DOI:
10.1039/C6RA19971B
(Paper)
RSC Adv., 2016,
6, 92688-92698
Purification, characterization, synthesis, in vivo and in vitro antihypertensive activity of bioactive peptides derived from coconut (Cocos nucifera L.) cake globulin hydrolysates
Received
7th August 2016
, Accepted 20th September 2016
First published on 22nd September 2016
Abstract
Coconut cake globulin hydrolysates (CCGH) with high ACE inhibitory activity (52.16%) were obtained by the sequential digestion of alcalase, flavourzyme, pepsin and trypsin assisted by high pressure pretreatment. It was found that systolic blood pressure of spontaneously hypertensive rats was reduced markedly (P < 0.05) after single and chronic oral administration of CCGH. CCGH were separated by ultrafiltration, Sephadex G-15 gel chromatography and RP-HPLC. Finally, two peptides Pro–Gln–Phe–Tyr–Trp (739.84 Da) and Val–Val–Leu–Tyr–Arg (648.81 Da) were identified. Their IC50 (the concentration of peptide that is required to inhibit 50% of ACE activity) were 0.104 ± 0.012 and 0.244 ± 0.026 mg mL−1. The two peptides exhibited potent non-competitive ACE inhibition and relatively good stability against gastrointestinal enzyme digestion. Furthermore, the two peptides could significantly lower the endothelin-1 content, and protect vascular endothelial cells from reactive oxygen species mediated damage. The results showed that coconut cake globulin could be effectively bioconverted to produce bioactive peptides, which could be used as a functional food ingredient to control ACE activity.
1. Introduction
Hypertension is the most common risk factor of heart diseases, and is identified as a major risk factor for the development of arteriosclerosis, stroke, myocardial infarction and end-stage renal disease, affecting one fourth of adults in the world.1 Blood pressure is regulated by a complex system involving the Renin–Angiotensin System (RAS), sympathetic nervous system, kidney and fluid balance mechanisms and nitric oxide system.2 Recently, the endothelin system has gained an increasingly recognized role in blood pressure regulation, in which the endothelin converting enzyme cleaves the biologically inactive intermediate termed big endothelin-1 to form endothelin-1, which has powerful vasoconstrictor and pressor properties.3 Moreover, more and more epidemiological evidence indicates that oxidative stress and associated oxidative damage are mediators in cardiovascular diseases including hypertension.4 Angiotensin-I converting enzyme (ACE) plays an important role in the regulation of blood pressure because catalyzes the conversion of inactive angiotensin-I into angiotensin-II, a potent vasoconstrictor and inactivates bradykinin, a potent vasodilator.5 Therefore the most well accepted mechanism underlying the blood-pressure-lowering effect of antihypertensive substrates is to be inhibition of ACE. Synthetic ACE inhibitors such as captopril and enalapril are often used to treat hypertension but they can cause adverse side effects. Since nutritional factors have been recognized to play a significant role in the prevention and/or treatment of hypertension, efforts are being put into the production of foods with antihypertensive activity. In the recent past, ACE inhibition peptides have been obtained from various food nature sources, including animal protein sources and plant protein sources like soybean, wheat and corn.6–9 Apart from the release, identification and in vivo or/and in vitro physiological effects, the structure–activity relationship and mechanism of action of some antihypertensive peptides, as well as their stability to processing and storage conditions used in food industry have been studied.5
Coconut cake, a byproduct of the coconut oil industry, contains about 10–16% of protein.10 Coconut cake protein (CCP) is a good source of edible protein for its abundant of essential amino acids and high emulsifying properties.11 Moreover, Mini and Rajamohan reported that CCP could prevent hyperlipidemia and lower serum cholesterol.12 Furthermore, the antioxidant and antifungal activity of CCP hydrolysates and bioactive peptides have been previously reported.13,14 And three antimicrobial peptides (Cn-AMP1, Cn-AMP2 and Cn-AMP3) were identified from green coconut water.15,16 However, there is little information regarding the antihypertensive effect of peptides from CCP as well as their preparation, purification and bioactivity.
Salt soluble seed storage protein is commonly known as globulin. The seed storage globulins from various sources make a significant contribution to the human diet. Most of the oil seeds like soybean, sunflower, groundnut and oil palm contain globulin as the major seed storage protein. Protein rich oil seed meal was being utilized to recover globulin to be marketed as food ingredients in developed countries. Globulin is the predominant fraction of CCP, accounting for about 60%.11 Previous study has found that the coconut cake globulin (CCG) had good antioxidant activity, excellent chelating ability on Fe2+ (89.14 ± 3.42%), and considerable protective effect against DNA damage and ACE inhibition ability,13 indicating that bioactivity peptides with potential hypertensive effect may be isolated from it. In addition, about 200 million tons of coconut cake is produced in the world, meaning that a large amount of protein could be produced.17 If peptides with high antihypertension could be obtained from it, the usage of coconut cake will be expanded and remarkable economic benefits may be achieved.
Therefore, the current study aims at the isolation, identification and synthesis of ACE inhibition peptides from enzymatic hydrolysates of coconut cake globulin. Their antihypertensive effect in vitro and in vivo, stability against gastrointestinal enzymes digestion, effect on intracellular endothelial-1 are studied, too.
2. Materials and methods
2.1. Materials and reagents
Coconut cake was obtained from South Coconut Food Processing Co., China. Flavourzyme (5 × 104 U g−1), alcalase (1 × 105 U g−1), pepsin (1 × 105 U g−1) and trypsin (1 × 105 U g−1) were purchased from Shanghai Biotech. Co., Ltd. (China). ACE (from rabbit lung) and N-hippuryl-His-Leu hydrate (HHL) were purchased from Sigma Co., USA. The human endothelial cell line (EA.hy926) was from the Type Culture Collection of the Chinese Academy of Sciences, Shanghai, China. Dulbecco's modified Eagle's medium (DMEM) and fetal bovine serum (FBS) were purchased from Sijiqing Biological Co., China. Human endothelin-1 (ET-1) Elisa kit was purchased from Ruiqi Biological Co. Ltd., China. MTT (3-(4,5-dimethylthiazol-2-yl)-2,5-diphenyltetrazolium bromide) and other chemicals and reagents were of analytical grade.
2.2. Preparation of coconut cake globulin (CCG)
Coconut cake was defatted three times with n-hexane (1
:
10, g mL−1) and dried, ground and passed through a sieve of 0.2 mm mesh. Thirty grams of defatted coconut cake were suspended in 300 mL of 0.4 M NaCl and stirred at 4 °C for 1 h. After filtrated, the residue was suspended in 0.4 M NaCl again and stirred at 4 °C for 1 h. This step was repeated in three times. The filtration was collected and centrifuged at 10000g for 30 min, the supernatant was collected, dialyzed against distilled water for 24 h at 4 °C. Then the dialyzation was centrifuged at 10000g for 30 min, and the precipitate was collected and lyophilized. Then coconut cake globulin (CCG) was obtained and stored at −20 °C.
2.3. Preparation of coconut cake globulin hydrolysates (CCGH)
To get peptides with high degree of hydrolysis (DH) and ACE inhibition activity, CCG was subjected to different treatments prior to enzymatic hydrolysis. Following the method of Zhang et al.18 with some modifications, four CCG were made for each pretreatment, one was left untreated and three were subjected to heating, ultrasound and high pressure treatment (HP), respectively. Two milliliters of CCG solution (5 g/100 mL) to be heated was incubated in a boiling water bath for 15 min, then immediately cooled to room temperature by tap water. The CCG solution (5 g/100 mL, 200 mL) for HP treatment was transferred into polyethylene bags and sealed under vacuum. HP treatment was performed by a HPP-L3 high-pressure apparatus (Tianjin HuaTaiSenMiao Biotechnology Co. Ltd., China) at 400 MPa, 35 °C for 15 min. Meanwhile the CCG solution (5 g/100 mL, 200 mL) for ultrasound treatment was treated using an ultrasonic generator (ST-1004, Guilin Sanxing ultrasonic equipment Co. Ltd., China) at 28 kHz, 600 W for 30 min (Pulse durations of on-time 2 s and off-time 2 s). These CCG solutions treated by heating, HP and ultrasound were collected and subjected to enzymatic hydrolysis, respectively.
Enzymatic hydrolysis of unpretreated CCG and three pretreated CCGs was performed using four different enzymes in the following sequence: alcalase, flavourzyme, pepsin and trypsin. The hydrolysis conditions used for these enzymes were shown in Table 1. At the end of the hydrolysis, the reaction solutions were incubated in 100 °C for 5 min to active the enzyme, and then centrifuged at 10000g, 4 °C for 20 min. The supernatant were collected, lyophilized, and then CCGHs with/without different pretreatments were obtained. The degree of hydrolysis (DH) and ACE inhibition ability of CCGH were determined using the method of Adler-Nissen and Jimsheena & Gowda,19,20 respectively. Results in Table 1 showed that the CCGH with HP pretreatment exhibited the highest DH and ACE inhibitory activity, thus it was chose to separate and identify antihypertensive peptides.
Table 1 Effects of different pretreatments on the degree of hydrolysis (DH) and ACE inhibition activity of coconut cake globulin hydrolysatesa
Pretreatments |
Proteolysisb |
DH (%) |
ACE inhibition activityc (%) |
Different small letters (a–d) in the same column means significant difference (P < 0.05). Proteolysis using enzymes in sequence of Alcalase (45 °C, pH 8.0, usage dose: 0.5 g/100 g protein, hydrolyzed time: 2 h), flavourzyme (50 °C, pH 7.0, usage dose: 0.5 g/100 g protein, 2 h), pepsin (37 °C, pH 2.0, usage dose: 0.3 g/100 g protein, 1 h), trypsin (37 °C, pH 7.0, usage dose: 0.3 g/100 g protein, 1 h). ACE inhibition activity of coconut cake globulin hydrolysates was tested at 1.0 mg mL−1. |
Unpretreated |
Alcalase, flavourzyme, pepsin, trypsin |
24.82 ± 2.26c |
25.07 ± 3.20c |
Heating, 100 °C, 15 min |
Alcalase, flavourzyme, pepsin, trypsin |
29.81 ± 4.30b |
38.03 ± 3.45b |
Ultrasonication, 28 kHz, 600 W, 30 min |
Alcalase, flavourzyme, pepsin, trypsin |
29.53 ± 8.14b |
37.25 ± 8.88b |
High pressure, 400 MPa, 15 min |
Alcalase, flavourzyme, pepsin, trypsin |
34.49 ± 7.82a |
52.16 ± 5.77a |
2.4. Separation by ultrafiltration and Sephadex G-15 gel chromatography
CCGH prepared by mixed enzymes assisted with HP was filtered sequentially using an ultrafiltration unit (Pellicon XL, Millipore, USA) through two ultrafiltration membranes with molecular weight (MW) cut-off of 5 and 3 kDa, respectively. Three fractions were obtained: CCGH-I with MW > 5 kDa, CCGH-II MW 3–5 kDa and CCGH-III with MW < 3 kDa. The fraction with the highest ACE inhibitory activity was further separated by a Sephadex G-15 gel filtration column (Φ 1.6 cm × 80 cm) equilibrated with distilled water at 0.6 mL min−1. The column was eluted with distilled water and monitored at 220 nm. Fractions were collected, lyophilized and stored at −20 °C.
2.5. ACE inhibition activity and inhibition pattern
According to the method of Jimsheena & Gowda,20 50 μL of samples (1.0 mg mL−1), 50 μL of ACE (25 mill units per mL) and 150 μL of 8.3 mM HHL were mixed and incubated at 37 °C for 60 min, followed by termination of the reaction by adding 250 μL of 1 M HCl. Then 1.4 mL of ethyl acetate was added and the mixture was vortexed for 5 s and centrifuged at 14100g for 5 min. 1 mL of the upper organic phase was transferred into a test tube and placed in a vacuum oven at 80 °C for 1 h. Subsequently, 2 mL of distilled water was added and the absorbance at 228 nm was read. Captopril (1 mg mL−1) was used as the comparison. The inhibition (%) was calculated as below: |
ACE inhibition (%) = (1 − AS/AC) × 100
| (1) |
where, AC was the absorbance of the ACE solution without an inhibitor, AS was the absorbance of mixture contained samples. Determination of the ACE inhibition pattern was carried out according to the method published by Bush et al.21 Different concentrations of ACE-inhibitory peptide (0.1, 0.2, 0.5 mg mL−1) were added to each reaction mixture and the enzyme activity was measured with different concentrations of HHL (0.76, 1.52, 3.04, 3.80 and 7.60 mM). The ACE-inhibitory activity was determined and the kinetics of ACE in the presence of the inhibitor was determined by Lineweaver–Burk plots.
2.6. Antihypertensive effect in spontaneously hypertensive rats (SHRs)
CCGH fraction from Sephadex G-15 chromatography with the highest ACE inhibition activity was evaluated the antihypertensive effect in (SHR). Twenty-five male SHRs aged 8 weeks were obtained from Vital River Laboratory Animal Technology Co., Ltd (Beijing, China). The rats were housed in colony cages maintained at 25 ± 1 °C and 45–55% relative humidity with a 12 h light/dark cycle. Tap water was freely available. After an acclimatization period of 1 week, the rats were divided into five groups of five rats each. The rats in negative control group were orally administered by gastric intubation a single dose of physiological saline (0.5 mL) once daily. And the rats in positive control group received captopril at 14 mg per kg per bodyweight once daily. The animals in the low-dosage, middle-dosage and high-dosage CCGH-treated groups received CCGH (dissolved in 1 mL of 0.9% saline) at 50, 100 and 200 mg per kg per bodyweight once daily, respectively. All of the treatments were conducted for 6 consecutive weeks. Every one week, the systolic blood pressure (SBP) and diastolic blood pressure (DBP) the rats were measured by the tail cuff method22 with a non-invasive blood-pressure apparatus (BP-300A Non-Invasive Blood Pressure Monitoring System, Chengdu, China). Each rat was measured at least three times. The heart rate of the rates was determined at the same time. At least 6 readings were recorded.
Moreover, these animal experiments were conducted in conformity with institutional guidelines for the care and use of laboratory animals in Chinese Tropical Agriculture Academic, Hainan, China, and conformed to the National Institutes of Health Guide for care and Use of Laboratory Animals (Publication No. 85-23, revised 1985). All experimental protocols were approved by the Review Committee for the Use of Human or Animal Subjects of Chinese Tropical Agriculture Academic.
2.7. Purification of the antihypertensive peptides
The CCGH fraction from Sephadex G-15 chromatography showing the highest ACE inhibition activity was separated by reversed-phase high performance liquid chromatography (RP-HPLC) on a Zorbax semi-preparative C18 column (Φ 9.4 mm × 250 mm, Agilent Technologies, USA), using a linear gradient of acetonitrile containing 0.1% TFA (5–30%, in 30 min) at a flow rate of 2.5 mL min−1. The fraction showing the highest ACE inhibition activity was further isolated on a Zorbax analysis C18 column (Φ 4.6 mm × 250 mm, Agilent Technologies, USA) with a linear gradient of acetonitrile containing 0.1% TFA (5–25%, in 20 min) at a flow rate of 1.0 mL min−1. The fractions with high activity were rechromatographed to confirm their purity on the same analytical C18 column at a flow rate of 1.0 mL min−1 with a linear gradient of acetonitrile containing 0.1% TFA (15–30%, in 12 min). The fractions with high ACE inhibition activity were subjected to LC-MS/MS analysis.
2.8. Molecular mass and amino acid sequence of the purified peptides
Accurate amino acid sequence and molecular mass of the purified peptides were determined by LC-MS/MS with a coupled Eksigent Nano LC (Eksigent Technologies, Dublin, CA, USA) and Thermo LTQ linear ion trap mass spectrometer (Thermo Fisher, San Jose, CA, USA). The acquired MS/MS data were interpreted using the bioinformatics search engine Mascot version 2.1.0 (Matrix Sciences, London, UK). The peptide sequences were matched to the published sequences of Elaeis guineensis proteins from the National Center for Biotechnology Information (NCBI, Bethesda, MD, USA) database.
2.9. Peptide synthesis
ACE inhibitory peptides identified in CCGH were synthesized by the solid phase procedure with a Liberty microwave peptide synthesizer (Mathews, NC, USA). Briefly, the peptides were analyzed with a Kromasil 100-5 C18 column (4.6 × 250 mm, particle size 5 μm) eluted with mobile phase consisting of 0.1% TFA in water and 0.1% TFA in acetonitrile. The purity and molecular mass of the synthetic peptides were determined by liquid chromatography coupled to mass spectrometry.
2.10. Stability of the synthetic peptides
Following the method of Tavares et al.,23 peptides solutions were adjusted to pH 2.0 and mixture with pepsin (E/S = 1
:
35), incubated at 37 °C for 1 h. Afterwards, the reaction mixture was adjusted to pH 7.0, pancreatin was added (1 g/25 g peptide). After incubated at 37 °C for 2 h, the digestion was terminated after boiling for 10 min. The ACE inhibition activity of the treated synthetic peptides and the untreated synthetic peptides were determined, respectively.
2.11. Effect on EA.hy926 cells' proliferation
The EA.hy926 cells was seeded in a 96-well plate at a density of 1.0 × 105 cells per mL, cultured in DMEM (containing 1% non-essential amino acid solution, 10% FBS, 100 IU mL−1 penicillin and 100 μg mL−1 streptomycin) in a humidified atmosphere of 5% CO2 at 37 °C for 24 h. Then the wells were washed with phosphate buffer solution (PBS) and cultured in fresh DMEM but without FBS at 37 °C for 12 h. The DMEM without FBS was removed and the cells were cultured in DMEM at 37 °C for another 24 h. Then the cells were treated with gradated concentrations of peptides (0.25, 0.5 and 1.0 mg mL−1) for 24 h. Captopril (2.0 mg mL−1) was used as the positive control, whereas the negative control was just given DMEM. Each treatment was repeated in six wells. After treatment, viability of the cells was measured using MTT method described by Mosmann.24 Briefly, the cells were washed with PBS and 20 μL of MTT (5 mg mL−1) were added into each well and kept at 37 °C for 4 h. Then MTT solution was removed and 100 μL of dimethyl sulfoxide were added. The absorbance was measured at 490 nm by a Varioskan Flash (Thermo Scientific, USA).
2.12. Effect on intracellular ET-1 content
The EA.hy926 cells were seeded in a 96-well plate at a density of 1.0 × 105 cells per mL. After being cultured in the DMEM at 37 °C for 24 h, the cells were treated with different concentrations of test samples (0.25, 0.5 and 1.0 mg mL−1) for 48 h. Captopril (2 mg mL−1) was used as positive control and the negative control was just given DMEM. After treatment, the growth medium of each well was collected to quantify ET-1 content using the ET-1 Elisa kit following the supplier's instructions.
2.13. Superoxide radical-scavenging activity
According to the method of Marklund and Marklund,25 100 μL of gradated concentration of samples (50–250 μg mL−1) and 3 mL of pyrogallol solution (3 mM) were mixed, the absorbance at 320 nm were recorded at 30 s intervals using a spectrophotometer. The scavenging activity was determined as the percentage of inhibiting pyrogallol autoxidation, which was calculated from the absorbance at 320 nm in the presence or absence of samples.
2.14. Protective effect against H2O2-induced cellular oxidative stress
Following the method described by Himaya et al. with some modifications,26 the EA.hy926 cells were seeded in 96-well plates with 5 × 103 cells per mL and incubated at 37 °C in 5% CO2 for 24 h. Then the cells were treated with various concentrations of test samples (0.25, 0.5 and 1.0 mg mL−1, dissolved in PBS) and incubated at 37 °C for 24 h, followed by treatment with 300 μM H2O2 for another 24 h. Cell viability was determined using the MTT method as described above. In the blank control, cells were treated only with the DMEM. In the H2O2 treated group (control group), cells were treated with 300 μM H2O2. In the sample group, cells were pretreated with different concentrations of samples then exposed to 300 μM H2O2. The protective effect of samples against H2O2-induced cellular oxidative stress was calculated as follows: |
Protective ability% = (AS − AC)/(AB − AC) × 100
| (2) |
where, AS was the absorbance at 490 nm of the sample group, AC was absorbance of the H2O2 treated group, and AB was the absorbance of blank control.
2.15. Statistical analysis
All the results were the means of triplicates. Data were subjected to analysis of variance and Duncan value with a confidence interval of 95% was calculated to compare means.
3. Results and discussion
3.1. Effect of enzymatic hydrolysis assisted with pretreatment on DH and ACE inhibition activity of CCG
As shown in Table 1, all the heating, ultrasound and HP pretreatment could effectively enhance the DH and ACE inhibition activity of CCGH (P < 0.05). HP may cause protein unfolding, which can enhance the susceptibility of proteins to enzymatic action by exposing new cleavage sites that allow enzymes to reach otherwise buried hydrolysis sites, thereby increasing the DH of CCG and release more peptides with ACE inhibition activity.27 Being heated in boiling water, some peptides chains of CCG may be degraded and some inner sites may be exposed, promoting the susceptibility of proteins to enzymatic action. Moreover, ultrasonic treatment may induce an easier dispersion and less agglomeration of CCG, which accelerated the mixing of substrate and proteases, resulting in an increase in DH. In this study, DH of CCGH without pretreatment (24.82 ± 2.26%) was higher that of coconut cake protein hydrolysates with papain (20.96 ± 0.52%),13 indicating that effect on CCG of mixture enzymes was better than that of single enzyme. Since the CCGH with HP pretreatment exhibited the highest DH and ACE inhibition activity, thus it was chose to separate and identify antihypertensive peptides.
3.2. Separation by ultrafiltration and Sephadex G-15 gel chromatography
Among the three fractions from ultrafiltration, CCGH-III exhibited a much higher ACE inhibition ability (58.37 ± 9.42%) than that of CCGH-I (14.79 ± 2.44%) and CCGH-II (24.06 ± 4.13%), which confirmed that protein hydrolysates with lower molecular mass exhibited stronger ACE inhibitory activity than large polypeptides.28 Through the Sephadex G-15 gel chromatography, CCGH-III was separated into five major fractions (Fig. 1a), of which fraction E (CCGH-E) showed the highest ACE inhibition activity at 1 mg mL−1 (Fig. 1b). It was chose to evaluate the in vivo antihypertensive effect.
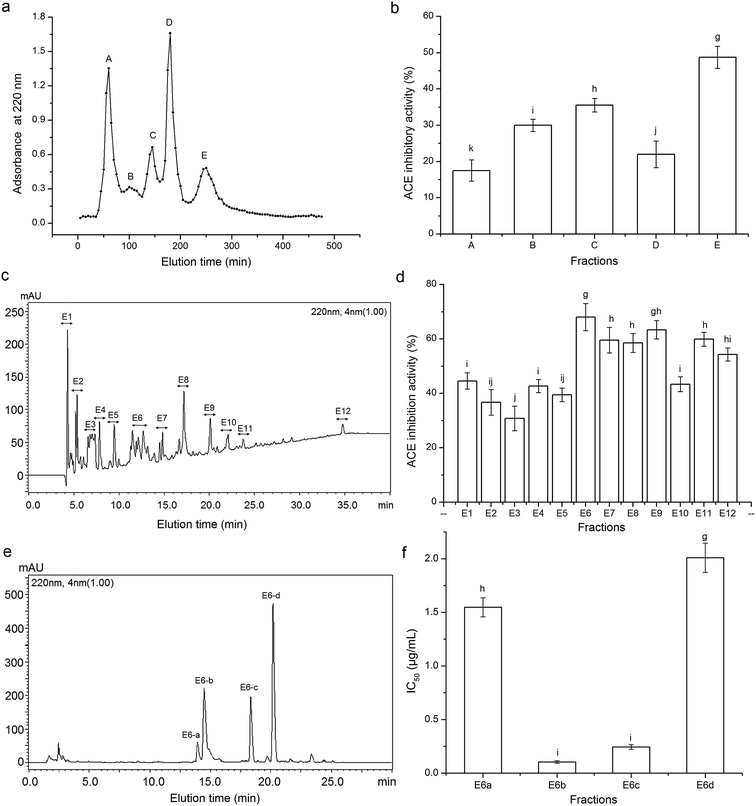 |
| Fig. 1 Sephadex G-25 gel chromatography profile of CCGH (a) and ACE inhibition activity of each fraction (b); chromatography of fraction E separated by semi-preparing RP-HPLC with the linear gradient of acetonitrile (5–30% in 30 min) containing 0.1% TFA at a flow rate of 2.5 mL min−1 (c); ACE inhibition ability of fractions E1-E12 (d); fraction E6 was isolated by analytical RP-HPLC at flow rate of 1 mL min−1 with the linear gradient of acetonitrile (5–25%, in 20 min) containing 0.1% TFA (e); and IC50 of fractions E6-a, E6-b, E6-c and E6-d on ACE inhibition activity (f). g–j different smaller letters on the bar meant significantly significance. | |
3.3. Antihypertensive effect in SHRs
As shown in Fig. 2A, the systolic blood pressure (SBP) in SHRs was lowered significantly (P < 0.05) from the second week of oral administration of CCGH-E (50, 100 and 200 mg kg−1). The middle dose and high dose of CCGH-E exhibited no significant difference in antihypertensive effect compared to captopril. Meanwhile, the diastolic blood pressure (SBP) of SHRs in middle- and high-dose group was also remarkably decreased (P < 0.05) after the second week of oral administration of CCGH-D (Fig. 2B). These results demonstrated that CCGH-E could play antihypertensive effect in vivo. Furthermore, there was a lower but no significant (P < 0.05) in body weight of SHRs in middle- and high-dose groups, compared to that in the negative group (Fig. 2C). Besides, SHRs in all the groups showed a normal range of HR from 6.44 to 8.57 Hz (Fig. 2D). It seemed that CCGH-E had no or very little effect on the gross metabolic conditions in SHRs.
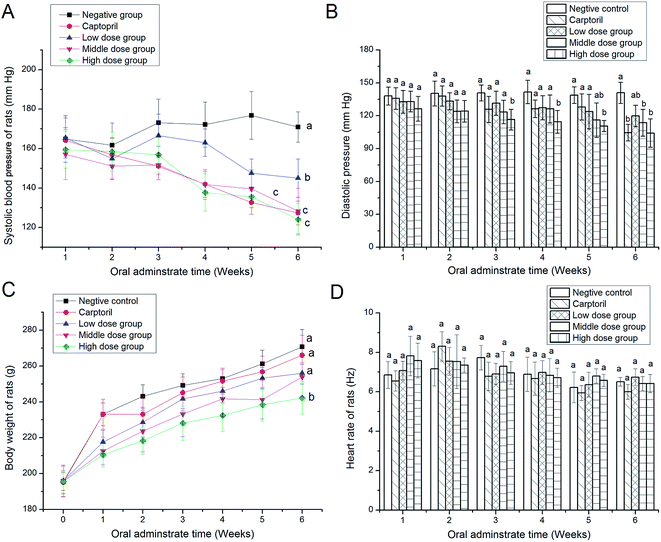 |
| Fig. 2 Time-course of changes in systolic blood pressure (A), diastolic blood pressure (B), body weight (C) and hear rate of spontaneous hypertensive rats (D), after single oral administration of fraction E isolated from coconut cake globulin hydrolysates (CCGH). Rats in negative control group were orally administered by a single dose of physiological saline (0.5 mL) once daily, whereas rats in positive control group received captopril at 14 mg per kg per body weight once daily. Rats in low-, middle- and high-dose group received PKEGH-E at 50, 100 and 200 mg per kg per bodyweight once daily, respectively. | |
3.4. Purification of the antihypertensive peptides
The fraction CCGH-E was further isolated by RP-HPLC on the semi-preparative C18 column and twelve major peaks named as E1–E12 were presented and collected (Fig. 1c and d). The fraction E6 with the lowest IC50 (0.852 ± 0.086 mg mL−1) was further purified by RP-HPLC in an analytical C18 column (Φ 4.6 mm × 250 mm). As shown in Fig. 1e, the fraction E6 was separated into four fractions named E6-a, E6-b, E6-c and E6-d. And the fraction E6-b and E6-c showed much higher ACE inhibitory activity (IC50: 0.104 ± 0.012 and 0.244 ± 0.020, respectively) than fraction E6-a and E6-d (IC50: 1.547 ± 0.089 and 2.009 ± 0.136 mg mL−1, respectively). Hence, the fraction E6-b and E6-c were rechromatographed to confirm their purity on the same analytical C18 column. Results in Fig. 3A and B showed that both fraction E6-b and E6-c showed a single peak, and they were subjected to ESI-MS/MS analysis.
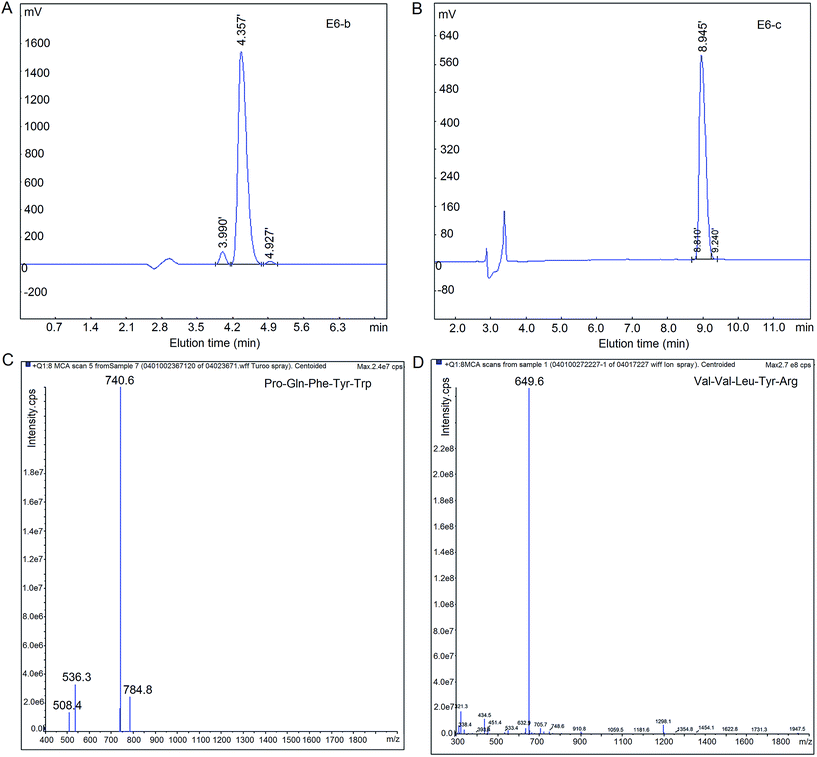 |
| Fig. 3 Chromatography of fraction E6-b (A) and E6-c (B) separated by analytical RP-HPLC with the linear gradient of acetonitrile (15–30%, in 12 min) containing 0.1% TFA at a flow rate of 1.0 mL min−1; and mass spectrums of peptides PQFYW (C) and VVLYR (D) identified by LC-MS/MS. | |
3.5. Characterization of purified peptides
As shown in Table 2, two peptides were identified by LC-MS/MS, of which Pro–Gln–Phe–Tyr–Trp was from fraction E6-b and Val–Val–Leu–Tyr–Arg was from fraction D6-c. Their mass spectrums by LC-ESI-MS/MS were shown in Fig. 3C and D. It was obvious that the identified peptides were all oligopeptides with 5 amino acid residues, in which hydrophobic residues were the predominant, corresponding to the report that the majority of ACE-inhibitory peptides were short sequences with 2–12 amino acids.5 Moreover, branched amino acid (Leu) and aromatic amino acids (Tyr and Phe) were the main in their C-terminal tripeptide. And Val–Val–Leu–Tyr–Arg had Arg residue at the C-terminal, may be attributed to the trypsin used during the preparation of CCGH. It was pointed out that trypsin prefer to hydrolyze peptide bond with Arg or Lys on the C-terminal end of the bond.6 Recent years, structure–activity studies on ACE inhibition peptides indicated that the C-terminal tripeptide residues played a predominant role in competitive binding to the active site of ACE.5 Gomez-Ruiz et al.29 have suggested that branched chain aliphatic amino acids may contribute significantly to increase ACE-inhibitory potential. In addition, the positive charge of Lys (ε-amino group) and Arg (guanidine group) as the C-terminal residue may contribute to the ACE inhibitory potency (Haque & Chand, 2008).30 Both the two identified peptides in this paper were in accordance with these structural features of ACE inhibitory peptides.
Table 2 ACE inhibitory peptides from fractions E6-b and E6-c identified by LC-MS/MS from coconut cake globulina and their stability against the simulated gastrointestinal digestionc
Fraction |
Peptide |
MW (Da) |
Matched sequence in Elaeis guineensisb |
Hydrophobic residues content |
IC50 (mg) |
IC50c (mg) |
Different small letters (a, b) in the same row means significant difference (P < 0.05). From National Center for Biotechnology Information (NCBI). Peptides were treated with pepsin and pancreatin. |
E6-b |
PQFYW Pro–Asn–Phe–Tyr–Trp |
739.84 |
K.PQFYW.R glutelin |
80.00% |
0.104 ± 0.012b |
0.116 ± 0.017b |
E6-c |
VVLYR Val–Val–Leu–Tyr–Arg |
648.81 |
R.VVLYR.N glutelin |
80.00% |
0.244 ± 0.026a |
0.257 ± 0.023a |
Furthermore, the peptide Pro–Gln–Phe–Tyr–Trp exhibited the highest ACE inhibition activity (0.104 ± 0.012 mg mL−1), in accordance with the report that the most effective ACE-inhibitory peptides contained aromatic amino acid in C-terminal tripeptide.5 Moreover, the IC50 of the two peptides (0.104 and 0.244 mg mL−1) was higher than that of peptides identified in protein hydrolysates from glycinin (Ser–Pro–Tyr–Pro, IC50: 0.098 mg mL−1), wheat gluten (Ile–Ala–Pro, IC50: 0.33 mg mL−1) and corn (Ala–Tyr, IC50: 0.037 mg mL−1);7–9 but much lower than that from skate skin (Gln–Leu–Gly–Phe–Leu–Gly–Pro–Arg, IC50: 1.39 mg mL−1), milk casein (Ala–Met–Pro–Lys–Pro–Trp, IC50: 1.84 mg mL−1) and pacific cod skin gelatin (Leu–Leu–Met–Leu–Asp–Asn–Asp–Leu–Pro–Pro, IC50: 1.84 mg mL−1).26,31,32 Accumulated findings suggested that apart from amino acid composition and sequence, the biologically active of hypertensive peptides was also affected by the molecular weight, processing method, food resources and so on.5
3.6. Stability and inhibition pattern of synthetic peptides
The ACE inhibition peptides identified in CCGH were synthesized to confirm their ACE inhibition activity and evaluate the stability against the gastrointestinal enzymes digestion. After the digestion of pepsin followed by pancreatin, there was no significant decrease (P < 0.05) in the ACE inhibition activity of the peptides PQFYW and VVLYR (Fig. 4), meaning that these peptides had good stability in gastrointestinal digestion system.
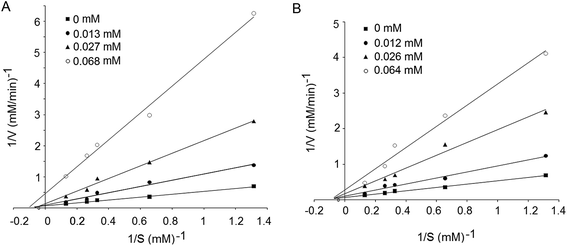 |
| Fig. 4 Lineweaver–Burk plot of the peptides identified in coconut cake globulin hydrolysates: PQFYW (A) and VVLYR (B). | |
ACE-inhibitory peptides were found to inhibit ACE via different inhibition modalities such as competitive, noncompetitive, uncompetitive and mixed inhibition manners. The kinetic constants of ACE in the presence of peptide Val–Val–Leu–Tyr–Arg revealed that, the Vmax decreased but Km was identical as the inhibitor concentration increased, which is the characteristic of noncompetitive inhibition modalities (Fig. 4B). However, in the presence of Pro–Gln–Phe–Tyr–Trp, Vmax decreased but Km changed differently (Fig. 3A), indicating a mixed modality of noncompetitive and uncompetitive inhibition. Similar peptides like EVSQGRP, SAAVGSP and VSRHFASYAN also exhibited mixed-mode inhibition patterns.33,34
3.7. Effect on EA.hy926 cells proliferation and the intracellular ET-1 content
Result in Fig. 5A revealed that the peptides PQFYW and VVLYR showed significant effect on the viability of EA.hy926 cells at high dose (1.0 mg mL−1, P < 0.05). Endothelial cells play an important role in balancing blood pressure, where ACE, ACE2 (angiotensin converting enzyme II, an antagonist of ACE), ET-1 are synthesized. Previous studies found that ACE inhibition peptides could play antihypertensive effect by affecting the proliferation of endothelial cells (Papine, 1997).35 Other than rennin angiotensin system (RAS), the endothelin system has an increasingly recognized role in blood pressure regulation, in which ET-1 has powerful vasoconstrictor and pressor properties. Thus, peptides could inhibit the form of intracellular ET-1 also had potential antihypertension.36 Results in Fig. 5B showed that the peptides PQFYW and VVLYR had significantly inhibition activity on the intracellular ET-1 content, indicating their potential antihypertensive effect (P < 0.05). Moreover, an obvious dose-dependent relationship was observed among the low-, medium- and high-dose groups. The highest inhibition ability was found on peptide PQFYW at 1.0 mg mL−1, in accordance with its highest ACE inhibitory activity as shown in Table 2. Liu et al.37 had demonstrated that peptides could play antihypertensive effect through inhibiting the expression of ET-1 in human umbilical vein cells.
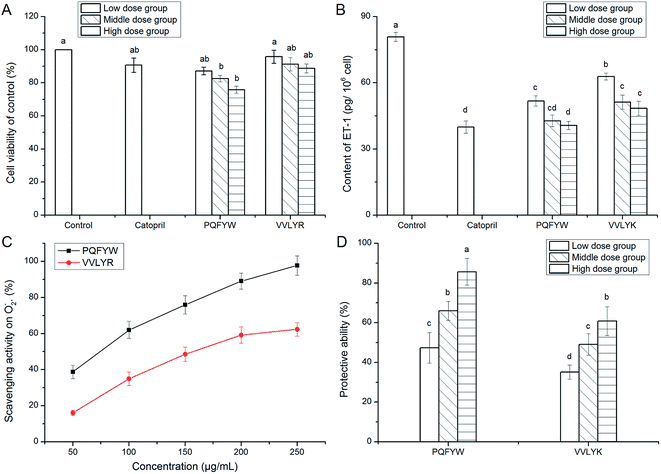 |
| Fig. 5 Effect of peptides identified in coconut cake globulin hydrolysates on the viability of EA.hy926 cells (A), and intracellular endothlin-1 content (B). The superoxide radical scavenging activity of the peptides (C); the protection of the peptides on EA.hy926 cells against damage induce by H2O2 (D). EA.hy926 cells in Low dose group, Middle dose group and High dose group were treated with 0.25, 0.5 and 1.0 mg mL−1 of peptides, respectively. Different small letters (a–d) on the bar meant significant difference (P < 0.05). | |
3.8. Superoxide radical-scavenging activity
As was depicted in Fig. 5C, both PQFYW and VVLYK exhibited good superoxide radical scavenging activity with a concentration-dependent increase. This was due to their hydrophobic amino acid and aromatic amino acid residues, for phenolic groups in aromatic amino acid could serve as hydrogen donors and inhibit the radical-mediated peroxidizing chain reaction.38 It was pointed that under high blood pressure, excessive superoxide radical would be produced and induced an injury to vascular endothelial cells, resulting in increase in intracellular ET-1 content, which may lead to a dysfunction in blood pressure regulation systems.36,39 Results in Fig. 5C meant that the two peptides could play antihypertensive effect through scavenging the excessive intracellular superoxide radical.
3.9. Protective effect against H2O2-induced cellular oxidative stress
More and more evidence demonstrated the relationship between hypertension and oxidative stress.4 H2O2 can trigger oxidative damage through the elevation of intracellular reactive oxygen species (ROS), in consequence may activate the cell apoptosis pathway and eventually lead to a variety of diseases including cancer, diabetes mellitus, cardiovascular diseases, etc.40 It was obvious that PQFYW and VVLYK had remarkably protection effect on EA.hy926 cells against H2O2 induced damage (Fig. 5D), and the peptide PQFYW showed the highest ability at concentration of 1.0 mg mL−1. Findings in Fig. 5C and D indicated that these peptides could exert antihypertensive effect through scavenging excessive free radicals and protect vascular endothelial cells from damage induced by excessive intracellular ROS.
4. Conclusions
Two novel ACE inhibitory peptides were identified in CCGH which was obtained by the sequential digestion of four enzymes assisted with HP pretreatment. The obtained peptides were non-competitive ACE inhibitor, comprised 5 amino acids with ACE inhibition IC50 values from 0.104 to 0.244 mg mL−1. And they had significant antihypertensive effect but little side-effect in SHRs. Moreover, these peptides could exert antihypertensive ability through reducing the intracellular ET-1 content, and/or through protecting vascular endothelial cells from reactive oxygen species mediated damage. Therefore, the peptides isolated from CCG could be used as a functional food ingredient to control ACE activity.
Acknowledgements
This work was supported by program of Science and Technology of 12th Five-year Plan (No. 2012BAD31B03).
References
- L. Lin, S. Lv and B. F. Li, Angiotensin-I-converting enzyme (ACE)-inhibitory and antihypertensive properties of squid skin gelatin hydrolysates, Food Chem., 2012, 131, 225–230 CrossRef CAS.
- H. Yoshii, N. Tachi, R. Ohba, O. Sakamura, H. Takeyama and T. Itani, Antihypertensive effect of ACE inhibitory oligopeptides from chicken egg yolks, Comp. Biochem. Physiol., Part C: Toxicol. Pharmacol., 2001, 128, 27–33 CrossRef CAS.
- E. L. Schiffrin, Vascular endothelin in hypertension, Vasc. Pharmacol., 2005, 43, 19–29 CrossRef CAS PubMed.
- B. J. Bhuyan and G. Mugesh, Synthesis, characterization and antioxidant activity of angiotensin I converting enzyme inhibitors, Org. Biomol. Chem., 2011, 9, 1356–1365 CAS.
- B. Hernández-Ledesma, M. M. Contreras and I. Recio, Antihypertensive peptides: Production, bioavailability and incorporation into foods, Adv. Colloid Interface Sci., 2011, 165, 23–25 CrossRef PubMed.
- R. J. Fitzgerald, B. A. Murray and D. J. Walsh, Hypotensive peptides from milk proteins, J. Nutr., 2004, 134, 980–988 Search PubMed.
- M. Kuba, C. Tana, S. Tawata and M. Yasuda, Production of angiotensin I-converting enzyme inhibitory peptides from soybean protein with Monascus purpureus acid proteinase, Process Biochem., 2005, 40, 2191–2196 CrossRef CAS.
- B. G. Thewissen, A. Pauly, I. Celus, K. Brijs and J. A. Delcour, Inhibition of angiotensin I-converting enzyme by wheat gliadin hydrolysates, Food Chem., 2011, 127, 1653–1658 CrossRef CAS.
- F. Lin, L. Chen, R. Liang, Z. Zhang, J. Wang, M. Cai and Y. Li, Pilot-scale production of low molecular weight peptides from corn wet milling byproducts and the antihypertensive effects in vivo and in vitro, Food Chem., 2011, 124, 801–807 CrossRef CAS.
- S. Thaiphanit and P. Anprung, Physicochemical and emulsion properties of edible protein concentrate from coconut (Cocos nucifera L.) processing by-products and the influence of heat treatment, Food Hydrocolloids, 2016, 52, 756–765 CrossRef CAS.
- M. R. N. Angelia, R. N. Garcia, K. M. P. Caldo, K. Prak, S. Utsumi and E. M. Tecson-Mendoza, Physicochemical and functional characterization of cocosin, the coconut 11S globulin, Food Sci. Technol. Int., 2010, 16(3), 225–232 CrossRef CAS PubMed.
- S. Mini and T. Rajamohan, Influence of coconut kernel protein on lipid metabolism in alcohol fed rats, Indian J. Exp. Biol., 2004, 42, 53–57 CAS.
- Y. J. Zheng, Y. Li, Y. L. Zhang and S. L. Zhao, Purification, characterization and synthesis of antioxidant peptides from enzymatic hydrolysates of coconut (Cocos nucifera L.) cake protein isolates, RSC Adv., 2016, 6, 54346–54356 RSC.
- H. X. Wang and T. B. Ng, An antifungal peptide from the coconut, Peptides, 2005, 26, 2392–2396 CrossRef CAS PubMed.
- S. M. Mandal, S. Dey, M. Mandal, S. Sarkar, S. Maria-Neto and O. L. Franco, Identification and structural insights of three novel antimicrobial peptides isolated from green coconut water, Peptides, 2009, 30, 633–637 CrossRef CAS PubMed.
- M. J. Santana, A. L. D. Olivera, L. H. K. Q. Júnior, S. M. Mandal, C. O. Matos, R. D. Dias, O. L. Franco and L. M. Liao, Structural insights into Cn-AMP1, a short disulfide-free multifunctional peptide from green coconut water, FEBS Lett., 2015, 589, 639–644 CrossRef CAS PubMed.
- S. Thaiphanit and P. Anprung, Physicochemical and emulsion properties of edible protein concentrate from coconut (Cocos nucifera L.) processing by-products and the influence of heat treatment, Food Hydrocolloids, 2016, 52, 756–765 CrossRef CAS.
- Y. Zhang, K. Olsen, A. Grossi and J. Otte, Effect of pretreatment on enzymatic hydrolysis of bovine collagen and formation of ACE-inhibitory peptides, Food Chem., 2013, 141, 2343–2354 CrossRef CAS PubMed.
- J. Adler-Nissen, Determination of the degree of hydrolysis
of food protein hydrolysates by trinitrobenzenesulfonic acid, J. Agric. Food Chem., 1979, 27, 1256–1262 CrossRef CAS PubMed.
- V. K. Jimsheena and L. R. Gowda, Colorimetric, high-throughput assay for screening angiotensin I-converting enzyme inhibitors, Anal. Chem., 2009, 81, 9388–9394 CrossRef CAS PubMed.
- K. Bush, P. R. Henry and D. S. Slusarchyk, Muraceins-muramyl peptides produced by Norcardia orientalis as angiotensin converting enzyme inhibitors, J. Antibiot., 1984, 37, 330–335 CrossRef CAS PubMed.
- K. S. Oh, W. Han, M. H. Wang and B. H. Lee, The effects of chronic treatment with Morus bombycis KOIDZUMI in spontaneously hypertensive rats, Biol. Pharm. Bull., 2007, 30, 1278–1283 CAS.
- T. Tavares, M. D. Contreras, M. Amorim, M. Pintado, I. Recio and F. X. Malcata, Novel whey-derived peptides with inhibitory effect against angiotensin-converting enzyme: In vitro effect and stability to gastrointestinal enzymes, Peptides, 2011, 32, 1013–1019 CrossRef CAS PubMed.
- T. Mosmann, Rapid colorimetric assay for cellular growth and survival: application to proliferation and cytotoxicity assays, J. Immunol. Methods, 1983, 65, 55–63 CrossRef CAS PubMed.
- S. Marklund and G. Marklund, Involvement of the superoxide anion radical in the autoxidation of pyrogallal and a convenient assay for superoxide dismutase, Eur. J. Biochem., 1974, 47, 469–474 CrossRef CAS PubMed.
- S. W. A. Himaya, D. H. Ngo, B. Ryu and S. K. Kim, An active peptide purified from gastrointestinal enzyme hydrolysate of Pacific cod skin gelatin attenuates angiotensin-1 converting enzyme (ACE) activity and cellular oxidative stress, Food Chem., 2012, 132, 1872–1882 CrossRef CAS.
- M. J. Eisenmenger and J. I. Reyes-De-Corcuera, High pressure enhancement of enzymes: a review, Enzyme Microb. Technol., 2009, 45, 331–347 CrossRef CAS.
- J. B. Jeong, B. O. De Lumen and H. J. Jeong, Lunasin peptide purified from Solanum nigrum L. protects DNA from oxidative damage by suppressing the generation of hydroxyl radical via blocking fenton reaction, Cancer Lett., 2010, 293, 58–64 CrossRef CAS PubMed.
- J. A. Gomez-Ruiz, M. Ramos and I. Recio, Angiotensin converting enzyme-inhibitory activity of peptides isolated from Manchego cheese. Stability under simulated gastrointestinal digestion, Int. Dairy J., 2004, 14, 1075 CrossRef.
- E. Haque and R. Chand, Antihypertensive and antimicrobial bioactive peptides from milk proteins, Eur. Food Res. Technol., 2008, 227, 7–15 CrossRef CAS.
- J. K. Lee, J. K. Jeon and H. G. Byun, Effect of angiotensin I converting enzyme inhibitory peptide purified from skate skin hydrolysate, Food Chem., 2011, 125, 495–499 CrossRef CAS.
- H. Karaki, K. Doi, S. Sugano, H. Uchiwa, R. Sugai and U. Murakami, et al., Antihypertensive effect of tryptic hydrolysate of milk casein in spontaneously hypertensive rats, Comp. Biochem. Physiol., C: Comp. Pharmacol., 1990, 96, 367 CrossRef CAS.
- B. Forghani, M. Zarei, A. Ebrahimpour, R. Philip, J. Bakar, A. Abdul-Hamid and N. Saari, Purification and characterization of angiotensin converting enzyme-inhibitory peptides derived from Stichopus horrens: Stability study against the ACE and inhibition kinetics, J. Funct. Foods, 2016, 20, 276–290 CrossRef CAS.
- G. Masuyer, S. L. U. Schwager, E. D. Sturrock, R. E. Isaac and K. R. Acharya, Molecular recognition and regulation of human angiotensin-I converting enzyme (ACE) activity by natural inhibitory peptides, Sci. Rep., 2012, 2, 717 Search PubMed.
- C. J. Papine, Improved endothelial function with angiotensin-converting enzyme inhibitors, Am. J. Cardiol., 1997, 79, 29–32 CrossRef.
- S. M. Wallace, L. Yasmin, C. M. McEniery, M. Makj-Petaja, A. D. Booth, J. R. Cockcroft and L. B. Wilkinson, et al., Isolated systolic hypertension is characterized by increased aortic stiffness and endothelial dysfunction, Hypertension, 2007, 50, 228–233 CrossRef CAS PubMed.
- D. Liu, L. Zhang, S. Li, F. Liu and S. Z. Liang, Influence of angiotensin-converting enzyme inhibitory peptide on endothelial cell proliferation and endothelin expression in human umbilical vein cells, Chin. J. Clin. Rehabil., 2006, 10(25), 160–163 CAS.
- B. H. Sarmadi and A. Ismail, Antioxidative peptides from food proteins: a review, Peptides, 2010, 31, 1949–1956 CrossRef CAS PubMed.
- A. Rekhviashvili, B. Tsinamdzgvrishvili and M. Chkhetia, The relationship between endothelial dysfunction and 242 hour blood pressure rhythm in patients with arterial hypertension, Georgian Med. News, 2008, 155, 13217 Search PubMed.
- R. J. Elias, S. S. Kellerby and E. A. Decker, Antioxidant activity of proteins and peptides, Crit. Rev. Food Sci. Nutr., 2008, 48, 430–441 CrossRef CAS PubMed.
|
This journal is © The Royal Society of Chemistry 2016 |
Click here to see how this site uses Cookies. View our privacy policy here.