DOI:
10.1039/C6RA19531H
(Paper)
RSC Adv., 2016,
6, 92510-92519
Improvement of corrosion resistance and magnetic properties for sintered NdFeB by alumina sol-containing conversion film†
Received
2nd August 2016
, Accepted 11th September 2016
First published on 14th September 2016
Abstract
Alumina film on NdFeB magnets is effective for improving the corrosion resistance and protecting the magnetic properties of the magnets, but the complicated preparation method limits its application. In this study, we prepare alumina-containing conversion film on NdFeB magnets using the reaction of NdFeB magnets with modified alumina sol at the interface. With the successful deposition of the alumina sol-containing conversion film (AS film), the corrosion current density value reduces by more than an order of magnitude and the intergranular corrosion is obviously suppressed in corrosive environment. The thermal stability and magnetic properties of NdFeB magnets are also improved by the AS film. The morphology, composition and structural optimization of the film are systematically investigated. Microstructural investigation suggests that the AS film is uniform and helps to repair defects on the NdFeB surface. Structure analyses reveal that the film is amorphous and protects the magnetic performance. In addition, the underlying mechanism for the formation of the AS film has been analyzed by zeta potential measurement and the potential of zero charge (Epzc) measurement at the sol–metal interface. These results have important implications for the preparation of no-magnetic-loss protection films on sintered NdFeB magnets.
1. Introduction
As the third generation of permanent magnet materials, NdFeB permanent magnets exhibit outstanding magnetic properties and are widely used in various fields, such as electronics, acoustics, communications, automation, magnetic resonance imaging, and biomedical applications.1 Many efforts have been made towards improving both their magnetic properties and thermal stability to meet the demands for different working conditions. It was found that partial substitution of Nd in sintered NdFeB magnets by rare earth (Tb, Dy, Pr) results in an enhancement of coercive field.2–4 However, substitution of rare earth leads to a drastic reduction of the saturation magnetization and the high cost limits the application. Our attention is focused on improvement of magnet performance using non-rare earth compounds. It has also been reported that non-rare earth compounds, such as Al, Mg, Cu and Co, are effective in increasing magnetic properties and thermal stability for NdFeB magnets.5–10 So it is reasonable to further explore methods to improve the NdFeB performance using Al element.
Insufficient corrosion resistance is another limitation for the applications of NdFeB magnets.11,12 The NdFeB magnets are composed of multiphase microstructure, containing matrix Nd2Fe14B phase and intergranular Nd-rich phase. The electrochemical potential of the Nd-rich phase is much more negative than that of the Nd2Fe14B phase, which makes it more vulnerable to decay. As the corrosion resistance of the Nd-rich phase is low, NdFeB magnets are prone to intergranular corrosion, resulting in pulverization failure and significant magnetic deterioration.12–14 In order to improve the corrosion resistance, numerous attempts have been employed, such as the addition of alloying elements and application of surface coatings. The addition of alloying elements, such as Cu, Zn, Al and Sn, can improve the corrosion resistance of NdFeB magnets, but usually at the expense of their magnetic properties.15–19 Electroplating or phosphate conversion coatings are widely employed, but often accompanied by environmental problems and magnetism loss in production.20–22 MgO or AlO film, prepared by magnetron sputtering, improved the magnetic properties and corrosion resistance by optimizing the grain boundaries of the magnets, but the high cost limited their application.23–25 Therefore, it is significant to develop environmentally friendly and in situ-formed alumina-containing film for protecting NdFeB magnets without sacrificing the magnetic performance.
In this work, we propose a one-step method to prepare alumina-sol containing conversion film (AS film) on NdFeB magnets. The chemical activity of NdFeB is high in modified alumina sol, and the dissolved ions from NdFeB promote the alumina sol to gel.26,27 The firstly dissolved Nd-rich phase can be protected by the gelled alumina sol, which helps to prepare uniform alumina-containing protection film on the NdFeB surface. The structure and composition of the AS film are investigated. The results show that the AS film is composed of some complexes containing Al, O and elements from the NdFeB magnet in amorphous state. Performance results demonstrate that the corrosion current density values reduce by more than an order of magnitude and the thermal stability of NdFeB magnets is also improved. We have also explored the underlying mechanism for the formation of alumina-containing film on sintered NdFeB from the modified alumina sol system.
2. Experimental
2.1 Sample preparations
Powder-sintered NdFeB magnets of grade 42H (Nd20.2Tb0.6Fe74.4B4.8) were used in the investigation. Samples (size Ф 18 mm × 4 mm) were polished with SiC abrasive papers (of grades in the range 600–2000), ultrasonically cleaned in alcohol and dried in air. Aluminium isopropoxide (AIP, 99.9%), ethyl alcohol (99.7%), cetyltrimethylammonium bromide (CTAB), acid catalyst and deionized water were used as received without any further purification.
Alumina sol was prepared from the acid-catalyzed reaction of AIP. In the synthesis procedure, AIP, CTAB and ethanol were mixed. Then the mixture was added to deionized water by stirring and the pH value was adjusted by the addition of acid catalyst solution. As shown in Fig. 1, when the AIP was hydrolyzed under acid-catalyzed conditions, part of the Al–OC3H7 transformed into Al–OH. Moreover, under acidic conditions, the activity of Al–OH groups was sufficiently high to undergo condensation under stirring and the alumina chains formed alumina nanoparticles, covered with –OH groups. The hydroxyl-wrapped alumina nanoparticles could adsorb hydrion and CTAB, then became positively charged.
 |
| Fig. 1 Growth model for alumina particles. | |
The AS films were formed by dipping NdFeB magnet samples in the treatment sol at 30 °C. Then the samples were washed with deionized water and dried thoroughly at 60 °C.
2.2 Characterization
A CHI660e electrochemical workstation (Chenhua, China) was used to perform electrochemical dynamic polarization. The tests were performed using a standard three-electrode system with a platinum foil and a saturated calomel electrode (SCE) as the counter and reference electrodes, respectively. The polarization measurements were carried out in the potential range from −0.95 V to −0.45 V (vs. SCE) with a scanning rate of 1 mV s−1. The linear Tafel segments of the cathodic curves were extrapolated to the corrosion potential (Ecorr) to obtain the corrosion current density (icorr). The polarization measurements were conducted in 3.5 wt% NaCl solution at 30 °C and carried out at least three times to ensure reproducible results. The neutral salt spray (NSS) test was carried out in a salt-fog cabinet (Beijing Yashilin Experimental Equipments Ltd, China) with a spraying NaCl solution (5 wt%) at 35 °C.
The magnetic properties of sintered magnets were measured by a hysteresis loop tracer (NIM-2000). The surface morphology and composition of NdFeB magnets with and without AS film were characterized by scanning electron microscopy (FESEM, Apollo 300) and energy dispersive spectroscopy (EDS). X-ray diffraction (XRD) analysis was performed using a Rigaku D/MAX-RB with Cu K radiation (K = 0.15406 nm), which was used to analyze the phase changes. XPS spectra of the sample surfaces were recorded using an ESCALAB 250 Xi system with an Al K anode. The XPS analysis was conducted after the surfaces were etched by an argon-ion beam. All energy values were corrected according to the C 1s spectral line at 284.6 eV. The zeta potential of the alumina particles was calculated using an electrophoretic light scattering spectrometer (Zeta Sizer, Model 3000HS, Malvern Instruments Ltd). Impedance–potential measurements were used to obtain the potential of zero charge (Epzc) through a plot of values of Rp versus applied electrode potential (E). The measurements were carried out in the potential range from −0.9 V to −0.6 V (vs. SCE) with an interval of 10 mV. The test frequency was 1 Hz with an amplitude of 5 mV. The impedance–potential measurements were conducted in silica sol at 25 °C and carried out at least three times to ensure reproducible results. The surface roughness of the NdFeB was observed with a Hirox KH7700 Digital microscope.
3. Results and discussion
3.1 Microstructure and composition of the coating
SEM and EDS were employed to characterize the morphology and composition of bare NdFeB and NdFeB with AS film. Fig. 2 shows representative SEM images of bare NdFeB and NdFeB with AS film obtained by dipping for 3 minutes. Fig. 2(A) shows that there existed some surface defects on bare NdFeB. These were caused by the brittle feature of the NdFeB magnet. As shown in Fig. 2(B), the breakage and desquamation on the bare NdFeB was padded by alumina particles. There also existed agglomerated alumina particles, which were formed by gelled alumina particles, on the AS film (white in contrast). The inset in Fig. 2(B) shows that the AS film was composed of gelled alumina particles on the surface. Fig. 2(C) and (D) show the results of area-scan EDS analysis. The signals of Al and O peaks in Fig. 2(D) suggested the existence of alumina on the surface of NdFeB magnets. The mass percent of O element in the AS film was much higher than that in alumina. At the same time, some ions from NdFeB could get combined with the charged alumina sol particles and the composition of the gelled AS film was more complex than the alumina state. As the AS film is thin, the strong signals of Fe and Nd peaks were also seen in Fig. 2(D).
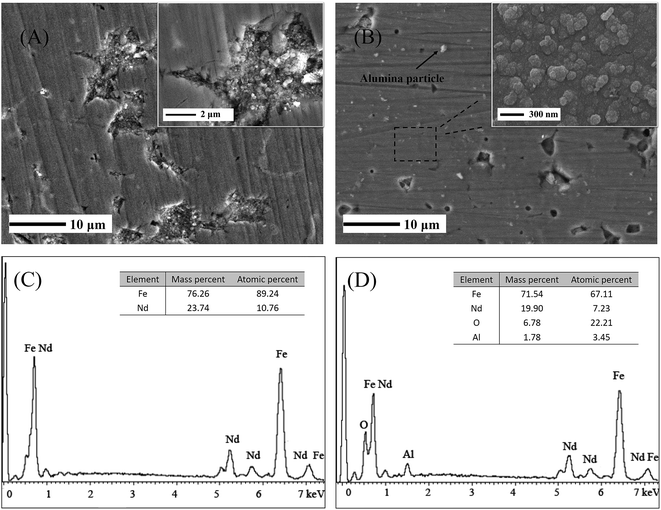 |
| Fig. 2 SEM images of (A) bare NdFeB magnet and (B) NdFeB with AS film. EDS spectra of (C) bare NdFeB magnet and (D) NdFeB with AS film. | |
Fig. 3 shows the cross section of NdFeB with AS film. To distinguish the substrate from the AS film, the specimen was ultrasonically cleaned in alcohol. The spectra of linear EDS analyses, which were scanned following the yellow arrow in the SEM image, shows the distribution of different elements. According to the EDS analyses, the dark region on the surface of NdFeB magnet is the AS conversion film formed from alumina sol. The AS film was compact and well combined with the substrate. And the alumina sol did not dissolve the Nd-rich phase too much, which is helpful for the protection of magnetic properties. The compact film on NdFeB could block the dissolution of substrate metal ions and, at the same time, suppress the formation of AS film. In addition, alumina particles, contained in the alumina-containing conversion film, could perform as corrosion inhibitors to protect the substrate from corrosion.
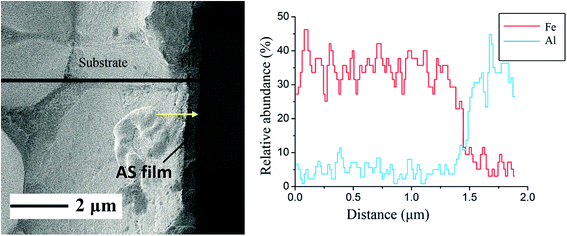 |
| Fig. 3 Cross-sectional SEM image of the NdFeB with AS film, and linear EDS analyses carried out following the yellow arrow. | |
3.2 XRD and TEM analyses
To evaluate the effects of the alumina sol on the NdFeB, the XRD patterns of bare NdFeB and NdFeB with AS film were recorded; the results are shown in Fig. 4. As seen in Fig. 4, the diffraction peaks of (0 0 4), (1 0 5), (0 0 6) and (0 0 8) were characteristic peaks of NdFeB magnets (PDF 36-1296, PDF 07-0090). In comparison with bare NdFeB, there was no newly emerging diffraction peak in the pattern of NdFeB with AS film, which helps to protect the magnetic properties. The relative intensity of the NdFeB diffraction peaks decreased when it was covered by AS film. As there is no new diffraction peak appearing, the AS film formed on the NdFeB magnets was composed of an amorphous structure. Transmission electron microscopy (TEM) was used to further study the structure of the AS film. The TEM images and diffraction patterns of bare NdFeB and NdFeB with AS film prepared from alumina sol are shown in Fig. 5. The tetragonal structure of NdFeB magnets is clearly shown in Fig. 5(A) and (B). While the disordered structure and blurred ring pattern indicated the AS film was amorphous. AS films were composed of some complexes containing Al and O and metal ions from the NdFeB magnet. This mixture is prone to form amorphous structure on the surface of NdFeB magnets.
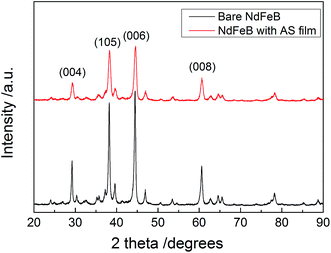 |
| Fig. 4 XRD patterns of bare NdFeB and NdFeB with AS film. | |
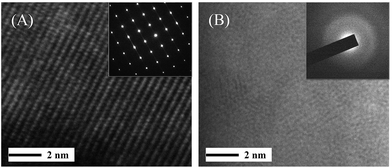 |
| Fig. 5 TEM images and diffraction patterns of (A) bare NdFeB and (B) NdFeB with AS film. | |
3.3 XPS analysis
In order to determine the chemical state changes of the elements in bare NdFeB and AS film, XPS analysis was used, and the high-resolution spectra of Al 2p, Nd 3d, Fe 2p and B 1s are presented in Fig. 6. Compared with bare NdFeB, there was a peak of Al 2p in the AS film (Fig. 5(A)). The binding energy of the Al 2p peak (74.5 eV) was assigned to Al3+, implying the existence of alumina in the AS film. For the bare NdFeB, the binding energies of Nd 3d (981.6 eV), Fe 2p (706.7 eV) and B 1s (187.7 eV) were in good agreement with the metal state of Nd and Fe. And in the AS film, the binding energies of Nd 3d (984.7 eV), Fe 2p (708.1 eV) and B 1s (191.2) were in good agreement with the oxidation state of Nd3+ and Fe2+. The results showed that the Nd and Fe elements changed from metal state to oxidation state in the formation process of the AS film.4,15,28 The results of XPS analysis demonstrate that the formation of AS film involves the oxidation process of elements in the NdFeB magnet and the deposition of alumina from the alumina sol.
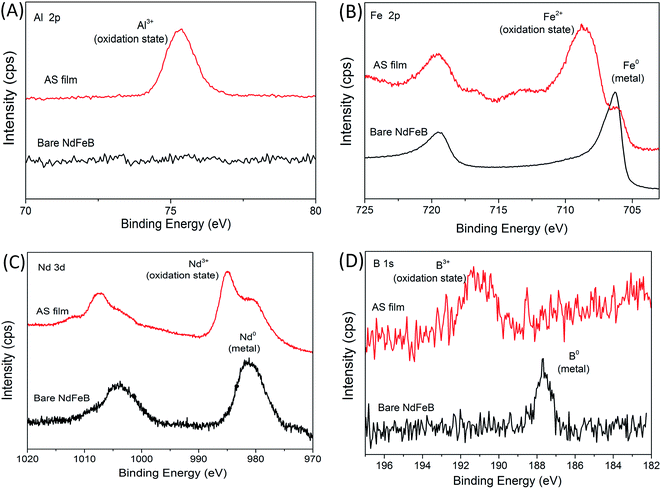 |
| Fig. 6 High-resolution XPS spectra of (A) Al, (B) Fe, (C) Nd and (D) B for bare NdFeB and NdFeB with AS film prepared from alumina sol. | |
3.4 Potential of zero charge (Epzc) and formation of AS film
The surface charge state of NdFeB magnets in alumina sol is important in studying the formation process of AS film. It can be determined by a comparison of open circuit potential (Eocp) with the potential of zero charge (Epzc). A plot of Rp vs. the applied potential is shown in Fig. 7. The net surface charge of the NdFeB at the Eocp can be utilized with respect to the equation:where Er is Antropov's “rational” potential of corrosion.29–33
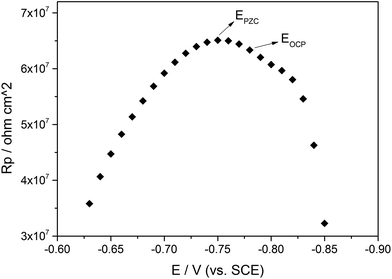 |
| Fig. 7 Plot of Rp vs. applied electrode potential for NdFeB magnets in alumina sol. | |
As shown in Fig. 7, the obtained curve appears parabolic in shape, with a maximum point shown at −0.75 V (vs. SCE). This point can be called the Epzc of the NdFeB in the alumina sol. The Eocp of NdFeB in the same conditions was −0.778 V (vs. SCE), which was more negative than the Epzc, indicating a negatively charged NdFeB surface (Er = Eocp − Epzc = −0.028 V). When the NdFeB magnet was immersed in the alumina sol, the elements of Nd, Fe and B dissolved in the solution as multivalent cations, such as Nd3+, Fe2+ and B3+ (eqn (2)–(4)). It also resulted in a negative charge on the NdFeB surface. The negative charge suppressed the further generation of Nd3+, Fe2+ and B3+.
The alumina sol was designed to promote the uniform formation of AS film on the surface of NdFeB magnets. The charge state of the alumina particles in the alumina sol is important for the formation of AS film on the NdFeB magnets. The zeta-potential of the alumina sol was calculated to determine the surface charge of alumina particles using an electrophoretic light scattering spectrometer. The zeta-potential of alumina particles was (31.8 ± 1.5) mV, implying the particle surface was positively charged by hydrogen ion:
|
x(AlOOH) + yH+ → x(AlOOH)·yH+
| (5) |
As shown in Fig. 8, when the NdFeB was dipped in the alumina sol, the positively charged alumina particles and the cations can easy reach the negatively loaded NdFeB surface because of electrostatic attraction. The acidic condition promoted the dissolution of NdFeB substrate and the consumption of hydrion near the substrate surface decreased the surface charge of alumina particles, which make it easier for them to gel on NdFeB. The third model in Fig. 8 shows that the alumina particles tended to chelate with the cations, which could precipitate and form the AS film. And the hydrion, adsorbed on the alumina particles, gained the electrons released from the negatively charged NdFeB surface and formed hydrogen in the process (eqn (6)). This reaction consumed the charged hydrogen ions on the surface of alumina particles and catalyzed the condensation of alumina sol so as to gel on the NdFeB surface. As the dissolution of NdFeB catalyzed the gelling of alumina particles, the Nd-rich phase, which was easier to dissolve, was firstly protected by the formed AS film. And the formation of the AS film continued after the Nd2Fe14B phase was covered by AS film. While the alumina particles chelated with these ions and gelled on the NdFeB to form AS film (eqn (7)–(9)). The reactions reduced the negative charge on the NdFeB surface, which would be conducive to the generation of Nd3+, Fe2+ and B3+. These reactions were finally suppressed when a compact AS film was produced on the NdFeB surface. Table 1 shows the increased mass of NdFeB with an increase of dipping time. The mass of the samples increased in the first 6 minutes because of the AS film formation on the substrate. When the dipping time was longer than 9 minutes, the increase of mass almost stopped, indicating that the formation of AS film was suppressed. The formed AS film would block the dissolution of metal ions and the thickness of AS film might not increase further.
|
 | (6) |
|
 | (7) |
|
 | (8) |
|
 | (9) |
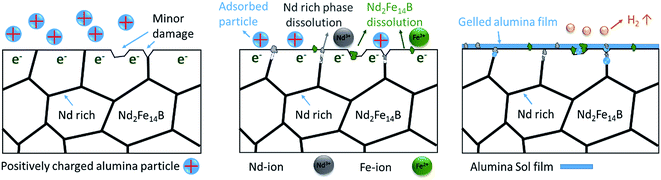 |
| Fig. 8 Growth model for alumina sol-containing conversion film on NdFeB magnet. | |
Table 1 Increased mass of the NdFeB magnets with dipping time
Dipping time (minutes) |
1 |
3 |
6 |
9 |
12 |
15 |
Increased mass (g m−2) |
0.4 |
1 |
1.6 |
1.8 |
1.9 |
1.9 |
3.5 Magnetic properties and temperature stability
Table 2 shows the magnetic properties at 25 °C for the NdFeB magnets before and after the formation of AS films. The remanence increased slightly from 0.927 to 0.933 T at 25 °C and the coercivity decreased from 1561 to 1553 kA m−1. The (BH)max of the bare magnet and the magnet with AS film were calculated to be 176.5 and 179 kJ m−3, respectively. The results demonstrated that the formation of AS films did not harm the magnetic properties of the NdFeB magnet, while the Jr and (BH)max increased in value. In order to measure the irreversible magnetic losses (Lirr) of the NdFeB magnet, the bare NdFeB and the NdFeB with AS film were heat treated for 4 hours at temperatures up to 120 °C and 160 °C. The irreversible magnetic losses due to the heat exposure, which causes the oxidation of Nd-rich phase, were calculated from: |
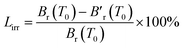 | (10) |
where Br(T0) and B′r(T0) are the remanence at 25 °C before and after the heat exposure.4,34,35 Based on the above definitions, both coefficients are negative. The lower the absolute values of the coefficients are, the higher is the thermal stability. As shown in Table 2, the irreversible magnetic loss of bare NdFeB exposed at 120 °C for 4 h was 2.15%, and was 2.47% after 4 h at 160 °C. However, the irreversible magnetic losses of NdFeB with AS films exposed at 120 °C and 160 °C for 4 h were only 1.62% and 1.81%, indicating that AS films decreased the irreversible magnetic losses of NdFeB magnets. According to the chemical reactivity features of the NdFeB magnet, the dissolved metal ions from NdFeB catalyzed the gelling of alumina particles and formed the AS film. The results of mass increasing with dipping time demonstrated that the formation of AS film was finally suppressed when a compact AS film was produced on the NdFeB surface. As the formation of AS film only consumed a small amount of NdFeB and most of the matrix Nd2Fe14B phase maintained stable, the magnetic properties of NdFeB magnets were not harmed with the formation of the AS film. At the same time, with the combination of dissolved intergranular Nd-rich phase with alumina particles, the grain boundary, close to the magnet surface, was filled by the gelled AS film compounds. The particle-like intergranular Nd–O–Fe–Al phase was reported to result in improved coercivity and thermal stability.24,25,36,37 And the AS film protected the NdFeB from oxidation on heat exposure, and the cores for the magnetization reversal process were not easily formed. As a result, the AS film helped to improve the thermal resistance of the sintered NdFeB magnet without sacrificing magnetic properties and made it possible to work at a higher temperature for a longer time.
Table 2 Magnetic properties at 25 °C, and irreversible magnetic losses (Lirr) at 120 °C and 160 °C
Sample |
Jr (T) |
Hcj (kA m−1) |
(BH)max (kJ cm−3) |
Lirr at 120 °C (%) |
Lirr at 160 °C (%) |
Bare NdFeB |
0.927 |
1561 |
176.5 |
2.15 |
2.47 |
NdFeB with AS film |
0.933 |
1553 |
179 |
1.62 |
1.81 |
3.6 Corrosion resistance
A standard solution with a content of 3.5 wt% NaCl was chosen as the corrosion medium for determination and comparison of corrosion behavior in the present work. The bare NdFeB and NdFeB with AS film obtained by dipping for 3 minutes were immersed in 3.5 wt% NaCl solution for 24 hours to evaluate the corrosion-resistant ability. As shown in Fig. 9(A), the bare NdFeB was seriously eroded. The surface of NdFeB was full of corrosion pits with lots of adsorbed corrosion products. Fig. 9(B) shows that NdFeB with AS film was well protected. There was no red corrosion product adsorbed on the substrate after the immersion. Fig. 9(C) shows that the bare NdFeB was covered by adsorbed corrosion products. At the same time, there were some cracks on the substrate, while some of the NdFeB particles chipped along grain boundaries. As shown in Fig. 9(D), the NdFeB particles did not chip off, while only some part of the Nd-rich phase eroded along the NdFeB particles. The surface of NdFeB did not crack with the protection of the AS film. And the corrosion products decreased obviously in comparison with the bare NdFeB.
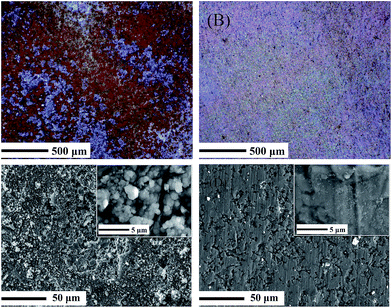 |
| Fig. 9 Optical and SEM images of (A and C) bare NdFeB magnets and (B and D) NdFeB with AS film immersed in 3.5 wt% NaCl solution for 24 hours. | |
The polarization curves of bare NdFeB and NdFeB with AS films (prepared with different dipping times) are shown in Fig. 10. Under high-overpotential conditions, the electrochemical reaction rate under kinetic control could be represented simply by the Tafel relationship. Therefore, by fitting the data via linear extrapolation at high overpotential (over 100 mV), we were able to obtain the corrosion current density (jcorr) from the intersection with the ordinate and the corrosion potential (Ecorr) from the intersection with the abscissa. The corresponding parameters are listed in Table 3. Compared with the bare NdFeB, the Ecorr of NdFeB with AS films dipped for 3 minutes, 6 minutes and 9 minutes shifted to more positive potentials: approximately 34 mV, 17 mV and 8 mV, respectively. Given that Ecorr is a measure of corrosion susceptibility, the shift in Ecorr to more positive values suggests an increase in corrosion resistance due to the presence of AS films. They changed the surface state of NdFeB and provide good corrosion resistance. Compared with bare NdFeB, the corrosion current densities of NdFeB with AS films were much lower, indicating that the AS films were effective in protecting the NdFeB magnets from corrosion. The jcorr of NdFeB with AS film dipped for 6 minutes was more than one order of magnitude lower than that of the bare NdFeB. The current densities increased slightly when the dipping time increased to 9 minutes. This may be caused by cracks on the AS film or the adsorbed Cl element with longer dipping time.
 |
| Fig. 10 Polarization curves of bare NdFeB and NdFeB with AS films (dipped for different times) in 3.5 wt% NaCl at 25 °C. | |
Table 3 The electrochemical parameters of bare NdFeB and NdFeB with alumina sol coatings in 3.5 wt% NaCl at 25 °C
Alumina sol dipping time (min) |
Ecorr (mV, vs. SCE) |
jcorr (A cm−2) |
0 |
−841 |
9.4 × 10−6 |
1 |
−807 |
4.5 × 10−6 |
2 |
−824 |
7.9 × 10−7 |
3 |
−833 |
1.9 × 10−6 |
The results showed that the dipping time in alumina sol had a significant impact on the corrosion resistance of AS films. The impact of dipping time on NdFeB substrate was further studied using SEM and EDS. Fig. 11(A) shows that the morphology of NdFeB with AS film obtained by dipping for 6 minutes was coarser. And there were some agglomerated particles on the film. As shown in Fig. 11(B), when the NdFeB was dipped for 9 minutes, cracks appeared on the film. As shown in Fig. 11(C) and (D), in comparison with the EDS spectra in Fig. 2, the peaks of Al and O elements were relatively stronger when the films were dipped for a longer time. This indicted more alumina sol was involved in the formation of AS film with an increase of dipping time, which helped to increase the corrosion resistance. The AS films dipped for 9 minutes contained extra peaks of Cl element. The adsorption of Cl element may lead to a decrease of the corrosion resistance. These results were consistent with those of polarization measurements.
 |
| Fig. 11 SEM images and EDS spectra of NdFeB with AS film obtained by dipping for (A and C) 6 minutes and (B and D) 9 minutes. | |
In order to further study the protective properties of the AS film obtained by dipping for 6 minutes for NdFeB, the NSS test was carried out. Fig. 12 shows the morphology of bare NdFeB (Fig. 12(A) and (C)) exposed to NSS environment for 1 h and 3 h, and NdFeB with AS film obtained by dipping for 6 minutes (Fig. 12(B) and (D)) exposed for 1 h and 12 h. When the exposure time was 1 hour, there were some corrosion pits adsorbed by corrosion products in Fig. 12(A), while few corrosion pits appeared in Fig. 12(B). As the exposure time reached 3 h, the bare NdFeB (Fig. 12(C)) was fully covered by red corrosion products. When the sample was rinsed with deionized water, many corroded NdFeB particles fell off because of the serious intergranular corrosion. Fig. 12(D) shows that pitting corrosion occurred on NdFeB with AS film after 12 h exposure to NSS environment. The 3D model images of corrosion pits on bare NdFeB (Fig. 12(E), 5 h exposure) and NdFeB with AS film (Fig. 12(F), 12 h exposure) were observed with a Hirox KH7700 Digital microscope. As shown in Fig. 12(E), the surface roughness of bare NdFeB reached 20 μm because of detachment of the NdFeB particles and the formation of red corrosion products. Fig. 12(F) shows that even when the exposure time reached 12 h, there was no grain spalling on the NdFeB with AS film. The quantity of corrosion products was much less than in Fig. 12(E), and the depth of the corrosion pits was less than 4 μm. The result indicated that the AS film effectively protected the NdFeB from the corrosive medium.
 |
| Fig. 12 Neutral salt spray results of (A) bare NdFeB, 1 h, (B) NdFeB with AS film, 1 h, (C and E) bare NdFeB, 3 h and (D and F) NdFeB with AS film, 12 h. | |
4. Conclusion
In this work, we proposed a one-step method to prepare AS conversion films on NdFeB magnets from a modified alumina sol. In the alumina sol, the negatively charged NdFeB surface adsorbed the alumina particles via electrostatic interaction. Thus the positively charged alumina particles chelated with cations and gelled on the NdFeB surface, which could precipitate and form the AS film. The film contained elements of Al, O, Nd, Fe and B, with the elements of Nd, Fe and B existing in the film as oxidation state. The corrosion current value decreased by more than one order of magnitude, suggesting that the AS film effectively improved the corrosion resistance of NdFeB magnets. As determined from EDS, XRD and TEM analyses, the film was composed of Al, O and metal elements in an amorphous state. And the formed AS film improved the thermal stability of NdFeB magnets and did not damage the magnetic properties. It is believed that the presented alumina-containing conversion film prepared from alumina sol has great significance in further improving the properties of NdFeB magnets.
Acknowledgements
Authors acknowledge financial support of the National Natural Science Foundation of China (51371020).
References
- M. Sagawa, S. Fujimura, N. Togawa, H. Yamamoto and Y. Matsuura, J. Appl. Phys., 1984, 55, 2083 CrossRef CAS.
- S. Sugimoto, J. Phys. D: Appl. Phys., 2011, 44, 064001 CrossRef.
- K. Hono and H. Sepehri-Amin, Scr. Mater., 2012, 67, 530–535 CrossRef CAS.
- X. G. Cui, C. Y. Cui, X. N. Cheng and X. J. Xu, Intermetallics, 2014, 55, 118–122 CrossRef CAS.
- T. Xie, S. Mao, C. Yu, S. Wang and Z. Song, Vacuum, 2012, 86, 1583–1588 CrossRef CAS.
- S. Pandian, V. Chandrasekaran, G. Markandeyulu, K. J. L. Iyer and K. V. S. Rama Rao, J. Appl. Phys., 2002, 92, 6082 CrossRef CAS.
- W. Mo, L. Zhang, A. Shan, L. Cao, J. Wu and M. Komuro, J. Alloys Compd., 2008, 461, 351–354 CrossRef CAS.
- O. Gutfleisch, M. A. Willard, E. Bruck, C. H. Chen, S. G. Sankar and J. P. Liu, Adv. Mater., 2011, 23, 821–842 CrossRef CAS PubMed.
- C. Sun, W. Q. Liu, H. Sun, M. Yue, X. F. Yi and J. W. Chen, J. Mater. Sci. Technol., 2012, 28, 927–930 CAS.
- W. Liu, C. Sun, M. Yue, H. Sun, D. Zhang, J. Zhang, X. Yi and J. Chen, J. Rare Earths, 2013, 31, 65–68 CrossRef CAS.
- I. Gurrappa and S. Pandian, Corros. Eng., Sci. Technol., 2006, 41, 57–61 CrossRef CAS.
- E. Isotahdon, E. Huttunen-Saarivirta, V. T. Kuokkala and M. Paju, Mater. Chem. Phys., 2012, 135, 762–771 CrossRef CAS.
- X. Zhang, Y. Ma, B. Zhang, Y. Li, M. Lei, F. Wang, M. Zhu and X. Wang, Corros. Sci., 2014, 87, 156–166 CrossRef CAS.
- E. Isotahdon, E. Huttunen-Saarivirta, S. Heinonen, V. T. Kuokkala and M. Paju, J. Alloys Compd., 2015, 626, 349–359 CrossRef CAS.
- J. Li, S. Mao, K. Sun, X. Li and Z. Song, J. Magn. Magn. Mater., 2009, 321, 3799–3803 CrossRef CAS.
- J. Ni, S. Zhou, Z. Jia and C. Wang, J. Alloys Compd., 2014, 588, 558–561 CrossRef CAS.
- G. Sadullahoğlu, B. Altuncevahir and O. Addemir, Acta Phys. Pol., A, 2014, 125, 1172–1175 CrossRef.
- S. Mao, H. Yang, J. Li, H. Ying and Z. Song, Vacuum, 2011, 85, 772–775 CrossRef CAS.
- J. Ma, X. Liu, W. Qu and C. Zhou, J. Therm. Spray Technol., 2014, 24, 394–400 CrossRef.
- H. Nan, L. Zhu, H. Liu and W. Li, Appl. Surf. Sci., 2015, 355, 1215–1221 CrossRef CAS.
- Z. Cheng, J. Lian, X. Hu, X. Yang and G. Li, Chem. Res. Chin. Univ., 2014, 30, 543–548 CrossRef CAS.
- C. B. Ma, F. H. Cao, Z. Zhang and J. Q. Zhang, Appl. Surf. Sci., 2006, 253, 2251–2256 CrossRef CAS.
- C. Ma, X. Liu and C. Zhou, J. Therm. Spray Technol., 2013, 23, 456–462 CrossRef.
- Q. Zhou, Z. W. Liu, X. C. Zhong and G. Q. Zhang, Mater. Des., 2015, 86, 114–120 CrossRef CAS.
- J. J. Ni, T. Y. Ma, Y. R. Wu and M. Yan, J. Magn. Magn. Mater., 2010, 322, 3710–3713 CrossRef CAS.
- M. Nofz, J. Pauli, M. Dressler, C. Jügeg and W. Altenburg, J. Sol-Gel Sci. Technol., 2006, 38, 25–35 CrossRef CAS.
- I. Kheirollahi, M. Abdellahi, M. Emamalizadeh and H. Sharifi, Ceram. Int., 2015, 41, 15083–15088 CrossRef CAS.
- V. Bilovol, S. Ferrari, D. Derewnicka and F. D. Saccone, Mater. Chem. Phys., 2014, 146, 269–276 CrossRef CAS.
- A. Ongun Yüce, B. Doğru Mert, G. Kardaş and B. Yazıcı, Corros. Sci., 2014, 83, 310–316 CrossRef.
- R. Yıldız, T. Doğan and İ. Dehri, Corros. Sci., 2014, 85, 215–221 CrossRef.
- R. Yıldız, A. Döner, T. Doğan and İ. Dehri, Corros. Sci., 2014, 82, 125–132 CrossRef.
- R. Solmaz, Corros. Sci., 2014, 81, 75–84 CrossRef CAS.
- A. Popova, E. Sokolova, S. Raicheva and M. Christov, Corros. Sci., 2003, 45, 33–58 CrossRef.
- R. Zhang, Y. Liu, J. Li, S. Gao and M. Tu, Mater. Charact., 2008, 59, 642–646 CrossRef CAS.
- Z. Hu, H. Wang, D. Ma and C. Luo, J. Low Temp. Phys., 2012, 170, 313–321 CrossRef.
- Z. W. Liu and H. A. Davies, J. Phys. D: Appl. Phys., 2007, 40, 315–319 CrossRef CAS.
- Z. Chen, A. Yan, X. Wang and G. C. Hadjipanayis, J. Appl. Phys., 1997, 81, 4456 CrossRef CAS.
Footnote |
† Electronic supplementary information (ESI) available. See DOI: 10.1039/c6ra19531h |
|
This journal is © The Royal Society of Chemistry 2016 |
Click here to see how this site uses Cookies. View our privacy policy here.