 244–253 CrossRef CAS PubMed.
- F. Chiellini, M. Ferri, A. Morelli, L. Dipaola and G. Latini, Prog. Polym. Sci., 2013, 38, 1067–1088 CrossRef CAS.
- Y. Liu, S. Wang and L. Wang, J. Agric. Food Chem., 2013, 61, 1160–1164 CrossRef CAS PubMed.
- A. Greco, D. Brunetti, G. Renna, G. Mele and A. Maffezzoli, Polym. Degrad. Stab., 2010, 95, 2169–2174 CrossRef CAS.
- P. Jia, M. Zhang, L. Hu, G. Feng, C. Bo and Y. Zhou, ACS Sustainable Chem. Eng., 2015, 3, 2187–2193 CrossRef CAS.
- J. Chen, Z. Liu, J. Jiang, X. Nie, Y. Zhou and R. E. Murray, RSC Adv., 2015, 5, 56171–56180 RSC.
- J. Ye, S. Liu, J. Xiang, J. Lei and C. Zhou, J. Appl. Polym. Sci., 2013, 129, 1915–1921 CrossRef CAS.
- P. Jia, M. Zhang, L. Hu and Y. Zhou, Pol. J. Chem. Technol., 2016, 18, 9–14 CAS.
- C. Cui, Y. Zhen, J. Qu, B. Chen and T. Tan, RSC Adv., 2016, 6, 11959–11966 RSC.
- C. Cui, Y. Tao, L. Li, B. Chen and T. Tan, J. Mol. Catal. B: Enzym., 2013, 91, 59–66 CrossRef CAS.
- X. L. Wu, M. Hou and J. Ge, Catal. Sci. Technol., 2015, 5, 5077–5085 CAS.
- V. Gotor-Fernandez, P. Fernandez-Torres and V. Gotor, Tetrahedron: Asymmetry, 2006, 17, 2558–2564 CrossRef CAS.
- R. A. Sheldon and S. van Pelt, Chem. Soc. Rev., 2013, 42, 6223–6235 RSC.
- W. Y. Lou, M. H. Zong, Y. Y. Liu and J. F. Wang, J. Biotechnol., 2006, 125, 64–74 CrossRef CAS PubMed.
- X. Wu, Y. Wang, J. Ju, C. Chen, N. Liu and Y. Chen, Tetrahedron: Asymmetry, 2009, 20, 2504–2509 CrossRef CAS.
- Y. F. Zhang, J. Ge and Z. Liu, ACS Catal., 2015, 5, 4503–4513 CrossRef.
- S. Kataoka, Y. Takeuchi, A. Harada, M. Yamada and A. Endo, Green Chem., 2010, 12, 331–337 RSC.
- D. Juais, A. F. Naves, C. Li, R. A. Gross and L. H. Catalani, Macromolecules, 2010, 43, 10315–10319 CrossRef CAS.
- D. Yıldırım and S. S. Tükel, Biocatal. Biotransform., 2014, 32, 251–258 CrossRef.
- C. Cui, R. Xie, Y. Tao, Q. Zeng and B. Chen, Biocatal. Biotransform., 2015, 33, 38–43 CrossRef CAS.
- J. C. J. Quilles, R. R. Brito, J. P. Borges, C. C. Aragon, G. Fernandez-Lorente, D. A. Bocchini-Martins, E. Gomes, R. D. Silva, M. Boscolo and J. M. Guisan, Biochem. Eng. J., 2015, 93, 274–280 CrossRef CAS.
- L. F. Godinho, C. R. Reis, H. J. Rozeboom, F. J. Dekker, B. W. Dijkstra, G. J. Poelarends and W. J. Quax, J. Biotechnol., 2012, 158, 36–43 CrossRef CAS PubMed.
- G. Fernández-Lorente, J. M. Palomo, C. Mateo, J. M. Guisan and R. Fernandez-Lafuente, Enzyme Microb. Technol., 2004, 34, 264–269 CrossRef.
- T. Tan, J. Lu, K. Nie, L. Deng and F. Wang, Biotechnol. Adv., 2010, 28, 628–634 CrossRef CAS PubMed.
- Y. Liu, F. Wang and T. Tan, J. Mol. Catal. B: Enzym., 2009, 56, 126–130 CrossRef CAS.
- C. Cui, N. Guan, C. Xing, B. Chen and T. Tan, Colloids Surf., B, 2016, 146, 490–497 CrossRef CAS PubMed.
- M. M. Zheng, L. Wang, F. H. Huang, L. Dong, P. M. Guo, Q. C. Deng, W. L. Li and C. Zheng, Ultrason. Sonochem., 2012, 19, 1015–1020 CrossRef CAS PubMed.
- L. Li, W. Du, D. Liu, L. Wang and Z. Li, J. Mol. Catal. B: Enzym., 2007, 43, 18 Search PubMed.
- J. Alves, N. Vieira, A. Cunha, A. Silva, M. Zachiaayub, R. Fernandez-Lafuente and R. Rodrigues, RSC Adv., 2014, 4, 6863–6868 RSC.
Footnote |
† Equal contributors. |
|
This journal is © The Royal Society of Chemistry 2016 |
Click here to see how this site uses Cookies. View our privacy policy here.