DOI:
10.1039/C6RA22942E
(Paper)
RSC Adv., 2016,
6, 103556-103566
A recyclable and water soluble copper(I)-catalyst: one-pot synthesis of 1,4-disubstituted 1,2,3-triazoles and their biological evaluation†
Received
14th September 2016
, Accepted 17th October 2016
First published on 25th October 2016
Abstract
A facile protocol for the regioselective synthesis of 1,4-disubstituted 1,2,3-triazoles via a three-component ‘click’ reaction of alkyl/benzyl bromides, sodium azide, and terminal alkynes catalyzed by an efficient water soluble copper(I) complex has been developed. The halides have been directly converted into 1,2,3-triazoles via in situ generation of azides and hence, handling of hazardous azide intermediates can be avoided. Gratifyingly, the inherent advantages of this one-pot process are the use of water as solvent, catalyst recyclability, reduced reaction times, simple recrystallization and high yields of the products. The broad scope of this protocol was also explored for the synthesis of a variety of diversely substituted biologically relevant DNA-interactive 1,2,3-triazolo-tetrahydro-β-carboline derivatives. These 1,2,3-triazolo-tetrahydro-β-carbolines were further evaluated for their in vitro cytotoxicity on selected human cancer cell lines of A-549, HCT-116, BT-549, MDA-MB-231, PC-3, NCI-H460 and HCT-15. DNA binding affinity through viscometry experiment and molecular modeling studies have indicated efficient minor groove binding of these new scaffolds.
Introduction
In modern drug discovery, a large number of lead molecules need to be generated in a short period of time using efficient strategies. The copper(I)-catalyzed 1,3-dipolar cycloaddition of azides and alkynes (CuAAC) or ‘click’ reaction is one such strategy for the rapid conjugation of molecular fragments through 1,2,3-triazoles under mild reaction conditions.1 Applications of click chemistry in drug discovery2 range from lead discovery and optimization through combinatorial and target-templated in vitro chemistry,3 to proteomics4 and DNA research.5 Though, the 1,2,3-triazole moiety does not occur in nature, the synthetic derivatives possessing this moiety exhibit diverse range of biological activities by readily associating with biological targets, through hydrogen-bonding and dipole interactions.6 The 1,2,3-triazoles are more than just passive linkers; they are stable to metabolic degradation and can also improve the solubility.7 Despite the numerous advantages of the CuAAC reactions, the organic azides used therein are highly hazardous in nature and, thus, their handling is very risky.8 Thus, a one-pot procedure for the synthesis of triazoles involving the reaction of azides, generated in situ from the corresponding halides, and alkynes is highly desirable. A number of such one-pot processes catalyzed by a variety of heterogeneously supported copper(I) catalysts have been reported.9 While these methods have certain advantages, synthesis of the catalysts is difficult, expensive and/or involve longer reaction times. So, there is a great need for the rapid development of efficient catalysts from cheaper and more readily available starting materials.
Even though, solvent free conditions are sought-after in terms of green chemistry, solid substrates and high exothermicity of the cycloaddition reaction, warrant the use of water as solvent.10 While, Cu(I) halides are popular catalysts in organic synthesis, their poor water solubility can interfere with the catalytic activity and reproducibility of the results. In order to overcome the drawbacks of Cu(I) halides, we have identified an isolable and stable Cu(I)-complex i.e., [bis[(tetrabutylammonium)di-μ-iodo-diiododicuprate(I)], with improved solubility in water and organic solvents.11 Recently, we have explored the utility of this Cu(I)-complex for the greener synthesis of α-ketoamides.12
Exploring the interaction of small molecules with DNA has become an active research area in drug discovery due to their potential as therapeutic agents against numerous diseases.13 DNA groove binders act by inhibiting nucleic acid or protein synthesis through non-covalent and/or covalent interactions in either the minor or major groove of the double helix, without causing any major distortion of the DNA backbone.14 Many β-carboline analogues were reported to display DNA binding, resulting in antitumor activity.15 They are a class of indole alkaloids originally isolated from the plant Peganum harmala. Thus, in continuation of our earlier efforts in the field of anti-cancer β-carbolines and their novel synthetic strategies,16 we have attempted to develop a green protocol for their 1,2,3-triazole ligation, aiming to improve their DNA-binding and cytotoxic activities. Herein, we report a novel, simple and efficient Cu(I)-catalyzed one-pot, three-component 1,3-dipolar cycloaddition of alkyl/benzyl bromides, sodium azide, and alkynes for the regioselective synthesis of 1,4-disubstituted 1,2,3-triazoles in water. Further, we have also applied this protocol for the synthesis of a variety of diversely substituted DNA-interactive 1,2,3-triazolo-tetrahydro-β-carbolines possessing anti-cancer activity. We have investigated the binding mode of these compounds with DNA through viscosity measurements and molecular modelling. To the best of our knowledge, this is the first report on the synthesis of 1,2,3-triazolo-tetrahydro-β-carbolines as new pharmacophoric scaffolds.
Results and discussion
Initially, the bis[(tetrabutylammonium)di-μ-iodo-diiododicuprate(I)] complex was synthesized from CuI (copper iodide) and TBAI (n-tetrabutylammonium iodide). Next, benzyl bromide (1a), sodium azide, and phenyl acetylene (2a) were used as model substrates to examine the catalytic activity of the synthesized complex under different reaction conditions (Table 1). The air and moisture stability of the catalyst allows the reactions to be performed in water under air atmosphere, without the concern of either oxidation or disproportionation generally observed with Cu(I) catalysts. A series of experiments were carried out by varying the solvents, amount of catalyst, temperature and time. The results are summarized in Table 1. Interestingly, the catalyst exhibited significant activity, particularly when water was used as a reaction media; the best yield (97%, Table 1, entry 7) was obtained at room temperature in 4 h in the presence of 2 mol% of catalyst.
Table 1 Screening of the ‘click’ reaction conditionsa
The solvents MeOH, EtOH and CH3CN gave poor to moderate reaction yields (41, 24 and 36% respectively, Table 1, entries 1–3); while no product was formed in DMF and THF (Table 1, entries 4 and 5 respectively). However, the H2O
:
tert-BuOH (4
:
1) solvent system also gave significant yield of 3a (80%, entry 6, Table 1) with 2 mol% of catalyst. It took longer for completion of the reaction (8 h) when only 1.0 mol% of the catalyst was used (Table 1, entry 9). In fact, the reaction was fairly efficient at elevated temperature of 60 °C in water, with a catalyst loading of only 1.0 mol% (93%, Table 1, entry 10). It is important to note that the reaction did not proceed in the absence of catalyst (entry 11, Table 1). Finally, the best yields were obtained when equimolar quantities of benzyl bromide, phenyl acetylene, and sodium azide in the presence of the Cu(I)-catalyst (2 mol%) are stirred at room temperature for 4 h in water. After completion of the reaction (monitored by TLC), the reaction mixture was extracted with ethyl acetate, concentrated and the product was recrystallized in 97% yield.
A wide range of diversely substituted alkynes 2a–e were reacted with a mixture of alkyl/benzyl bromides 1a–f and sodium azide by using this optimized protocol to produce the corresponding 1,4-disubstituted-1,2,3-triazoles (Table 2). The substitution on alkyne or alkyl/benzyl bromide did not have any appreciable influence on the outcome of the reaction (Table 2, entries 3a–m). The reaction proceeded uniformly for unsubstituted, ortho- and para-substituted alkynes to yield the final products. Phenylacetylene (2a), N-linked (2b) and O-linked (2c–e) alkynes reacted remarkably well to furnish the 1,2,3-triazoles in good yields. In almost all the cases, the reactions smoothly proceeded within 4 h and the products were isolated in excellent yields with significant purity. Moreover, this methodology is highly compatible with a variety of functional groups, such as fluoro, nitro, cyano, methoxy, allyl and ester as shown in Tables 2 and 3. Further, the scope of the reaction has also been successfully extended to bromides such as methyl bromoacetate (1d) and phenacyl bromide (1e). Unfortunately, aliphatic halogenated substrates i.e., bromoalkanes such as 1-bromobutane, 1-bromooctane; aromatic halides such as bromobenzene and chlorobenzene failed to undergo this one-pot reaction to produce the corresponding 1,2,3-triazoles. This may be attributed to the difficulty in formation of the corresponding organic azide under the optimized reaction conditions. However, the substrate scope can be extended to alkyl bromides by heating the reaction mixture; for example, when the reaction of naphthalimide linked alkyl bromide (1f) with phenylacetylene and sodium azide was heated at 60 °C, the corresponding triazole (3m) was obtained in good yield. Additionally, unreactive alkynes such as 1-hexyne, 1-heptyne and 1-octyne did not furnish the corresponding triazoles.
Table 2 Bis[(tetrabutylammonium)di-μ-iodo-diiododicuprate(I)]-catalyzed one-pot reaction of alkyl/benzyl bromides with terminal alkynes and sodium azidea
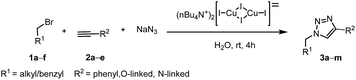
|
The reaction was performed with alkyl/benzyl bromides (1, 1.0 mmol), sodium azide (1.0 mmol), alkynes (2, 1.0 mmol), in the presence of Cu(I)-catalyst (2 mol%) in water. Isolated yields. Reaction was carried out at 60 °C for 4 h. |
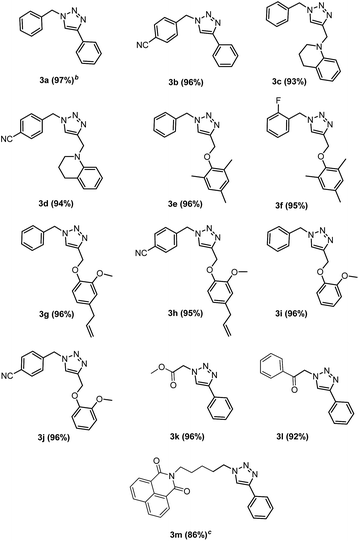 |
Table 3 Bis[(tetrabutylammonium)di-μ-iodo-diiododicuprate(I)]-catalyzed one-pot reaction of benzyl halides with N-propargylated tetrahydro-β-carbolines and sodium azidea

|
The reaction was performed with benzyl bromides (1, 1.0 mmol), sodium azide (1.0 mmol), alkynes (4, 1.0 mmol), in the presence of Cu(I)-catalyst (2 mol%) in water. Isolated yields. |
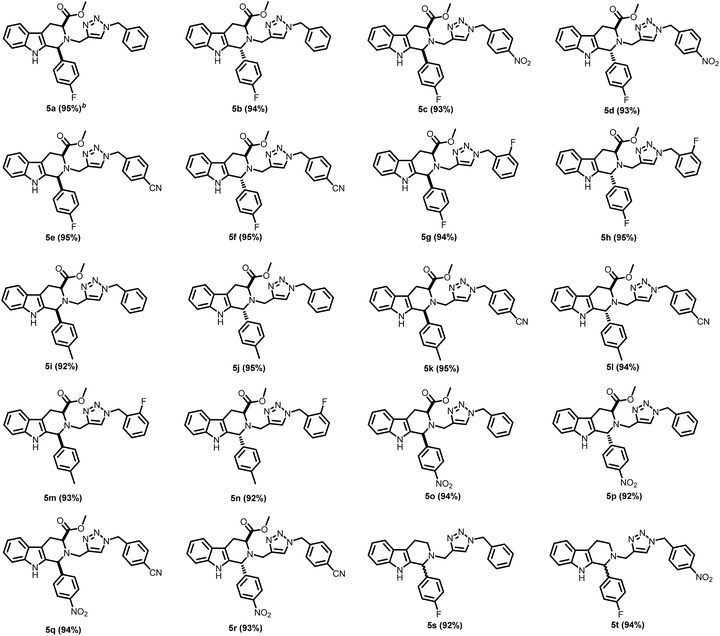 |
As an extension of the three component procedure, this catalytic system was further applied towards the synthesis of biologically relevant 1,2,3-triazolo-tetrahydro-β-carbolines (Table 3, entries 5a–t). The alkyne building blocks (4a–g) were prepared from the corresponding cis- and trans-tetrahydro-β-carbolines by propargylation of the piperidinyl nitrogen. These alkynes were reacted with a mixture of benzyl bromides (1a–d) and sodium azide by using the optimized method to give the corresponding 1,4-disubstituted-1,2,3-triazolo-tetrahydro-β-carbolines in good to excellent yields. The substitution on the phenyl ring at the position-1 of tetrahydro-β-carboline scaffold or on benzyl bromide did not have any appreciable influence on the outcome of the reaction. Moreover, there is no difference between the reaction rates of N-propargylated cis- and trans-tetrahydro-β-carbolines. Interestingly, N-propargylated tetrahydro-β-carboline lacking a methyl carboxylate group on 3-position (4g) also participated in this reaction to provide the corresponding products in 92% and 94% yields respectively (Table 3, entry 5s and 5t respectively). These excellent results indicate that the developed protocol can be used for the synthesis and ligation of complex biologically relevant molecules through 1,2,3-triazoles by ‘click’ reaction.
The novel 1,2,3-triazolo-tetrahydro-β-carboline derivatives were then evaluated for their in vitro cytotoxicity against A-549 (human lung carcinoma), HCT-116 (human colorectal carcinoma), BT-549 (human breast carcinoma), MDA-MB-231 (human triple negative breast carcinoma), PC-3 (human prostate carcinoma), NCI-H460 (human non small cell lung carcinoma), HCT-15 (human colorectal adenocarcinoma) cell lines by 3-(4,5-dimethylthiazol-2-yl)-2,5-diphenyltetrazolium bromide (MTT) assay and compared with that of the naturally occurring potent cytotoxic β-carboline, harmine. The results of this assay expressed as IC50 values were summarized in Table 4.
Table 4 Cytotoxicity (IC50 μM) of compounds 5a–t against selected human cancer cell lines
Compound |
A-549a |
HCT-116b |
BT-549c |
MDA-MB-231d |
PC-3e |
NCI-H460f |
HCT-15g |
A-549 (human lung carcinoma). HCT-116 (human colorectal carcinoma). BT-549 (human breast carcinoma). MDA-MB-231 (human triple negative breast carcinoma). PC-3 (human prostate carcinoma). NCI-H460 (human non small cell lung carcinoma). HCT-15 (human colorectal adenocarcinoma). Half maximal inhibitory concentration values (IC50) are the mean ± SD of three individual experiments performed in duplicate. Harmine (natural product) was included as reference standard. |
5a |
18.3 ± 1.06 |
>25 |
>25 |
20.1 ± 0.19 |
>25 |
>25 |
>25 |
5b |
9.7 ± 0.81 |
4.2 ± 0.42 |
9.3 ± 1.09 |
9.5 ± 0.33 |
8.5 ± 0.45 |
7.4 ± 0.19 |
7.3 ± 0.06 |
5c |
>25 |
>25 |
24.1 ± 0.54 |
>25 |
>25 |
25.0 ± 0.22 |
>25 |
5d |
>25 |
22.1 ± 1.29 |
21.1 ± 0.22 |
>25 |
>25 |
>25 |
>25 |
5e |
>25 |
>25 |
>25 |
>25 |
21.2 ± 0.23 |
>25 |
22.2 ± 0.26 |
5f |
>25 |
>25 |
17.2 ± 1.14 |
19.2 ± 0.24 |
19.9 ± 0.73 |
>25 |
>25 |
5g |
20.3 ± 0.16 |
>25 |
>25 |
>25 |
>25 |
>25 |
>25 |
5h |
>25 |
12.1 ± 0.19 |
6.3 ± 0.24 |
19.6 ± 0.31 |
23.1 ± 0.24 |
24.8 ± 0.22 |
23.2 ± 1.36 |
5i |
>25 |
>25 |
>25 |
>25 |
20.2 ± 0.12 |
>25 |
>25 |
5j |
16.2 ± 0.24 |
24.1 ± 0.25 |
16.6 ± 0.23 |
>25 |
>25 |
25.0 ± 0.13 |
>25 |
5k |
>25 |
>25 |
>25 |
>25 |
>25 |
>25 |
23.5 ± 1.16 |
5l |
>25 |
23.5 ± 0.13 |
>25 |
20.2 ± 0.36 |
>25 |
>25 |
>25 |
5m |
>25 |
>25 |
>25 |
19.2 ± 0.24 |
25.0 ± 0.14 |
>25 |
>25 |
5n |
21.2 ± 0.27 |
>25 |
18.2 ± 1.27 |
17.1 ± 0.72 |
>25 |
21.5 ± 0.46 |
24.2 ± 0.26 |
5o |
23.2 ± 0.40 |
>25 |
>25 |
>25 |
>25 |
>25 |
24.1 ± 0.11 |
5p |
>25 |
22.9 ± 0.09 |
9.4 ± 0.31 |
16.2 ± 0.84 |
22.1 ± 0.05 |
6.25 ± 0.14 |
>25 |
5q |
>25 |
>25 |
>25 |
>25 |
>25 |
>25 |
>25 |
5r |
>25 |
19.1 ± 0.24 |
18.3 ± 0.44 |
>25 |
>25 |
>25 |
>25 |
5s |
16.2 ± 1.20 |
23.3 ± 1.09 |
9.2 ± 0.51 |
>25 |
>25 |
20.2 ± 1.24 |
23.2 ± 0.15 |
5t |
>25 |
>25 |
>25 |
18.2 ± 0.46 |
20.2 ± 0.36 |
>25 |
>25 |
Harmine |
12.40 ± 1.1 |
18.14 ± 0.48 |
14.36 ± 0.18 |
14.2 ± 0.80 |
19.51 ± 0.60 |
22.3 ± 0.50 |
21.05 ± 0.40 |
In the cell lines investigated, these compounds have displayed significant in vitro cytotoxicity in comparison to that of harmine. The compounds 5b, 5h, 5p and 5s appear to be effective against the breast carcinoma (BT-549) cell line with IC50 values lower than 10 μM, among which compound 5h displayed the highest cytotoxicity (6.3 ± 0.24, IC50 μM). Compound 5h was also more cytotoxic against the colorectal carcinoma (HCT-116) cell line (12.1 ± 0.19, IC50 μM) than harmine (18.14 ± 0.48, IC50 μM). In the human non-small cell lung carcinoma (NCI-H460) cell line, only the hybrids 5b, 5n, 5p and 5s were more cytotoxic than harmine (22.3 ± 0.50, IC50 μM). Interestingly, the compound 5b displayed a wide range of cytotoxicities against all the tested human cancer cell lines, with IC50 values lower than 10 μM.
Relative viscosity experiments were performed to ascertain the mode of interaction of the most potent compounds 5b, 5h and 5p with DNA. Intercalation of molecules between the base pairs of the two strands of DNA increases the viscosity of DNA solutions considerably; whereas only slight increase in viscosity is shown by groove binders or DNA surface binders. In contrast, covalent DNA-binding usually reduces the relative viscosity of DNA solutions. The relative viscosities were compared with that of the controls, ethidium bromide, harmine and Hoechst 33258. The DNA intercalators, ethidium bromide and harmine showed a strong increase in the relative viscosity by lengthening the DNA double helix; whereas relative viscosity changed only slightly in case of Hoechst 33258, a minor groove binder. On slowly increasing the concentration of the 1,2,3-triazolo-tetrahydro-β-carboline derivatives 5b, 5h and 5p, the relative viscosity of the complex solutions has increased slightly. These results indicate that these derivatives are DNA minor groove binders. Among the derivatives studied, 5b showed better increase in viscosity than that of Hoechst 33258 upon its interaction with DNA, indicating significant minor groove binding affinity (Fig. 1). The data obtained from viscosity studies are consistent with the results obtained from in vitro cytotoxicity studies.
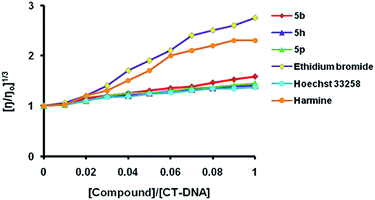 |
| Fig. 1 Relative viscosity experiment of hybrids 5b, 5h and 5p with CT-DNA. Ethidium bromide, harmine and Hoechst 33258 were used as controls. | |
Molecular docking simulations were performed into the DNA duplex d(CGCGAATTCGCG)2 using XP Glide 6.9 (Schrödinger 2015-4). The docked poses for one of the top scored compounds 5b, shown in Fig. 2, depict its binding along the curvature of the minor groove. This can be attributed to the presence of 1,2,3-triazole ring which forms a bend in the molecule and orients it perfectly in a crescent shape along length of the minor groove. Additionally, the benzyl group at the 1st position of 1,2,3-triazole ring formed side wise π–π stacking with the DNA base pairs. The hydrogen-bonds between the indole –NH, fluorine, and the side chains of the base pairs also lead to the stabilization of the docked poses electronically. All these interactions strengthen the minor groove binding affinity of the 1,2,3-triazolo-tetrahydro-β-carboline derivatives.
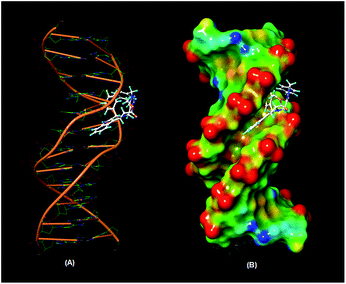 |
| Fig. 2 Docked pose of 5b in the DNA minor groove (A) and surface representation (B). | |
In order to study the formation of the complex and its stability, the best scored poses of DNA–ligand complexes with 5b, 5h and 5p from the molecular docking were subjected to Molecular Dynamics (MD) simulations. The snapshots of 5b-DNA complex during 4 ns MD simulation in explicit aqueous solution are shown in Fig. 3. Fig. 4 depicts the plot of root-mean-square deviation (RMSD) of the backbone atoms as a function of time. From the plot, it can be deduced that the complex does not show major deviation from the initial position over the course of 4 ns MD simulation. Even the docked poses of 5h and 5p were reasonably stable up to 4 ns MD simulation. Generalized Born (GB) method was used for the calculation of the final binding interaction energies which indicated stable complex formation.
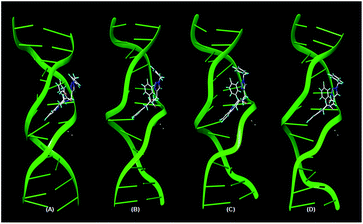 |
| Fig. 3 Snapshots of DNA-5b complex at 0 ns (A), 1 ns (B), 2 ns (C) and 4 ns (D) time scale. | |
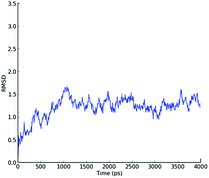 |
| Fig. 4 RMSD of the trajectories from backbone versus simulation time (4 ns). | |
Based on the in vitro cytotoxicity and DNA-binding affinity of the novel derivatives, the Structure Activity Relationships (SARs) were derived. It was observed that only the 1,2,3-triazolo-tetrahydro-β-carbolines with trans-configuration possessed significant cytotoxicity and DNA-binding potential. As deduced by molecular docking, this is due to the ability of the trans-compounds to adopt a crescent shape to bind along the curvature of the minor groove. Moreover, fluorine on the phenyl ring at the position-1 of tetrahydro-β-carboline scaffold enhanced the cytotoxicity and DNA-binding potential due to its hydrogen bonding propensity. As the benzyl group at the 1st position of triazole is involved in side wise π–π stacking interaction with the DNA base pairs, electron-withdrawing groups decreased their DNA binding affinity.
The efficiency of a catalyst depends upon its extent of reusability and its lifetime. Thus, the catalytic reusability was studied by performing a set of recycling experiments for the synthesis of 3a using 2 mol% of catalyst. At the end of each cycle, the products were recovered quantitatively from the reaction mixture by extraction using ethyl acetate. For the subsequent cycle, the ensuing aqueous layer containing the catalyst was loaded with the initial substrates, under the same reaction conditions. The catalytic solution could be recycled for four runs without any significant loss of reactivity, but by the fifth cycle, the yield of 3a had dropped to 75% as shown in Fig. 5. This may be attributed to the gradual increase in the concentration of NaBr in the aqueous layer after every reaction cycle, which may interfere with the catalytic activity.
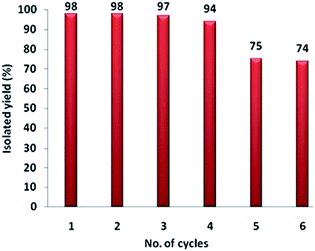 |
| Fig. 5 Recyclability chart of Cu(I)-catalyst in H2O for the synthesis of compound 3a. | |
Experimental section
General information
All solvents were purified and dried using standard methods prior to use. Commercially available reagents were used without further purification. 1H NMR spectra were recorded on an NMR instrument operated at 500 MHz. Chemical shifts are reported in ppm with the solvent resonance as the internal standard (CDCl3: δ = 7.26 ppm). 13C NMR spectra were recorded on an NMR instrument operated at 125 MHz with complete proton decoupling. Chemical shifts are reported in ppm with the solvent resonance as the internal standard (CDCl3: δ = 77.16 ppm). The following abbreviations were used for 1H NMR spectra to indicate the signal multiplicity: s (singlet), d (doublet), t (triplet), q (quartet) and m (multiplet). MS and HRMS were measured in EI or ESI mode and the mass analyzer of the HRMS was TOF. Thin layer chromatography was performed on pre-coated glass back plates and visualized with UV light at 254 nm. All computational modeling are performed on a desktop PC with a CentOS linux version 6.7 using Maestro software version 10.4. The viscosities of the complexes were determined by the Rolling-Ball Viscometer, Lovis 2000 M/ME (Anton Paar GmbH, Graz, Austria).
Bis[(tetrabutylammonium)di-μ-iodo-diiododicuprate(I) complex (nBu4N+)2(Cu2I4)=
A round bottomed flask equipped with mechanical stirrer was charged with CuI (209.48 g, 1.10 mol), TBAI (410.01 g, 1.11 mol) and peroxide free anhydrous THF (500 mL) under nitrogen atmosphere. The mixture was warmed at 50 °C until a homogenous pale yellow solution was obtained. The mixture was cooled to room temperature and then further to 6 °C over one hour. Subsequently, degassed tBuOMe (750 mL) was added slowly and the mixture was stirred for one hour at 6 °C. The crystalline solid was filtered off and washed with tBuOMe (450 mL) 5 times. The solid was dried under a stream of nitrogen to provide (nBu4N+)2(Cu2I4)= as an off white crystalline solid (600 g); mp 91–92 °C; 1H NMR (500 MHz, CDCl3): δ = 3.33–3.30 (m, 16H), 1.72–1.66 (m, 16H), 1.49 (apparent hextet, J = 7.4 Hz, 16H), 1.01 (t, J = 7.3 Hz, 24H); 13C NMR (125 MHz, CDCl3): δ = 59.22, 24.31, 19.82, 13.76.
General procedure for synthesis of compounds 3a–m and 5a–t
Bromide (1.0 mmol), NaN3 (1.0 mmol), and terminal alkyne (1.0 mmol) were added to a solution of the catalyst (2.0 mol%) in water (3 mL). The reaction mixture was stirred at room temperature for 4 h until the disappearance of the starting material, as indicated by TLC. Later, it was extracted with EtOAc (2 × 10 mL) and the combined organic phase was washed with water (2 × 10 mL), dried over anhydrous MgSO4, filtered and concentrated under reduced pressure. The residue obtained after evaporation contained the crude product and was recrystallized to yield the pure 1,2,3-triazoles.
1-Benzyl-4-phenyl-1H-1,2,3-triazole (3a)17. Colourless solid (227 mg, 97%); mp 126–127 °C; 1H NMR (500 MHz, CDCl3): δ = 7.80 (d, J = 7.32 Hz, 2H), 7.66 (s, 1H), 7.40–7.34 (m, 5H), 7.32–7.29 (m, 3H), 5.56 (s, 2H); 13C NMR (125 MHz, CDCl3): δ = 148.1, 134.6, 130.4, 129.0, 128.7, 128.0, 127.9, 125.6, 119.4, 54.1; HRMS (ESI-TOF) m/z calcd for C15H14N3 [M + H]+ 236.1188, found 236.1190.
4-((4-Phenyl-1H-1,2,3-triazol-1-yl)methyl)benzonitrile (3b)17. Off-white solid (249 mg, 96%); mp 135–136 °C; 1H NMR (500 MHz, CDCl3): δ = 7.80 (d, J = 7.32 Hz, 2H), 7.73 (s, 1H), 7.66 (d, J = 8.24 Hz, 2H), 7.42–7.31 (m, 5H), 5.63 (s, 2H); 13C NMR (125 MHz, CDCl3): δ = 148.5, 139.8, 132.8, 130.0, 128.8, 128.3, 128.2, 125.6, 119.6, 118.0, 112.6, 53.3; HRMS (ESI-TOF) m/z calcd for C16H13N4 [M + H]+ 261.1140, found 261.1143.
1-((1-Benzyl-1H-1,2,3-triazol-4-yl)methyl)-1,2,3,4-tetrahydroquinoline (3c). White solid (282 mg, 93%); mp 65–66 °C; 1H NMR (500 MHz, CDCl3): δ = 7.37–7.33 (m, 3H), 7.29 (s, 1H), 7.22–7.20 (m, 2H), 7.00 (t, J = 7.62 Hz, 1H), 6.95 (d, J = 7.32 Hz, 1H), 6.63–6.57 (m, 2H), 5.45 (s, 2H), 4.56 (s, 2H), 3.35 (t, J = 5.49 Hz, 2H), 2.74 (t, J = 6.25 Hz, 2H), 1.97–1.92 (m, 2H); 13C NMR (125 MHz, CDCl3): δ = 145.9, 144.7, 134.7, 129.1, 128.9, 128.5, 127.7, 127.0, 122.8, 121.4, 116.3, 111.1, 53.9, 49.6, 47.2, 27.8, 22.2; HRMS (ESI-TOF) m/z calcd for C19H21N4 [M + H]+ 305.1766, found 305.1770.
4-((4-((3,4-Dihydroquinolin-1(2H)-yl)methyl)-1H-1,2,3-triazol-1-yl)methyl)benzonitrile (3d). Yellow liquid (309 mg, 94%); 1H NMR (500 MHz, CDCl3): δ = 7.62 (d, J = 8.08 Hz, 2H), 7.33 (s, 1H), 7.27 (d, J = 8.08 Hz, 2H), 6.98 (t, J = 7.47 Hz, 1H), 6.95 (d, J = 7.01 Hz, 1H), 6.60–6.57 (m, 2H), 5.51 (s, 2H), 4.57 (s, 2H), 3.35 (t, J = 5.49 Hz, 2H), 2.73 (t, J = 6.25 Hz, 2H), 1.97–1.92 (m, 2H); 13C NMR (125 MHz, CDCl3): δ = 146.3, 144.6, 139.8, 132.7, 129.1, 128.1, 126.9, 122.8, 121.7, 118.0, 116.4, 112.5, 111.0, 53.1, 49.7, 47.2, 27.8, 22.2; HRMS (ESI-TOF) m/z calcd for C20H20N5 [M + H]+ 330.1719, found 330.1720.
1-Benzyl-4-((mesityloxy)methyl)-1H-1,2,3-triazole (3e). Colourless liquid (294 mg, 96%); 1H NMR (500 MHz, CDCl3): δ = 7.53 (s, 1H), 7.33 (Brs, 3H), 7.24 (Brs, 2H), 6.79 (s, 2H), 5.50 (s, 2H), 4.90 (s, 2H), 2.20 (s, 9H); 13C NMR (125 MHz, CDCl3): δ = 152.8, 144.8, 134.4, 133.2, 130.3, 129.2, 128.8, 128.5, 127.8, 122.4, 65.4, 53.9, 20.4, 16.0; HRMS (ESI-TOF) m/z calcd for C19H22N3O [M + H]+ 308.1763, found 308.1765.
1-(2-Fluorobenzyl)-4-((mesityloxy)methyl)-1H-1,2,3-triazole (3f). White solid (308 mg, 95%); mp 69–70 °C; 1H NMR (500 MHz, CDCl3): δ = 7.65 (s, 1H), 7.38–7.34 (m, 1H), 7.29 (t, J = 7.47 Hz, 1H), 7.17–7.11 (m, 2H), 6.81 (s, 2H), 5.61 (s, 2H), 4.92 (s, 2H), 2.22 (s, 9H); 13C NMR (125 MHz, CDCl3): δ = 161.4, 159.4, 152.9, 133.4, 130.8 (d, J = 8.17 Hz), 130.5, 129.3, 124.7 (d, J = 3.63 Hz), 121.8, 121.7, 115.8, 115.6, 65.5, 47.6, 20.6, 16.1; HRMS (ESI-TOF) m/z calcd for C19H21FN3O [M + H]+ 326.1669, found 326.1670.
4-((4-Allyl-2-methoxyphenoxy)methyl)-1-benzyl-1H-1,2,3-triazole (3g)18. Off-white solid (321 mg, 96%); mp 90–91 °C; 1H NMR (500 MHz, CDCl3): δ = 7.54 (s, 1H), 7.36–7.34 (m, 3H), 7.25–7.24 (m, 2H), 6.94 (d, J = 7.93 Hz, 1H), 6.69–6.67 (m, 2H), 5.97–5.89 (m, 1H), 5.49 (s, 2H), 5.23 (s, 2H), 5.08–5.04 (m, 2H), 3.80 (s, 3H), 3.32 (d, J = 6.71 Hz, 2H); 13C NMR (125 MHz, CDCl3): δ = 149.4, 145.7, 144.7, 137.4, 134.3, 133.7, 129.0, 128.6, 128.0, 122.7, 120.4, 115.6, 114.5, 112.1, 63.4, 55.7, 54.1, 39.7; HRMS (ESI-TOF) m/z calcd for C20H22N3O2 [M + H]+ 336.1712, found 336.1715.
4-((4-((4-Allyl-2-methoxyphenoxy)methyl)-1H-1,2,3-triazol-1-yl)methyl)benzonitrile (3h). Brown solid (342 mg, 95%); mp 95–96 °C; 1H NMR (500 MHz, CDCl3): δ = 7.64 (d, J = 7.62 Hz, 2H), 7.61 (s, 1H), 7.31 (d, J = 7.78 Hz, 2H), 6.92 (d, J = 8.08 Hz, 1H), 6.70–6.67 (m, 2H), 5.97–5.89 (m, 1H), 5.56 (s, 2H), 5.24 (s, 2H), 5.08–5.04 (m, 2H), 3.81 (s, 3H), 3.32 (d, J = 6.56 Hz, 2H); 13C NMR (125 MHz, CDCl3): δ = 149.4, 145.6, 145.3, 139.6, 137.3, 133.9, 132.7, 128.3, 122.9, 120.4, 118.0, 115.7, 114.5, 112.6, 112.2, 63.3, 55.7, 53.3, 39.7; HRMS (ESI-TOF) m/z calcd for C21H21N4O2 [M + H]+ 361.1665, found 361.1666.
1-Benzyl-4-((2-methoxyphenoxy)methyl)-1H-1,2,3-triazole (3i)18. Yellow solid (283 mg, 96%); mp 115–117 °C; 1H NMR (500 MHz, CDCl3): δ = 7.55 (s, 1H), 7.36–7.33 (m, 3H), 7.26–7.24 (m, 2H), 7.03 (d, J = 7.47 Hz, 1H), 6.94 (t, J = 7.78 Hz, 1H), 6.88–6.87 (m, 2H), 5.50 (s, 2H), 5.26 (s, 2H), 3.82 (s, 3H); 13C NMR (125 MHz, CDCl3): δ = 149.5, 147.4, 144.6, 134.3, 129.0, 128.6, 128.0, 122.7, 121.8, 120.8, 114.4, 111.7, 63.2, 55.7, 54.1; HRMS (ESI-TOF) m/z calcd for C17H18N3O2 [M + H]+ 296.1399, found 296.1400.
4-((4-((2-Methoxyphenoxy)methyl)-1H-1,2,3-triazol-1-yl)methyl)benzonitrile (3j). Yellow solid (307 mg, 96%); mp 82–83 °C; 1H NMR (500 MHz, CDCl3): δ = 7.63 (s, 1H), 7.61 (d, J = 7.78 Hz, 2H), 7.29 (d, J = 7.78 Hz, 2H), 7.00 (d, J = 7.78 Hz, 1H), 6.93 (t, J = 7.17 Hz, 1H), 6.87–6.83 (m, 2H), 5.54 (s, 2H), 5.23 (s, 2H), 3.79 (s, 3H); 13C NMR (125 MHz, CDCl3): δ = 149.4, 147.2, 144.9, 139.6, 132.6, 128.2, 123.1, 121.9, 120.7, 118.0, 114.3, 112.5, 111.7, 62.9, 55.6, 53.2; HRMS (ESI-TOF) m/z calcd for C18H17N4O2 [M + H]+ 321.1352, found 321.1355.
Methyl 2-(4-phenyl-1H-1,2,3-triazol-1-yl)acetate (3k). Colourless solid (208 mg, 96%); mp 81–82 °C; 1H NMR (500 MHz, CDCl3): δ = 7.91 (s, 1H), 7.84 (d, J = 7.17 Hz, 2H), 7.41 (t, J = 7.78 Hz, 2H), 7.33 (t, J = 7.21 Hz, 1H), 5.20 (s, 2H), 3.80 (s, 3H); 13C NMR (125 MHz, CDCl3): δ = 166.6, 148.1, 130.1, 128.7, 128.2, 125.7, 120.9, 53.0, 50.7; HRMS (ESI-TOF) m/z calcd for C11H12N3O2 [M + H]+ 218.0930, found 218.0932.
1-Phenyl-2-(4-phenyl-1H-1,2,3-triazol-1-yl)ethan-1-one (3l). White solid (241 mg, 92%); mp 146–148 °C; 1H NMR (500 MHz, CDCl3): δ = 8.02 (d, J = 7.21 Hz, 2H), 7.95 (s, 1H), 7.86 (d, J = 7.21 Hz, 2H), 7.67 (t, J = 7.23 Hz, 1H), 7.54 (t, J = 7.21 Hz, 2H), 7.43 (t, J = 7.21 Hz, 2H), 7.34 (t, J = 7.32 Hz, 1H), 5.89 (s, 2H); 13C NMR (125 MHz, CDCl3): δ = 190.2, 148.0, 134.6, 133.8, 130.2, 129.1, 128.8, 128.2, 128.1, 125.8, 121.5, 55.5; HRMS (ESI-TOF) m/z calcd 1 for C16H14N3O [M + H]+ 264.1137, found 264.1139.
2-(5-(4-Phenyl-1H-1,2,3-triazol-1-yl)pentyl)-1H-benzo[de]isoquinoline-1,3(2H)-dione (3m). White solid (352 mg, 86%); mp 142–143 °C; 1H NMR (500 MHz, CDCl3): δ = 8.54 (d, J = 6.40 Hz, 2H), 8.18 (d, J = 7.40 Hz, 2H), 7.82 (d, J = 6.40 Hz, 3H), 7.70 (t, J = 7.40 Hz, 2H), 7.40 (t, J = 7.10 Hz, 2H), 7.31 (t, J = 6.40 Hz, 1H), 4.42 (t, J = 6.40 Hz, 2H), 4.17 (t, J = 6.40 Hz, 2H), 2.08–2.02 (m, 2H), 1.84–1.78 (m, 2H), 1.51–1.45 (m, 2H); 13C NMR (125 MHz, CDCl3): δ = 164.1, 147.5, 133.9, 131.4, 131.1, 130.4, 128.7, 128.0, 126.8, 125.6, 122.4, 119.5, 50.1, 39.6, 29.7, 27.2, 23.7; HRMS (ESI-TOF) m/z calcd for C25H23N4O2 [M + H]+ 411.1821, found 411.1822.
Methyl (1S,3S)-2-((1-benzyl-1H-1,2,3-triazol-4-yl)methyl)-1-(4-fluorophenyl)-2,3,4,9-tetrahydro-1H-pyrido[3,4-b]indole-3-carboxylate (5a). Yellow solid (470 mg, 95%); mp 100–101 °C; 1H NMR (500 MHz, CDCl3): δ = 7.51 (d, J = 7.16 Hz, 1H), 7.45 (s, 1H), 7.39 (d, J = 7.16 Hz, 3H), 7.26–7.24 (m, 4H), 7.21–7.17 (m, 2H), 7.13–7.07 (m, 2H), 6.93 (t, J = 8.39 Hz, 2H), 5.54–5.45 (m, 3H), 4.13 (d, J = 8.16 Hz, 1H), 4.06 (t, J = 7.01 Hz, 1H), 3.83 (dd, J = 3.50, 14.49 Hz, 1H), 3.61 (s, 3H), 3.24–3.15 (m, 2H); 13C NMR (125 MHz, CDCl3): δ = 173.5, 163.6 (d, J = 247.04 Hz), 147.2, 137.8, 136.7, 134.9, 134.3, 130.6 (d, J = 8.17 Hz), 129.3, 128.9, 128.1, 127.0, 122.4 (d, J = 19.07 Hz), 119.6, 118.4, 115.8 (d, J = 20.79 Hz), 111.1, 106.7, 60.3, 58.8, 54.3, 51.7, 46.2, 24.4; HRMS (ESI-TOF) m/z calcd for C29H27FN5O2 [M + H]+, 496.2149, found 496.2150.
Methyl (1R,3S)-2-((1-benzyl-1H-1,2,3-triazol-4-yl)methyl)-1-(4-fluorophenyl)-2,3,4,9-tetrahydro-1H-pyrido[3,4-b]indole-3-carboxylate (5b). Pale yellow solid (465 mg, 94%); mp 134–135 °C; 1H NMR (500 MHz, CDCl3): δ = 7.50 (d, J = 7.17 Hz, 1H), 7.44 (s, 1H), 7.38 (d, J = 7.17 Hz, 3H), 7.25–7.23 (m, 4H), 7.20–7.16 (m, 2H), 7.12–7.06 (m, 2H), 6.92 (t, J = 8.39 Hz, 2H), 5.53–5.44 (m, 3H), 4.12–4.05 (m, 2H), 3.82 (dd, J = 3.50, 14.49 Hz, 1H), 3.60 (s, 3H), 3.23–3.14 (m, 2H); 13C NMR (125 MHz, CDCl3): δ = 173.2, 163.3 (d, J = 247.04 Hz), 146.9, 137.5, 136.4, 134.7, 134.1, 130.4 (d, J = 8.17 Hz), 129.0, 128.6, 127.9, 126.7, 122.1 (d, J = 19.07 Hz), 119.3, 118.1, 115.5 (d, J = 20.79 Hz), 110.8, 106.4, 60.0, 56.9, 54.0, 51.5, 45.9, 24.2; HRMS (ESI-TOF) m/z calcd for C29H27FN5O2 [M + H]+, 496.2149, found 496.2150.
Methyl (1S,3S)-1-(4-fluorophenyl)-2-((1-(4-nitrobenzyl)-1H-1,2,3-triazol-4-yl)methyl)-2,3,4,9-tetrahydro-1H-pyrido[3,4-b]indole-3-carboxylate (5c). Off-white solid (502 mg, 93%); mp 143–144 °C; 1H NMR (500 MHz, CDCl3): δ = 8.21 (d, J = 7.62 Hz, 2H), 7.51 (d, J = 6.41 Hz, 2H), 7.37 (d, J = 8.08 Hz, 2H), 7.32 (s, 2H), 7.17 (d, J = 7.01 Hz, 2H), 7.09 (t, J = 7.01 Hz, 2H), 7.01 (t, J = 7.62 Hz, 2H), 5.64–5.56 (m, 2H), 5.07 (s, 1H), 4.02–3.90 (m, 2H), 3.73 (s, 3H), 3.66 (d, J = 6.40 Hz, 1H), 3.26 (t, J = 14.03 Hz, 1H), 3.07 (d, J = 13.58 Hz, 1H); 13C NMR (125 MHz, CDCl3): δ = 173.5, 163.5 (d, J = 247.04 Hz), 147.9, 143.2, 141.6, 136.3, 135.9, 133.7, 131.3 (d, J = 8.17 Hz), 128.4, 126.3, 124.2 (d, J = 19.07 Hz), 121.9, 119.6, 118.0, 115.6 (d, J = 21.07 Hz), 110.8, 106.3, 61.5, 60.1, 52.9, 52.2, 45.2, 25.7; HRMS (ESI-TOF) m/z calcd for C29H26FN6O4 [M + H]+, 541.2000, found 541.2001.
Methyl (1R,3S)-1-(4-fluorophenyl)-2-((1-(4-nitrobenzyl)-1H-1,2,3-triazol-4-yl)methyl)-2,3,4,9-tetrahydro-1H-pyrido[3,4-b]indole-3-carboxylate (5d). White solid (502 mg, 93%); mp 99–100 °C; 1H NMR (500 MHz, CDCl3): δ = 8.22 (d, J = 8.08 Hz, 2H), 7.49 (d, J = 6.40 Hz, 2H), 7.36 (d, J = 8.08 Hz, 2H), 7.28 (s, 2H), 7.18 (d, J = 7.01 Hz, 2H), 7.09 (t, J = 7.01 Hz, 2H), 6.94 (t, J = 8.08 Hz, 2H), 5.63–5.55 (m, 2H), 5.48 (s, 1H), 4.15–4.05 (m, 2H), 3.85 (d, J = 6.40 Hz, 1H), 3.61 (s, 3H), 3.24–3.15 (m, 2H); 13C NMR (125 MHz, CDCl3): δ = 173.2, 163.3 (d, J = 247.05 Hz), 148.0, 147.5, 141.7, 137.5, 136.4, 134.0, 130.4 (d, J = 8.17 Hz), 128.4, 126.7, 124.2 (d, J = 19.07 Hz), 121.8, 119.4, 118.1, 115.6 (d, J = 21.07 Hz), 110.8, 106.3, 60.1, 57.0, 52.9, 51.5, 45.9, 24.3, HRMS (ESI-TOF) m/z calcd for C29H26FN6O4 [M + H]+, 541.2000, found 541.2001.
Methyl (1S,3S)-2-((1-(4-cyanobenzyl)-1H-1,2,3-triazol-4-yl)methyl)-1-(4-fluorophenyl)-2,3,4,9-tetrahydro-1H-pyrido[3,4-b]indole-3-carboxylate (5e). Yellow solid (494 mg, 95%); mp 119–120 °C; 1H NMR (500 MHz, CDCl3): δ = 7.65 (d, J = 8.08 Hz, 2H), 7.52–7.48 (m, 3H), 7.46 (d, J = 7.47 Hz, 1H), 7.33 (s, 1H), 7.30 (d, J = 8.08 Hz, 2H), 7.17 (d, J = 7.78 Hz, 1H), 7.12–7.06 (m, 2H), 7.01 (t, J = 8.54 Hz, 2H), 5.59–5.51 (m, 2H), 5.07 (s, 1H), 4.01–3.90 (m, 2H), 3.72 (s, 3H), 3.66 (dd, J = 3.81, 9.76 Hz, 1H), 3.28–3.23 (m, 1H), 3.08 (dd, J = 3.50, 14.80 Hz, 1H); 13C NMR (125 MHz, CDCl3): δ = 173.5, 163.5 (d, J = 247.05 Hz), 139.8, 136.3, 132.8, 131.3 (d, J = 8.17 Hz), 128.2, 126.3, 121.9, 119.5, 118.1 (d, J = 18.0 Hz), 115.5 (d, J = 20.17 Hz), 112.6, 110.8, 106.2, 61.4, 60.1, 53.2, 52.1, 45.1, 25.7; HRMS (ESI-TOF) m/z calcd for C30H26FN6O2 [M + H]+ 521.2101, found 521.2110.
Methyl (1R,3S)-2-((1-(4-cyanobenzyl)-1H-1,2,3-triazol-4-yl)methyl)-1-(4-fluorophenyl)-2,3,4,9-tetrahydro-1H-pyrido[3,4-b]indole-3-carboxylate (5f). Yellow solid (494 mg, 95%); mp 189–190 °C; 1H NMR (500 MHz, CDCl3): δ = 7.66 (d, J = 8.24 Hz, 2H), 7.50 (d, J = 7.47 Hz, 1H), 7.41 (s, 1H), 7.31–7.27 (m, 5H), 7.19 (d, J = 7.62 Hz, 1H), 7.13–7.07 (m, 2H), 6.96 (t, J = 8.24 Hz, 2H), 5.59–5.50 (m, 3H), 4.28–3.82 (m, 3H), 3.63 (s, 3H), 3.23 (Brs, 2H); 13C NMR (125 MHz, CDCl3): δ = 173.1, 163.4 (d, J = 247.04 Hz), 139.8, 136.4, 132.8, 130.5 (d, J = 8.17 Hz), 128.2, 126.7, 121.8, 119.4, 118.2 (d, J = 18.0 Hz), 115.6 (d, J = 20.17 Hz), 112.7, 110.8, 106.3, 60.1, 57.0, 53.2, 51.6, 46.0, 24.3; HRMS (ESI-TOF) m/z calcd for C30H26FN6O2 [M + H]+, 521.2101, found 521.2104.
Methyl (1S,3S)-2-((1-(2-fluorobenzyl)-1H-1,2,3-triazol-4-yl)methyl)-1-(4-fluorophenyl)-2,3,4,9-tetrahydro-1H-pyrido[3,4-b]indole-3-carboxylate (5g). Yellow solid (482 mg, 94%); mp 100–101 °C; 1H NMR (500 MHz, CDCl3): δ = 7.53–7.50 (m, 3H), 7.46 (d, J = 7.32 Hz, 1H), 7.37–7.33 (m, 1H), 7.24–7.22 (m, 2H), 7.17–7.06 (m, 5H), 7.01 (t, J = 8.54 Hz, 2H), 5.56 (s, 2H), 3.97 (s, 2H), 3.71 (s, 3H), 3.66–3.61 (m, 2H), 3.27–3.23 (m, 1H), 3.08 (dd, J = 3.20, 14.54 Hz, 1H); 13C NMR (125 MHz, CDCl3): δ = 173.2, 163.5 (d, J = 247.04 Hz), 161.4 (d, J = 247.95 Hz), 142.5, 136.4 (d, J = 16.34 Hz), 131.4 (d, J = 8.17 Hz), 130.8 (d, J = 8.17 Hz), 130.4 (d, J = 2.72 Hz), 126.4, 124.8 (d, J = 3.67 Hz), 123.9, 121.8, 119.5, 118.0, 115.8 (d, J = 20.88 Hz), 115.5 (d, J = 21.79 Hz), 110.8, 106.3, 61.0, 59.9, 52.0, 47.7, 47.6, 44.8, 25.7; HRMS (ESI-TOF) m/z calcd for C29H26F2N5O2 [M + H]+, 514.2055, found 514.2050.
Methyl (1R,3S)-2-((1-(2-fluorobenzyl)-1H-1,2,3-triazol-4-yl)methyl)-1-(4-fluorophenyl)-2,3,4,9-tetrahydro-1H-pyrido[3,4-b]indole-3-carboxylate (5h). Yellow solid (487 mg, 95%); mp 108–109 °C; 1H NMR (500 MHz, CDCl3): δ = 7.51 (d, J = 7.17 Hz, 1H), 7.43 (Brs, 1H), 7.38–7.34 (m, 1H), 7.30–7.29 (m, 3H), 7.23 (t, J = 7.01 Hz, 1H), 7.19–7.07 (m, 5H), 6.94 (t, J = 8.54 Hz, 2H), 5.57–5.48 (m, 3H), 4.13 (d, J = 7.17 Hz, 1H); 4.05 (t, J = 7.01 Hz, 1H), 3.85–3.79 (m, 1H), 3.61 (s, 3H), 3.24–3.21 (m, 2H); 13C NMR (125 MHz, CDCl3): δ = 173.0, 163.2 (d, J = 247.04 Hz), 161.2 (d, J = 247.94 Hz), 142.3, 136.4 (d, J = 16.34 Hz), 131.1 (d, J = 8.17 Hz), 130.6 (d, J = 8.17 Hz), 130.2 (d, J = 2.72 Hz), 126.1, 124.5 (d, J = 3.67 Hz), 123.6, 121.5, 119.2, 117.8, 115.6 (d, J = 20.88 Hz), 115.3 (d, J = 21.88 Hz), 110.5, 106.1, 60.8, 56.7, 51.8, 47.4, 47.3, 44.6, 25.5; HRMS (ESI-TOF) m/z calcd for C29H26F2N5O2 [M + H]+, 514.2055 found 514.2050.
Methyl (1S,3S)-2-((1-benzyl-1H-1,2,3-triazol-4-yl)methyl)-1-(p-tolyl)-2,3,4,9-tetrahydro-1H-pyrido[3,4-b]indole-3-carboxylate (5i). Yellowish brown solid (451 mg, 92%); mp 96–97 °C; 1H NMR (500 MHz, CDCl3): δ = 7.50 (d, J = 6.56 Hz, 1H), 7.41–7.37 (m, 4H), 7.24–7.19 (m, 5H), 7.15–7.14 (m, 1H), 7.10–7.05 (m, 4H), 5.53–5.44 (m, 3H), 4.12 (d, J = 14.64 Hz, 1H), 4.06 (t, J = 3.96 Hz, 1H), 3.85 (d, J = 14.64 Hz, 1H), 3.60 (s, 3H), 3.23–3.13 (m, 2H), 2.31 (s, 3H); 13C NMR (125 MHz, CDCl3): δ = 173.3, 147.2, 138.7, 137.7, 136.4, 134.7, 129.3, 129.0, 128.7, 128.6, 127.8, 126.8, 122.2, 121.5, 119.2, 118.1, 110.7, 106.1, 60.4, 56.8, 54.0, 51.4, 45.9, 24.3, 21.1; HRMS (ESI-TOF) m/z calcd for C30H30N5O2 [M + H]+ 492.2400, found 492.2403.
Methyl (1R,3S)-2-((1-(2-fluorobenzyl)-1H-1,2,3-triazol-4-yl)methyl)-1-(p-tolyl)-2,3,4,9-tetrahydro-1H-pyrido[3,4-b]indole-3-carboxylate (5j). White solid (466 mg, 95%); mp 127–128 °C; 1H NMR (500 MHz, CDCl3): δ = 7.49 (d, J = 6.86 Hz, 1H), 7.39–7.36 (m, 4H), 7.24–7.20 (m, 5H), 7.15–7.14 (m, 1H), 7.10–7.06 (m, 4H), 5.52–5.44 (m, 3H), 4.13–4.06 (m, 2H), 3.86 (d, J = 14.19 Hz, 1H), 3.61 (s, 3H), 3.23, 3.18 (m, 2H), 2.31 (s, 3H); 13C NMR (125 MHz, CDCl3): δ = 173.3, 147.2, 138.6, 137.6, 136.3, 134.7, 129.2, 128.9, 128.7, 128.5, 127.8, 126.8, 122.2, 121.4, 119.1, 118.0, 110.8, 106.1, 60.4, 56.7, 53.9, 51.4, 45.9, 24.2, 21.0; HRMS (ESI-TOF) m/z calcd for C30H30N5O2 [M + H]+ 492.2400, found 492.2403.
Methyl (1S,3S)-2-((1-(4-cyanobenzyl)-1H-1,2,3-triazol-4-yl)methyl)-1-(p-tolyl)-2,3,4,9-tetrahydro-1H-pyrido[3,4-b]indole-3-carboxylate (5k). Off-white solid (490 mg, 95%); mp 140–141 °C; 1H NMR (500 MHz, CDCl3): δ = 7.66 (d, J = 8.08 Hz, 2H), 7.50 (d, J = 7.01 Hz, 1H), 7.43 (s, 1H), 7.29 (t, J = 4.12 Hz, 3H), 7.22 (d, J = 7.62 Hz, 2H), 7.16–7.14 (m, 1H), 7.10–7.07 (m, 4H), 5.58–5.45 (m, 3H), 4.15 (d, J = 14.64 Hz, 1H), 4.05 (t, J = 5.49 Hz, 1H), 3.88 (d, J = 14.80 Hz, 1H), 3.61 (s, 3H), 3.24–3.14 (m, 2H), 2.31 (s, 3H); 13C NMR (125 MHz, CDCl3): δ = 173.2, 147.7, 139.9, 138.6, 137.8, 136.4, 134.5, 132.7, 129.3, 128.7, 128.1, 126.8, 122.5, 121.6, 119.2, 118.0, 112.6, 110.8, 106.0, 60.4, 56.8, 51.5, 45.9, 24.3, 22.6, 21.1; HRMS (ESI-TOF) m/z calcd for C31H29N6O2 [M + H]+ 517.2352, found 517.2348.
Methyl (1R,3S)-2-((1-(4-cyanobenzyl)-1H-1,2,3-triazol-4-yl)methyl)-1-(p-tolyl)-2,3,4,9-tetrahydro-1H-pyrido[3,4-b]indole-3-carboxylate (5l). Green solid (485 mg, 94%); mp 143–144 °C; 1H NMR (500 MHz, CDCl3): δ = 7.66 (d, J = 5.79 Hz, 2H), 7.49 (d, J = 3.81 Hz, 1H), 7.39 (s, 1H), 7.29 (t, J = 5.18 Hz, 3H), 7.22–7.08 (m, 7H), 5.59–5.46 (m, 3H), 4.15 (d, J = 14.64 Hz, 1H), 4.06 (t, J = 5.49 Hz, 1H), 3.89 (d, J = 12.81 Hz, 1H), 3.62 (s, 3H), 3.29–3.11 (m, 2H), 2.31 (s, 3H); 13C NMR (125 MHz, CDCl3): δ = 173.0, 147.5, 139.7, 138.3, 137.6, 136.1, 134.3, 132.5, 129.1, 128.4, 127.9, 126.5, 122.2, 121.3, 119.0, 117.8, 112.3, 110.5, 105.8, 60.2, 52.9, 51.2, 45.7, 24.1, 22.3, 20.8; HRMS (ESI-TOF) m/z calcd for C31H29N6O2 [M + H]+ 517.2352, found 517.2348.
Methyl (1S,3S)-2-((1-(2-fluorobenzyl)-1H-1,2,3-triazol-4-yl)methyl)-1-(p-tolyl)-2,3,4,9-tetrahydro-1H-pyrido[3,4-b]indole-3-carboxylate (5m). Yellow solid (473 mg, 93%); mp 166–167 °C; 1H NMR (500 MHz, CDCl3): δ = 7.50 (d, J = 6.25 Hz, 1H), 7.41 (s, 1H), 7.36–7.31 (m, 2H), 7.23–7.22 (m, 3H), 7.14 (t, J = 6.86 Hz, 3H), 7.09 (d, J = 6.25 Hz, 4H), 5.57–5.46 (m, 3H), 4.13–4.07 (m, 2H), 3.86 (d, J = 14.34 Hz, 1H), 3.61 (s, 3H), 3.24–3.21 (m, 2H), 2.32 (s, 3H); 13C NMR (125 MHz, CDCl3): δ = 173.3, 161.4 (d, J = 247.04 Hz), 147.2, 138.6, 137.7, 136.4, 134.7, 130.7 (d, J = 8.17 Hz), 130.3 (d, J = 2.72 Hz), 129.3, 128.7, 126.8, 124.7 (d, J = 3.63 Hz), 122.4, 122.1 (d, J = 14.53 Hz), 121.5, 119.2, 118.1, 115.7 (d, J = 20.88 Hz), 110.8, 106.1, 60.4, 56.8, 51.4, 47.5, 46.0, 24.3, 21.0; HRMS (ESI-TOF) m/z calcd for C30H29FN5O2 [M + H]+ 510.2305, found 510.2310.
Methyl (1R,3S)-2-((1-benzyl-1H-1,2,3-triazol-4-yl)methyl)-1-(p-tolyl)-2,3,4,9-tetrahydro-1H-pyrido[3,4-b]indole-3-carboxylate (5n). Yellow solid (468 mg, 92%); mp 97–98 °C; 1H NMR (500 MHz, CDCl3): δ = 7.54–7.50 (m, 2H), 7.39–7.33 (m, 2H), 7.24–7.19 (m, 3H), 7.16–7.11 (m, 3H), 7.08–7.07 (m, 4H), 5.57–5.44 (m, 3H), 4.12–4.06 (m, 2H), 3.83 (d, J = 14.64 Hz, 1H), 3.61 (s, 3H), 3.25–3.16 (m, 2H), 2.32 (s, 3H); 13C NMR (125 MHz, CDCl3): δ = 173.3, 161.3 (d, J = 247.04 Hz), 147.2, 138.6, 137.5, 136.3, 134.6, 130.6 (d, J = 8.17 Hz), 130.3 (d, J = 2.72 Hz), 129.2, 128.6, 126.8, 124.6 (d, J = 3.63 Hz), 122.4, 122.0 (d, J = 14.64 Hz), 121.3, 119.0, 117.9, 115.7 (d, J = 20.88 Hz), 110.7, 106.0, 60.3, 56.8, 51.3, 47.4, 45.8, 24.2, 21.0; HRMS (ESI-TOF) m/z calcd for C30H29FN5O2 [M + H]+ 510.2305, found 510.2310.
Methyl (1S,3S)-2-((1-benzyl-1H-1,2,3-triazol-4-yl)methyl)-1-(4-nitrophenyl)-2,3,4,9-tetrahydro-1H-pyrido[3,4-b]indole-3-carboxylate (5o). Yellow solid (490 mg, 94%); mp 179–180 °C; 1H NMR (500 MHz, CDCl3): δ = 8.16 (d, J = 8.69 Hz, 2H), 7.74 (d, J = 8.54 Hz, 2H), 7.47 (d, J = 7.01 Hz, 1H), 7.42 (s, 1H), 7.39–7.34 (m, 4H), 7.25–7.24 (m, 2H), 7.17–7.15 (m, 1H), 7.12–7.07 (m, 2H), 5.55–5.46 (m, 2H), 5.29 (s, 1H), 3.95–3.85 (m, 2H), 3.68 (s, 3H), 3.65 (dd, J = 3.96, 9.76 Hz, 1H), 3.28–3.23 (m, 1H), 3.10 (dd, J = 3.50, 14.95 Hz, 1H); 13C NMR (125 MHz, CDCl3): δ = 173.2, 148.3, 147.7, 142.0, 136.5, 134.4, 132.3, 130.4, 129.1, 128.7, 127.9, 126.2, 123.8, 123.7, 122.2, 119.7, 118.1, 110.9, 106.7, 60.8, 59.7, 54.1, 52.1, 45.3, 25.6; HRMS (ESI-TOF) m/z calcd for C29H27N6O4 [M + H]+ 523.2094, found 523.2095.
Methyl (1R,3S)-2-((1-benzyl-1H-1,2,3-triazol-4-yl)methyl)-1-(4-nitrophenyl)-2,3,4,9-tetrahydro-1H-pyrido[3,4-b]indole-3-carboxylate (5p). Yellow solid (480 mg, 92%) mp 179–180 °C; 1H NMR (500 MHz, CDCl3): δ = 8.02 (d, J = 8.69 Hz, 2H), 7.67 (s, 1H), 7.53–7.52 (m, 1H), 7.39–7.37 (m, 5H), 7.29 (d, J = 14.80 Hz, 2H), 7.25 (d, J = 1.83 Hz, 1H), 7.13–7.06 (m, 3H), 5.54–5.46 (m, 3H), 4.10 (d, J = 14.49 Hz, 1H), 3.98 (t, J = 5.34 Hz, 1H), 3.63 (d, J = 7.17 Hz, 1H), 3.60 (s, 3H), 3.24–3.15 (m, 2H); 13C NMR (125 MHz, CDCl3): δ = 172.9, 149.4, 147.4, 146.3, 136.5, 134.5, 132.4, 129.4, 129.1, 128.8, 127.9, 126.5, 123.7, 122.3, 122.0, 119.5, 118.2, 110.9, 106.9, 60.1, 56.7, 54.1, 51.6, 45.8, 23.8; HRMS (ESI-TOF) m/z calcd for C29H27N6O4 [M + H]+ 523.2094, found 523.2095.
Methyl (1S,3S)-2-((1-(4-cyanobenzyl)-1H-1,2,3-triazol-4-yl)methyl)-1-(4-nitrophenyl)-2,3,4,9-tetrahydro-1H-pyrido[3,4-b]indole-3-carboxylate (5q). Yellow solid (514 mg, 94%); 132–133 °C; 1H NMR (500 MHz, CDCl3): δ = 8.10 (d, J = 8.67 Hz, 2H), 7.67 (d, J = 8.25 Hz, 2H), 7.59 (s, 1H), 7.53–7.51 (m, 3H), 7.37 (s, 1H), 7.33 (d, J = 8.24 Hz, 2H), 7.19–7.17 (m, 1H), 7.13–7.08 (m, 2H), 5.63 (s, 1H), 5.56 (s, 2H), 4.19 (d, J = 14.60 Hz, 1H), 4.03 (t, J = 5.0 Hz, 1H), 3.77 (d, J = 14.52 Hz, 1H), 3.62 (s, 3H), 3.26–3.20 (m, 2H); 13C NMR (125 MHz, CDCl3): δ = 173.0, 147.9, 139.9, 136.9, 133.1, 129.8, 128.5, 126.7, 124.1, 122.5, 119.9, 118.6, 118.2, 113.0, 111.2, 107.1, 60.6, 59.9, 53.6, 52.0, 46.3, 24.3; HRMS (ESI-TOF) m/z calcd for C30H26N7O4 [M + H]+ 548.2046, found 548.2045.
Methyl (1R,3S)-2-((1-(4-cyanobenzyl)-1H-1,2,3-triazol-4-yl)methyl)-1-(4-nitrophenyl)-2,3,4,9-tetrahydro-1H-pyrido[3,4-b]indole-3-carboxylate (5r). Yellow solid (508 mg, 93%); mp 149–150 °C; 1H NMR (500 MHz, CDCl3): δ = 8.10 (d, J = 8.69 Hz, 2H), 7.66 (d, J = 8.24 Hz, 2H), 7.58 (s, 1H), 7.53–7.50 (m, 3H), 7.36 (s, 1H), 7.32 (d, J = 8.24 Hz, 2H), 7.19–7.16 (m, 1H), 7.13–7.08 (m, 2H), 5.63 (s, 1H), 5.56 (s, 2H), 4.19 (d, J = 14.64 Hz, 1H), 4.02 (t, J = 5.03 Hz, 1H), 3.77 (d, J = 14.49 Hz, 1H), 3.62 (s, 3H), 3.26–3.20 (m, 2H); 13C NMR (125 MHz, CDCl3): δ = 172.8, 147.7, 139.6, 136.6, 132.8, 129.6, 128.2, 126.5, 123.8, 122.2, 119.7, 118.3, 118.0, 112.8, 111.0, 106.8, 60.4, 56.8, 53.3, 51.7, 46.0, 24.1; HRMS (ESI-TOF) m/z calcd for C30H26N7O4 [M + H]+ 548.2046, found 548.2045.
2-((1-Benzyl-1H-1,2,3-triazol-4-yl)methyl)-1-(4-fluorophenyl)-2,3,4,9-tetrahydro-1H-pyrido[3,4-b]indole (5s). Light brown solid (402 mg, 92%); mp 239–240 °C; 1H NMR (500 MHz, CDCl3): δ = 7.50 (d, J = 6.56 Hz, 1H), 7.37–7.29 (m, 9H), 7.18 (d, J = 7.01 Hz, 1H), 7.09 (t, J = 6.56 Hz, 2H), 7.00 (t, J = 7.47 Hz, 2H), 5.57 (dd, J = 14.80, 39.36 Hz, 2H), 4.74 (s, 1H), 3.86 (dd, J = 14.19, 57.37 Hz, 2H), 3.23 (Brs, 1H), 2.96–2.77 (m, 3H); 13C NMR (125 MHz, CDCl3): δ = 163.4 (d, J = 247.04 Hz), 136.2, 134.6, 130.8 (d, J = 2.72 Hz), 129.0, 128.7, 127.9, 126.8, 122.6 (d, J = 19.07 Hz), 121.6, 119.3, 118.2, 115.6 (d, J = 21.8 Hz), 110.7, 108.8, 62.2, 54.0, 48.5, 48.3, 20.8; HRMS (ESI-TOF) m/z calcd for C27H25FN5 [M + H]+ 438.2094, found 438.2091.
1-(4-Fluorophenyl)-2-((1-(4-nitrobenzyl)-1H-1,2,3-triazol-4-yl)methyl)-2,3,4,9-tetrahydro-1H-pyrido[3,4-b]indole (5t). Yellow solid (453 mg, 94%); mp 246–247 °C; 1H NMR (500 MHz, DMSO-d6): δ = 10.32 (s, 1H), 8.24–8.19 (m, 3H), 7.52 (d, J = 8.24 Hz, 2H), 7.41 (d, J = 7.62 Hz, 1H), 7.35 (t, J = 6.86 Hz, 2H), 7.20–7.14 (m, 3H), 6.99 (t, J = 7.32 Hz, 1H), 6.94 (t, J = 7.47 Hz, 1H), 5.78 (s, 2H), 4.79 (s, 1H), 3.78 (dd, J = 10.68, 46.23 Hz, 2H), 3.10 (Brs, 1H), 2.77 (Brs, 2H), 2.67 (Brs, 1H); 13C NMR (125 MHz, DMSO-d6): δ = 162.4 (d, J = 247.04 Hz), 147.0, 144.1, 143.5, 137.5 (d, J = 2.72 Hz), 136.2, 134.3, 130.7 (d, J = 8.17 Hz), 128.7, 126.2, 124.3, 123.8, 120.5, 118.1, 117.5, 114.8 (d, J = 20.8 Hz), 110.9, 107.0, 60.5, 51.7, 47.6, 46.7, 20.1; HRMS (ESI-TOF) m/z calcd for C27H24FN6O2 [M + H]+ 483.1945, found 483.1940.
MTT assay
The cytotoxic activity of the compounds (5a–t) was determined using MTT assay. Initially, 1 × 104 cells per well were seeded in 100 mL DMEM, 10% FBS in each well of 96-well microculture plates and incubated for 24 h at 37 °C. Compounds, diluted to the desired concentrations (for preliminary screening; 20 mM concentration) in culture medium, were added to the wells. After 48 h of incubation, 10 mL MTT (3-(4,5-dimethylthiazol-2-yl)-2,5-diphenyl tetrazolium bromide) (5 mg mL−1) was added to each well and the plates were further incubated at 37 °C for 4 h. The supernatant from each well was carefully removed, formazan crystals were dissolved in 200 mL of DMSO and incubated at 37 °C for 10 min. All the values were expressed as mean ± SEM of three independent experiments in which each treatment was performed in triplicate wells. The human tumor cell lines used were A-549 (human lung carcinoma), HCT-116 (human colorectal carcinoma), BT-549 (human breast carcinoma), MDA-MB-231 (human triple negative breast carcinoma), PC-3 (human prostate carcinoma), NCI-H460 (human non small cell lung carcinoma), HCT-15 (human colorectal adenocarcinoma).
Viscosity measurements
The viscosities of the complexes were determined by the Rolling-Ball Viscometer, Lovis 2000 M/ME (Anton Paar GmbH, Graz, Austria), based on the falling ball principle. The temperature was controlled to ±0.005 K by a built in Peltier thermostat. A calibrated glass capillary (1.59 mm) with a steel ball was filled with the sample for measuring the ball falling time at angles in the range of 20° to 70°. The ball falling time and densities were used to estimate kinematic as well as dynamic viscosities at 25 °C. In each measurement, the uncertainty of the viscosity is 0.006 mPas. DNA solution was prepared in 100 mM Tris–HCl (pH 7.0). Viscosity was measured while each derivative (5 μM) was added to CT-DNA solution (50 μM). 5 μM solutions of ethidium bromide, harmine and Hoechst 33258 were used as controls. Data was represented graphically as (η/η0)1/3 versus the ratio of the concentration of the hybrid to CT-DNA, where η is the viscosity of CT-DNA in the presence of the derivative and η0 is the viscosity of CT-DNA solution.
Molecular modelling studies
The title compounds were built in Maestro 10.4, prepared using Ligprep 3.6 and geometrically minimized with Macromodel 11.0 followed by conformational analysis. Truncated Newton Conjugate Gradient minimization was used with 500 iterations and convergence threshold of 0.05 kJ mol−1. The d(CGCGAATTCGCG)2 duplex was prepared using protein preparation wizard. The Glide XP 6.9 algorithm was employed using a grid box volume of 10 × 10 × 10 Å. The lowest energy pose for each compound was selected and the docked complexes were further optimized using molecular dynamics simulations using Desmond 4.4 with OPLS-AA force field in explicit solvent with the TIP3P water model. Before MD simulations, the systems were minimized and pre-equilibrated using the default relaxation routine implemented in Desmond. Constant volume (NVT) MD simulation was performed for the first 100 ps during which temperature of the system was raised from 0 to 300 K and for further simulation the temperature was maintained at 300 K. Subsequently, the system was equilibrated in NPT which is composed of minimization and short MD simulation (12 and 24 ps) to relax the model system. After that, long equilibration MD simulation was performed for first 2 ns and long production MD simulation for 4 ns. Data were collected every 10 ps during the MD runs. The complexes present in trajectory file after production phase of MD simulations, were clustered according to the RMSD of backbone. The interaction energies in these clusters were calculated using the using MM/GBSA present in Prime 4.2.
Conclusions
In conclusion, a water soluble Cu(I)-catalyst was used for the efficient synthesis of 1,4-disubstituted 1,2,3-triazoles by a three-component ‘click’ reaction using alkyl/benzyl bromides, sodium azide, and terminal alkynes with mild reaction conditions. The advantages of this catalyst over previous reports are that preparation is easy and fast, catalyst recyclability and water has been used as green solvent. The triazole products could be easily isolated by means of simple extraction with ethyl acetate and further simple recrystallization. This catalytic system obviates the necessity to isolate potentially explosive organic azides and the Cu(I) complex can be used for the synthesis of significant bioactive anticancer molecules. This protocol can be further exploited for the conjugation of potent anti-cancer scaffolds through 1,2,3-triazoles to achieve synergistic effects.
Acknowledgements
S. N. and K. V. are thankful to DoP, Ministry of Chemicals and Fertilizers Govt. of India, New Delhi for the award of Research Fellowship. Dr N. Shankaraiah gratefully acknowledges SERB, DST, Govt. of India for research grant (YSS-2015-001709).
Notes and references
-
(a) V. V. Rostovtsev, L. G. Green, V. V. Fokin and K. B. Sharpless, Angew. Chem., 2002, 114, 2708–2711 (Angew. Chem., Int. Ed., 2002, 41, 2596–2599) CrossRef;
(b) C. W. Tornøe, C. Christensen and M. Meldal, J. Org. Chem., 2002, 67, 3057–3064 CrossRef;
(c) K. R. Senwar, P. Sharma, S. Nekkanti, M. Sathish, A. Kamal, B. Sridhar and N. Shankaraiah, New J. Chem., 2015, 39, 3973–3981 RSC.
-
(a) F. Amblard, J. H. Cho and R. F. Schinazi, Chem. Rev., 2009, 109, 4207–4220 CrossRef CAS PubMed;
(b) C. R. Becer, R. Hoogenboom and U. S. Schubert, Angew. Chem., Int. Ed., 2009, 48, 4900–4908 CrossRef CAS PubMed;
(c) E. M. Sletten and C. R. Bertozzi, Angew. Chem., Int. Ed., 2009, 48, 6974–6998 CrossRef CAS PubMed;
(d) K. B. Sharpless and R. Manetsch, Expert Opin. Drug Discovery, 2006, 1, 525–538 CrossRef CAS PubMed;
(e) G. C. Tron, T. Pirali, R. A. Billington, P. L. Canonico, G. Sorba and A. A. Genazzani, Med. Res. Rev., 2008, 28, 278–308 CrossRef CAS PubMed.
- W. G. Lewis, L. G. Green, F. Grynszpan, Z. Radić, P. R. Carlier, P. Taylor, M. G. Finn and K. B. Sharpless, Angew. Chem., Int. Ed., 2002, 41, 1053–1057 CrossRef CAS.
-
(a) Q. Wang, T. R. Chan, R. Hilgraf, V. V. Fokin, K. B. Sharpless and M. G. Finn, J. Am. Chem. Soc., 2003, 125, 3192–3193 CrossRef CAS PubMed;
(b) A. E. Speers, G. C. Adam and B. F. Cravatt, J. Am. Chem. Soc., 2003, 125, 4686–4687 CrossRef CAS PubMed.
- T. S. Seo, Z. Li, H. Ruparel and J. Ju, J. Org. Chem., 2003, 68, 609–612 CrossRef CAS PubMed.
- D. K. Dalvie, A. S. Kalgutkar, S. C. Khojasteh-Bakht, R. S. Obach and J. P. O'Donnell, Chem. Res. Toxicol., 2002, 15, 269–299 CrossRef CAS PubMed.
- W. S. Horne, M. K. Yadav, C. D. Stout and M. R. Ghadiri, J. Am. Chem. Soc., 2004, 126, 15366–15367 CrossRef CAS PubMed.
- E. F. V. Scriven and K. Turnbull, Chem. Rev., 1988, 88, 297–368 CrossRef CAS.
-
(a) R. B. Nasir Baig and R. S. Varma, Green Chem., 2012, 14, 625–632 RSC;
(b) H. Sharghi, R. Khalifeh and M. M. Doroodmand, Adv. Synth. Catal., 2009, 351, 207–218 CrossRef CAS;
(c) J.-A. Shin, Y.-G. Lim and K.-H. Lee, J. Org. Chem., 2012, 77, 4117–4122 CrossRef CAS PubMed;
(d) F. Alonso, Y. Moglie, G. Radivoy and M. Yus, Adv. Synth. Catal., 2010, 352, 3208–3214 CrossRef CAS;
(e) R. Hudson, C. J. Li and A. Moores, Green Chem., 2012, 14, 622–624 RSC;
(f) F. Alonso, Y. Moglie, G. Radivoy and M. Yus, Org. Biomol. Chem., 2011, 9, 6385–6395 RSC;
(g) B. J. Borah, D. Dutta, P. P. Saikia, N. C. Barua and D. K. Dutta, Green Chem., 2011, 13, 3453–3460 RSC;
(h) M. Nasr-Esfahani, I. Mohammadpoor-Baltork, A. R. Khosropour, M. Moghadam, V. Mirkhani, S. Tangestaninejad and H. A. Rudbari, J. Org. Chem., 2014, 79, 1437–1443 CrossRef CAS PubMed;
(i) T. Jin, M. Yan and Y. Yamamoto, ChemCatChem, 2012, 4, 1217–1229 CrossRef CAS.
- H. C. Kolb and K. B. Sharpless, Drug Discovery Today, 2003, 8, 1128–1137 CrossRef CAS PubMed.
- M. Asplund, S. Jagner and M. Nilsson, Acta Chem. Scand., Ser. A, 1982, 36, 751–755 CrossRef.
- S. Nekkanti, K. Veeramani, N. Praveen Kumar and N. Shankaraiah, Green Chem., 2016, 18, 3439–3447 RSC.
-
(a) N. Shahabadi and M. Maghsudi, Mol. BioSyst., 2014, 10, 338–347 RSC;
(b) A. Kamal, S. Prabhakar, M. J. Ramaiah, P. V. Reddy, C. R. Reddy, A. Mallareddy, N. Shankaraiah, T. L. N. Reddy, S. N. C. V. L. Pushpavalli and M.-P. Bhadra, Eur. J. Med. Chem., 2011, 46, 3820–3831 CrossRef CAS PubMed;
(c) A. Kamal, N. Shankaraiah, C. R. Reddy, S. Prabhakar, N. Markandeya, H. K. Srivastava and G. N. Sastry, Tetrahedron, 2010, 66, 5498–5506 CrossRef CAS;
(d) A. Kamal, S. Prabhakar, N. Shankaraiah, C. R. Reddy and P. V. Reddy, Tetrahedron Lett., 2008, 49, 3620–3624 CrossRef CAS;
(e) A. Kamal, N. Shankaraiah, V. Devaiah, K. L. Reddy, A. Juvekar, S. Sen, N. Kurian and S. Zingde, Bioorg. Med. Chem. Lett., 2008, 18, 1468–1473 CrossRef CAS PubMed.
- G. Barone, A. Terenzi, A. Lauria, A. M. Almerico, J. M. Leal, N. Busto and B. García, Coord. Chem. Rev., 2013, 257, 2848–2862 CrossRef CAS.
-
(a) L. C. Tu, C. S. Chen, I. C. Hsiao, J. W. Chern, C. H. Lin, Y. C. Shen and S. F. Yeh, Chem. Biol., 2005, 12, 1317–1324 CrossRef CAS PubMed;
(b) S. Sarkar, P. Pandya and K. Bhadra, PLoS One, 2014, 9, e108022 Search PubMed.
-
(a) W. A. Silva, M. T. Rodrigues, N. Shankaraiah, R. B. Ferreira, C. K. Z. Andrade, R. A. Pilli and L. S. Santos, Org. Lett., 2009, 11, 3238–3241 CrossRef PubMed;
(b) N. Shankaraiah, K. P. Siraj, S. Nekkanti, V. Srinivasulu, M. Satish, P. Sharma, K. R. Senwar, M. V. P. S. Vishnuvardhan, S. Ramakrishna and A. Kamal, Bioorg. Chem., 2015, 59, 130–139 CrossRef CAS PubMed;
(c) N. Shankaraiah, S. Nekkanti, K. J. Chudasama, K. R. Senwar, P. Sharma, M. K. Jeengar, V. G. M. Naidu, V. Srinivasulu and A. Kamal, Bioorg. Med. Chem. Lett., 2014, 24, 5413–5417 CrossRef CAS PubMed;
(d) A. Kamal, V. Srinivasulu, V. L. Nayak, M. Sathish, N. Shankaraiah, C. Bagul, N. V. S. Reddy, N. Rangaraj and N. Nagesh, ChemMedChem, 2014, 9, 2084–2098 CrossRef CAS PubMed;
(e) S. Nekkanti, N. Praveen Kumar, P. Sharma, A. Kamal, F. M. Nachtigall, O. Forero-Doria, L. S. Santos and N. Shankaraiah, RSC
Adv., 2016, 6, 2671–2677 CAS;
(f) N. Shankaraiah, C. Jadala, S. Nekkanti, K. R. Senwar, N. Nagesh, S. Shrivastava, V. G. M. Naidu, M. Sathish and A. Kamal, Bioorg. Chem., 2016, 64, 42–50 CrossRef CAS PubMed;
(g) A. Kamal, M. Sathish, A. V. G. Prasanthi, J. Chetna, Y. Tangella, V. Srinivasulu, N. Shankaraiah and A. Alarifi, RSC Adv., 2015, 5, 90121–90126 RSC.
- W. Wang, J. Wu, C. Xia and F. Li, Green Chem., 2011, 13, 3440–3445 RSC.
- M. Irfan, B. Aneja, U. Yadava, S. I. Khan, N. Manzoor, C. G. Daniliuc and M. Abid, Eur. J. Med. Chem., 2015, 93, 246–254 CrossRef CAS PubMed.
Footnote |
† Electronic supplementary information (ESI) available: 1H and 13C NMR. See DOI: 10.1039/c6ra22942e |
|
This journal is © The Royal Society of Chemistry 2016 |