DOI:
10.1039/C6RA19885F
(Review Article)
RSC Adv., 2016,
6, 100585-100589
Enhanced microwave absorbing properties of PANI/CoFe2O4/PVDF composite
Received
6th August 2016
, Accepted 10th October 2016
First published on 10th October 2016
Abstract
Polyaniline/CoFe2O4/polyvinylidene fluoride (PANI/CoFe2O4/PVDF) composites were synthesized by an in situ polymerization method. The electromagnetic data demonstrate that the PANI/CoFe2O4/PVDF composite exhibit an excellent microwave absorption in the range of 2.0–18.0 GHz. The maximum reflection loss (RL) value can reach −57.7 dB at 7.6 GHz with a thickness of 4 mm and the absorption bandwidth with the RL below −10 dB is up to 3.4 GHz (from 6.5 to 9.9 GHz), which indicates that the microwave absorbing properties of the PANI/CoFe2O4 composite can be enhanced by the introduction of PVDF and the PANI/CoFe2O4/PVDF composite has potential applications for high-efficient microwave absorbers.
1. Introduction
With the development of electronic technology, electromagnetic wave absorbing materials have attracted much attention because of prospective applications in both civil and military fields.1–4 According to the electromagnetic wave energy conversion principle, the proper impedance matching between dielectric loss and magnetic loss determines the reflection and attenuation characteristics of electromagnetic wave absorbers. The attenuation mechanism of electromagnetic wave absorbing materials is mainly based on high magnetic loss and high dielectric loss.5–9 Therefore, ferrite and conducting polymers have been developed to attenuate electromagnetic wave.10–12 CoFe2O4 is a spinel ferrite with a high saturation magnetization and a high magnetic loss. PANI, as a conducting polymer, shows a high electric conductivity and is a very promising electromagnetic absorber. However, when pure PANI or CoFe2O4 is independently used for microwave absorbing materials, their attenuation mechanism is not able to achieve the impedance matching between dielectric loss and magnetic loss, which restricts the microwave absorbing abilities.13–15 Recently, many studies have been carried out to investigate the PANI/CoFe2O4 composite to further improve the microwave absorbing properties.16–20 Nonetheless, some electromagnetic waves would be reflected before penetrating the PANI/CoFe2O4 composite. The reports on the introduction of electromagnetic transparent materials to improve the microwave absorbing properties of PANI/CoFe2O4 composite are quite scarce.
In this article, PVDF was introduced as an electromagnetic transparent component to prepare the PANI/CoFe2O4/PVDF composite due to PVDF is a typical electromagnetic transparent material. A synergetic effect between magnetic nanometers and PVDF is also beneficial to the absorption rate.21–23 Moreover, the introduction of PVDF would also improve the impedance matching because PVDF is a kind of ferroelectric polymer.24,25
2. Experimental
PVDF was provided by Alfa Aesar Chemical Company Limited. Cobalt ferrite (99.5%) was purchased from Shanghai Aladdin Biological Technology Company. Besides, ammonium persulphate (APS), hydrochloric acid (HCl) and aniline were purchased from Sinopharm Chemical Reagent Company Limited, China. Aniline was distilled at reduced pressure before being used. Deionized water was used for all the experiments.
The PANI/CoFe2O4 composite and the PANI/CoFe2O4/PVDF composite were prepared by an in situ polymerization method. According to different mass ratios, CoFe2O4 powder and PVDF powder were dispersed in a 2 M HCl solution, which were placed into a boiling flask-3-neck for 30 min with stirring. And then the temperature of the mixture was decreased down to 0 °C and some monomer aniline was added into the boiling flask-3-neck. The solution of (NH4)2S2O8 was added dropwisely to initiate the polymerization with the molar ratio of (NH4)2S2O8 to aniline of 1
:
1. The reaction was carried out at 0 °C for 8 h. Finally the PANI/CoFe2O4 composite and the PANI/CoFe2O4/PVDF composite were obtained by filtration, washing several times with ultrapure water and drying in vacuum at 60 °C for 10 h. PVDF was provided by Alfa Aesar (China) Chemical Company Limited. Besides, all the above reagents were purchased from Sinopharm Chemical Reagent Company Limited, China.
The compound structure of the samples was obtained with a Fourier transform infrared spectrometer (FTIR, Bruker Vector-22, Germany). The phase identification of the samples was performed with an X-ray diffractometer (Rigaku, D/MAX-2400, Japan). The scanning electron microscope (SEM, Hitachi S-4800, Japan) and the transmission electron microscopy (TEM, FEI TecnaiG2F20S-TWIN, USA) were employed to analyze the morphology of the samples. The magnetic hysteresis loops of the composite powder were measured by a vibrating sample magnetometer (Lake Shore, 7410, USA). The electromagnetic parameters of the samples were measured by a HP8720ES vector network analyzer.
3. Results and discussion
3.1 FTIR spectra
The FTIR spectra of PANI/CoFe2O4 composite, PANI/CoFe2O4/PVDF composite, PVDF, PANI and CoFe2O4 powders are shown in Fig. 1. For CoFe2O4 powder, it can be seen that the peak at 592 cm−1 is the characteristic band of Fe–O band stretching modes of tetrahedral and octahedral sites as a normal spinel structure.26,27 The spectrum of pure PANI reveals that the characteristic bands at 1575 cm−1 and 1480 cm−1 are attributed to the characteristic C
C stretching of the quinoid and benzenoid rings while the peaks at 1293 cm−1 is assigned to the C–N stretching of the secondary aromatic amine. The bands at 1110 cm−1 and 792 cm−1 can be ascribed to out-of-plane bending of C–H.28–32 The peaks at 1575, 1480 and 1293 cm−1 hypsochromically shift to 1577, 1482 and 1295 cm−1 in the PANI/CoFe2O4 composite compared with PANI, suggesting the successful coating of PANI on the surfaces of CoFe2O4 particles. The hypsochromic shift of the vibrations might be ascribed to the π–π interactions between the PANI molecules and CoFe2O4.33 The pure PVDF has the CH symmetric and CH2 asymmetric vibration at 3026 cm−1, 2980 cm−1 and 1389 cm−1, and CF2 deformation and stretching vibrations at 1241 cm−1 and 1066 cm−1.34 For the PANI/CoFe2O4/PVDF composite the characteristic peaks at 1556, 1473 and 1137 cm−1 are ascribed to C
C stretching vibration in quinoid ring and benzenoid rings of PANI, whereas the bands at 1279 cm−1 is attributed to the C–N stretching modes.30–32 In addition, it is clear that the PANI/CoFe2O4/PVDF composite displays greater bathochromic shifts, which demonstrates that PVDF has been covalently bonded onto PANI and CoFe2O4.33–36
 |
| Fig. 1 FTIR spectra of (a) PVDF, (b) PANI/CoFe2O4/PVDF composite, (c) PANI/CoFe2O4 composite, (d) PANI, (e) CoFe2O4 powder. | |
3.2 XRD analysis
The crystal structures of PANI/CoFe2O4/PVDF composite, PANI/CoFe2O4 composite, PVDF, PANI and CoFe2O4 were characterized using XRD, as displayed in Fig. 2. It is known that the structure of PANI is predominately amorphous. The broad amorphous diffraction peaks centered at 2θ of 25.3° and 27.5° are discovered, corresponding to the parallel and perpendicular periodicity of the PANI chains. For CoFe2O4, diffraction peaks can be perfectly assigned to (111), (220), (311), (222), (400), (331), (422) and (511) planes of the cubic spinel CoFe2O4 (JCPDS no. 22-1086). The peaks at 18.4°, 20° and 26.5° in all the samples correspond to α-phase PVDF crystal.37 As for the PANI/CoFe2O4 composite and PANI/CoFe2O4/PVDF composite, the characteristic peaks of corresponding constituents can be easily found. These results indicate that the PANI/CoFe2O4 composite and the PANI/CoFe2O4/PVDF composite have been successfully obtained.
 |
| Fig. 2 XRD patterns of (a) PVDF, (b) PANI/CoFe2O4/PVDF composite, (c) PANI/CoFe2O4 composite, (d) CoFe2O4 powder, (e) PANI. | |
3.3 Morphology analysis
The SEM and TEM images of PANI/CoFe2O4 composite and PANI/CoFe2O4/PVDF composite are shown in Fig. 3. From Fig. 3a, it can be found that the surface of PANI/CoFe2O4 composite is rough and the spinel contour of the CoFe2O4 in the bi-phase composite is vague, indicating that the CoFe2O4 particles are coated by PANI, and an excellent adhesion between PANI and CoFe2O4 can be obtained. From Fig. 3b it can be seen that the PANI/CoFe2O4 hybrids have been completely coated by PVDF. In order to illustrate the internal structure of PANI/CoFe2O4/PVDF composite, the image of PANI/CoFe2O4/PVDF composite is presented in Fig. 3c and e. From Fig. 3c and e, it can be found that in the PANI/CoFe2O4/PVDF composite PANI and CoFe2O4 are evenly distributed in PVDF. To verify the crystalline structure of CoFe2O4, the HRTEM image of PANI/CoFe2O4/PVDF composite is shown in Fig. 3d. The lattice fringes of the particles can be found in the HRTEM image of a part of the cubic phase, corresponding to the lattice spacing of d(311) = 0.25 nm. In addition, the SAED pattern (Fig. 3f) of particles can be well indexed to the (311) crystal planes of pure phase of CoFe2O4. This further confirms the existence of CoFe2O4 crystals in the PANI/CoFe2O4/PVDF composite, which is consistent with the XRD results.
 |
| Fig. 3 The SEM and TEM images of (a) PANI/CoFe2O4 composite, (b) PANI/CoFe2O4/PVDF composite, (c) and (e) TEM micrographs of PANI/CoFe2O4/PVDF composite, (d) HRTEM image and (f) SAED patterns of PANI/CoFe2O4/PVDF composite. | |
3.4 Magnetic property
The hysteresis loop was measured using VSM to verify the magnetic properties of the samples. The hysteresis loops of CoFe2O4 powder, PANI/CoFe2O4 composite and PANI/CoFe2O4/PVDF composite are shown in Fig. 4. The saturated magnetization (Ms) and coercive force (Hc) data of CoFe2O4 powder, PANI/CoFe2O4 composite and PANI/CoFe2O4/PVDF composite obtained from Fig. 4 are listed in Table 1. Due to the fact that PANI and PVDF are non-magnetic the Ms and Mr value of PANI/CoFe2O4 composite and PANI/CoFe2O4/PVDF composite are lower than those of pure CoFe2O4 powder. There is no obvious difference of Hc values between CoFe2O4 powder, PANI/CoFe2O4 composite and PANI/CoFe2O4/PVDF composite.
 |
| Fig. 4 Magnetization curves of CoFe2O4 powder, PANI/CoFe2O4 composite and PANI/CoFe2O4/PVDF composite. | |
Table 1 Magnetic properties of the samples
Sample |
Hc (Oe) |
Ms (emu g−1) |
Mr (emu g−1) |
CoFe2O4 |
1705 |
33.6 |
15.2 |
PANI/CoFe2O4 composite |
1709 |
15.9 |
7.9 |
PANI/CoFe2O4/PVDF composite |
1709 |
10.1 |
4.9 |
3.5 Microwave absorbing properties
To reveal the microwave absorbing properties of PANI/CoFe2O4 composite and PANI/CoFe2O4/PVDF composite, the reflection loss (RL) can be calculated from the measured complex relative permittivity and permeability data for the absorber thickness and given frequency by the following equations: |
 | (1) |
|
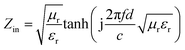 | (2) |
where Zin is the input impedance of the absorber. εr and μr are the complex permittivity and permeability of the absorber respectively. c is the velocity of electromagnetic waves in free space and f is the frequency. d is the thickness of the absorber.
The calculated reflection losses at different thicknesses of pure CoFe2O4 powder, PANI/CoFe2O4 composite and PANI/CoFe2O4/PVDF composite are shown in Fig. 5. In Fig. 5a, the maximum RL of pure CoFe2O4 powder is −14.2 dB at 11.3 GHz. For the PANI/CoFe2O4 composite in Fig. 5b it is clearly seen that the maximum RL is −20.2 dB at 8.3 GHz with the thickness of 3 mm, and the absorption bandwidth with the RL below −10 dB is up to 3 GHz. With the introduction of PVDF, the electromagnetic absorbing properties of PANI/CoFe2O4 composite could be remarkably improved. As shown in Fig. 5c, the maximum RL of PANI/CoFe2O4/PVDF composite is −57.7 dB at 7.6 GHz with the thickness of 4 mm, and the absorption bandwidth with the RL below −10 dB is up to 3.4 GHz. Electromagnetic parameters such as complex permittivity (ε′ and ε′′), complex permeability (μ′ and μ′′), of CoFe2O4 powder, PANI/CoFe2O4 composite and PANI/CoFe2O4/PVDF composite were measured. The results are illustrated in Fig. 6. Fig. 6a shows the real and imaginary permittivity and permeability in the range of 2–18 GHz for (a) pure CoFe2O4 powder, (b) PANI/CoFe2O4 composite and (c) PANI/CoFe2O4/PVDF composite, respectively. From Fig. 6a, it can seen that the introduction of PANI can significantly increase the ε′ and ε′′ values of the composites due to the high electrical conductivity of PANI, which is beneficial to the enhancement of microwave absorbing property. Compared with the PANI/CoFe2O4 composite, the ε′′ and μ′′ values of PANI/CoFe2O4/PVDF composite is more close to each other. The PANI/CoFe2O4/PVDF composite could have a better impedance match between the dielectric loss and the magnetic loss, which leads to more excellent electromagnetic wave absorbing properties. For better comparison, the microwave absorbing properties of PANI/CoFe2O4/PVDF composite and other reported CoFe2O4-based composites are shown in Table 2. To our knowledge, the microwave absorbing properties for this work is much enhanced compared with previously documented CoFe2O4-based composites, which implies that the PANI/CoFe2O4/PVDF composite is very promising to be used as a microwave absorbing material.
 |
| Fig. 5 Reflection loss (RL) in 2–18 GHz for (a) pure CoFe2O4 powder, (b) PANI/CoFe2O4 composite and (c) PANI/CoFe2O4/PVDF composite with different thicknesses. | |
 |
| Fig. 6 Frequency dependences of the electromagnetic parameters of the powder prepared: (a) pure CoFe2O4 powder, (b) PANI/CoFe2O4 composite, (c) PANI/CoFe2O4/PVDF composite. | |
Table 2 Comparison of the microwave absorbing properties of PANI/CoFe2O4/PVDF composite and other reported CoFe2O4-based composites
Sample |
Minimum RL value (dB) |
Optimum thickness (mm) |
Optimum frequency (GHz) |
Frequency range (RL <−10 dB) |
Ref. |
Polyaniline/CoFe2O4 nano-composite |
−16.71 dB |
2 mm |
16.01 |
4.68 |
19 |
Graphene@CoFe2O4@polyaniline nanocomposites |
−47.7 dB |
1.6 mm |
14.9 GHz |
3.7 GHz |
20 |
PANI/CoFe2O4/PVDF composite |
−57.7 dB |
4 mm |
7.6 GHz |
3.4 GHz |
This work |
4. Conclusions
The PANI/CoFe2O4/PVDF composite with enhanced microwave absorbing properties have been successfully synthesized by the in situ polymerization method. FTIR, XRD, SEM and TEM studies show that there is a good wrapping of CoFe2O4 with PANI and PVDF. The microwave absorbing properties show that the maximum RL of the PANI/CoFe2O4 composite is −20.2 dB at 8.3 GHz with the thickness of 3 mm, and the absorption bandwidth with the RL below −10 dB is up to 2.7 GHz. However, the maximum RL of the PANI/CoFe2O4/PVDF composite is −57.7 dB at 7.6 GHz with the thickness of 4 mm, and the absorption bandwidth with the RL below −10 dB is up to 3.4 GHz. The results indicate that PANI/CoFe2O4/PVDF composite may be an attractive candidate material for microwave absorption.
Acknowledgements
This work is supported by the National Natural Science Foundation of China (Grant No. 51572159), the Chinese Postdoctoral Science Foundation (Grant No. 2016M590916), the Scientific Research Foundation for the Returned Overseas Chinese Scholars, State Education Ministry, the Science and Technology Foundation of Weiyang District of Xi'an City (Grant No. 201605), the Industrialization Foundation of Education Department of Shaanxi Provincial Government (Grant No. 16JF002).
References
- J. Huo, L. Wang and H. J. Yu, J. Mater. Sci., 2009, 44, 3917–3927 CrossRef CAS.
- X. Guo, Y. Deng, D. Gu, R. Che and D. Zhao, J. Mater. Chem., 2009, 19, 6706–6712 RSC.
- W. L. Song, M. S. Cao, B. Wen, Z. L. Hou, J. Cheng and J. Yuan, Mater. Res. Bull., 2012, 47, 1747–1754 CrossRef CAS.
- A. Verma, A. K. Saxena and D. C. Dube, J. Magn. Magn. Mater., 2003, 263, 228–234 CrossRef CAS.
- N. Chen, K. Yang, M. Gu, N. Chen and K. Yang, J. Alloys Compd., 2010, 490, 609–612 CrossRef CAS.
- R. Che, L. M. Peng, X. Duan, Q. Chen and X. Liang, Adv. Mater., 2004, 16, 401–405 CrossRef CAS.
- S. He, G. S. Wang, C. Lu, J. Liu, B. Wen, H. Liu, L. Guo and M. S. Cao, J. Mater. Chem. A, 2013, 1, 4685–4692 CAS.
- G. S. Wang, Y. Y. Wu, X. J. Zhang, Y. Li, L. Guo and M. S. Cao, J. Mater. Chem. A, 2014, 2, 8644–8651 CAS.
- X. J. Zhang, G. S. Wang, W. Q. Cao, Y. Z. Wei, J. F. Liang, L. Guo and M. S. Cao, ACS Appl. Mater. Interfaces, 2014, 6, 7471–7478 CAS.
- J. W. Liu, R. C. Che, H. J. Chen, F. Zhang, F. Xia, Q. S. Wu and M. Wang, Small, 2012, 8, 1214–1221 CrossRef CAS PubMed.
- H. John, R. M. Thomas, J. Jacob, K. T. Mathew and R. Joseph, Polym. Compos., 2007, 28, 588–592 CrossRef CAS.
- P. Chandrasekhar, Conducting Polymers, Fundamentals and Applications, Springer, USA, 2005 Search PubMed.
- M. Oyharçabala, T. Olingaa, M.-P. Foulca, S. Lacommeb, E. Gontierb and V. Vigneras, Compos. Sci. Technol., 2012, 74, 107–112 CrossRef.
- S. M. Abbas, A. K. Dixit, R. Chatterjee and T. C. Goel, J. Magn. Magn. Mater., 2007, 309, 20–24 CrossRef CAS.
- S. M. Abbas, M. Chandra, A. Verma, R. Chatterjee and T. C. Goel, Composites, Part A, 2006, 37, 2148–2154 CrossRef.
- J. A. Khan, M. Qasim, B. R. Singh, S. Singha, M. Shoeba, W. Khana, D. Dasb and A. H. Naqvia, Spectrochimica Acta Part A Molecular & Biomolecular Spectroscopy, 2013, 109, 313–321 CAS.
- N. Gandhi, K. Singh, A. Ohlan, D. P. Singh and S. K. Dhawan, Compos. Sci. Technol., 2011, 71, 1754–1760 CrossRef CAS.
- B. Belaabed, J. L. Wojkiewicz, S. Lamouri, N. Kamchi and T. Lasri, J. Alloys Compd., 2012, 527, 137–144 CrossRef CAS.
- J. Jiang, C. Yang, H. Wang, X. H. Liu and L. H. Li, Chem. Res. Chin. Univ., 2014, 35, 402–408 CAS.
- P. Liu, Y. Huang and X. Zhang, Synth. Met., 2015, 201, 76–81 CrossRef CAS.
- X. Bai, Y. Zhai and Y. Zhang, J. Phys. Chem. C, 2011, 115, 11673–11677 CAS.
- L. Yang, S. L. Phua and C. L. Toh, RSC Adv., 2013, 3, 6377–6385 RSC.
- Z. Liu, G. Bai, Y. Huang, F. F. Li, Y. F. Ma, T. Y. Guo, X. B. He, X. Lin, H. J. Gao and Y. S. Chen, J. Phys. Chem. C, 2007, 111, 13696–13700 CAS.
- V. Eswaraiah, V. Sankaranarayanan and S. Ramaprabhu, Macromol. Mater. Eng., 2011, 296, 894–898 CrossRef CAS.
- Y. Shen, Y. Guan, Y. Hu, Y. C. Lei, Y. Song, Y. H. Lin and C. W. Nan, Appl. Phys. Lett., 2013, 103, 072906–072910 CrossRef.
- E. E. Tanrverdi, A. T. Uzumcu and H. Kavas, Micro Nano Lett., 2011, 3, 99–107 Search PubMed.
- A. Baykal, N. Kasapoğlu, Y. Köseoğlu, A. C. Başaran, H. Kavas and S. M. Toprak, Cent. Eur. J. Chem., 2008, 6, 125–130 CAS.
- Y. G. Wang, H. Q. Li and Y. Y. Xia, Adv. Mater., 2006, 18, 2619–2623 CrossRef CAS.
- R. K. Pandey and V. Lakshminarayanan, J. Phys. Chem. C, 2009, 13, 21596–21603 Search PubMed.
- C. C. Hu and J. Y. Lin, Electrochim. Acta, 2002, 47, 4055–4067 CrossRef CAS.
- N. L. Yang, J. Zhai, M. X. Wan, D. Wang and L. Jiang, Synth. Met., 2010, 160, 1617–1622 CrossRef CAS.
- J. T. Zhang and X. S. Zhao, J. Phys. Chem. C, 2012, 116, 5420–5426 CAS.
- Z. F. Li, H. Y. Zhang, Q. Liu and Y. D. Liu, Carbon, 2014, 71, 257–267 CrossRef CAS.
- X. J. Zhang, G. S. Wang, W. Q. Cao, Y. Z. Wei, M. S. Cao and L. Guo, RSC Adv., 2014, 4, 19594–19601 RSC.
- R. J. Tseng, C. O. Baker, B. Shedd and J. X. Huang, Appl. Phys. Lett., 2007, 90, 053101–053103 CrossRef.
- Z. F. Li, D. B. Frank, M. F. Bertinoc and C. S. Kimd, Sens. Actuators, B, 2012, 161, 390–395 CrossRef CAS.
- G. T. Davis, J. E. Mckinney, M. G. Broadhurst and S. C. Roth, J. Appl. Phys., 1978, 49, 4998–5002 CrossRef CAS.
|
This journal is © The Royal Society of Chemistry 2016 |
Click here to see how this site uses Cookies. View our privacy policy here.