DOI:
10.1039/C6RA18268B
(Paper)
RSC Adv., 2016,
6, 110661-110673
Antioxidant properties of Artemisia herba-alba and Eucalyptus camaldulensis essentials oils on malathion-induced reproductive damage in rat†
Received
18th July 2016
, Accepted 25th October 2016
First published on 7th November 2016
Abstract
Malathion (M) is an organophosphorus pesticide of utmost concern because of its adverse effects on non-targeted organisms. It is well-known for its anti-acetylcholinesterase activity and its negative effects on the liver, pancreas and the immune, hematological and reproductive systems. Natural antioxidants including phenolics, essential oils and vitamins, among others, might have protective effects on the toxicity of malathion. The intent of this study was to examine whether the treatment with Artemisia herba-alba (AhaEO) and Eucalyptus camaldulensis (EcEO) essential oils may be useful to overcome the deleterious effects of malathion administration on reproductive function in male rats. Depending on what group they were in, the following dosages were administered to rats via oral gavage for 21 days: only malathion at a dose of 200 mg kg−1 b.w.; malathion (200 mg kg−1 b.w.) following treatment with either AhaEO or EcEO (50 mg kg−1 b.w.); only AhaEO or EcEO. The results indicate that malathion affects the male reproductive system by the reduction of epididymal sperm count, motility and viability, and by increasing abnormal sperm morphology. These effects were tightly associated with oxidative stress, as revealed by the significant increase in lipid peroxidation (MDA) and hydrogen peroxide (H2O2), and the decrease in thiol groups (SH) and antioxidant enzymes such as superoxide dismutase (SOD), catalase (CAT) and glutathione peroxidase (GPx) in the testis and epididymis of M-treated rats. Essentials oils of AhaEO and EcEO prevented testicular and epididymis oxidative damage, as evidenced by reduced MDA and H2O2 levels, and improved total sperm motility, viability and morphology in rat spermatozoa. Our study shows that AhaEO and EcEO can offer protection against malathion-induced reproductive toxicity via their potent antioxidant activity, and may become a potential treatment for male infertility associated with malathion exposure.
Introduction
The increasing release of chemicals into the environment has drawn attention towards gaining a better understanding of their toxicity to humans and ecotoxicological effects. Many studies suggest that environmental contaminants disrupt male reproduction and play an important role in the reduction of the quality and quantity of human semen.1 Several pesticides employed today are known to adversely impair the reproductive competence of males in laboratory, field, clinical and occupational settings. Published studies report that organophosphorus can impair fertility, deteriorate semen quality, and cause testicular degeneration, male reproductive failure and malformations in rodent fetuses following repeated exposure.2 Malathion has harmful effects on various organs and systems including the kidneys,3 liver,3 lungs,4 brain5 and erythrocytes.6 This insecticide is metabolized in the liver by multiple specific cytochrome P450 enzymes through several reaction pathways.7 Indeed, malathion is known to inhibit acetylcholinesterase activity in target tissues.8,9 The harmful effects of malathion are, in part, ascribed to its ability to induce oxidative stress, characterized by a depletion of endogenous antioxidant enzyme activity such as SOD, CAT and GPx, with a concomitant accumulation of reactive oxygen species (ROS) in tissues. The uncontrolled generation of ROS can cause damage to a wide range of essential biomolecules, such as DNA,10 proteins11 and lipids,12 and they have been associated with numerous diseases, including atherosclerosis, carcinogenesis, coronary heart disease, pathogenesis of ageing and many degenerative diseases. Therefore, it is essential to search for and utilize effective antioxidants to scavenge free radicals in the human body. Essential oils are bioactive secondary metabolites and some of them have a wide array of biological activities including antioxidant, antimicrobial, anti-inflammatory, anti-tumor, and analgesic activities, among others.13 Their high efficacy, low mammalian toxicity, low environmental toxicity and wide public acceptance, make them potential therapeutic and preventive agents against a plethora of diseases and toxicants.
Artemisia herba-alba (Asteraceae) is a greenish-silver perennial dwarf shrub growing in arid and semi-arid regions. It occurs through the Mediterranean region in North Africa, Spain, deserts of Sinai Peninsula, Middle East, Northwestern Himalayas, and in India.14,15 This plant is credited with a long list of medicinal uses and is commonly used for the treatment of colds, coughing, bronchitis, intestinal disorders and diabetes.16,17 The leishmanicidal, antibacterial, and antifungal properties of A. herba-alba extracts have also been reported.18,19
Species from the genus Eucalyptus have been used for pharmaceutical and medicinal purposes,20,21 and several studies have reported that E. camaldulensis leaves display multiple pharmacological activities, including antibacterial,22 anti-inflammatory,23 antitermitic,24 larvicidal and mosquito repellent,25,26 and antioxidative activities, among others.27 Most of these intriguing biological activities of A. herba-alba and E. camaldulensis have been attributed to their particular essential oil composition.22 Despite the substantial data on the chemical composition of their essential oils and their biological activities, little is known about their effects on malathion-induced reproductive damage. Consequently, the present work was intended to evaluate the effects of E. camaldulensis (EcEO) and A. herba-alba (AhaEO) essential oils against malathion-induced male reproductive toxicity.
Material and methods
Chemicals
5,5-Dithio-bis(2-nitrobenzoic acid) (DTNB), trichloroacetic acid (TCA), acetylcholine iodide, S-butyrylcholine, butylated hydroxytoluene (BHT), potassium hydroxide (KOH), ethanol, ether, Coomassie G250, bovine serum albumin (BSA), orthophosphoric acid and sodium chloride (NaCl) were obtained from Sigma-Aldrich Inc. (Steinheim, Germany).
Plant collection
The aerial parts of A. herba-alba and E. camaldulensis were collected in June 2013 from the locality of Tabarka (North-western Tunisia) and identified by the laboratory of taxonomy in the Faculty of Sciences of Tunis (Tunis, Tunisia). The voucher specimens have been deposited in the herbarium of the Higher Institute of Biotechnology of Béja and also in the Department of Biological Sciences, Faculty of Science of Tunis, Tunisia.
Extraction and analysis of essential oils
The air dried aerial parts (100 g) were subjected to hydrodistillation for 3 h using a Clevenger-type apparatus. The oils obtained were dried over anhydrous sodium sulphate (Na2SO4) and stored in amber and air-tight vials at 0 °C until required.
Analytical gas chromatography (GC-FID) was carried out on a Hewlett-Packard 6890 gas chromatograph series II (Agilent Technologies, Palo Alto, California, USA) equipped with HP-5 (60 m × 0.25 mm, 0.25 μm film thickness) capillary columns.
Diluted oil samples in n-hexane were injected with a split ratio of 1
:
60 and a continuous flow rate of 1.6 mL min−1 of chromatographic grade nitrogen was used. The oven temperature was initially held for 10 min at 35 °C, ramped at 3 °C min−1 up to 205 °C and held at a constant temperature for 10 min. The injector and FID detector temperatures were held at 250 and 300 °C, respectively.
The gas chromatography-mass spectrometry (GC-MS) analyses were performed on a gas chromatograph HP 6890 (II) interfaced with an HP 5973 mass spectrometer (Agilent Technologies, Palo Alto, California, USA) with electron impact ionization (70 eV). An HP-5MS capillary column (60 m × 0.25 mm, 0.25 μm film thickness) was used. The column temperature was programmed to rise from 40 to 280 °C at a rate of 5 °C min−1. The carrier gas was helium with a flow rate of 1.2 mL min−1. The scan time and mass range were 1 second and 50–550 m/z, respectively.
The volatile compounds were identified by the comparison of their retention indices relative to (C7–C28) n-alkanes with those in the literature and/or those of authentic compounds available in our laboratory, and by matching their mass spectral fragmentation patterns with corresponding data (Wiley 275.L library) and other published mass spectra.
Relative percentages of the identified compounds were obtained from the electronic integration of the FID peak areas.
Free radical scavenging activity
For the evaluation of the free radical scavenging activity, the 2,2-diphenyl-1-picrylhydrazyl (DPPH) assay was used.28 Briefly, various concentrations of the essential oils (20, 50, 100, 150, and 200 μg mL−1) were added to 1 mL of a freshly prepared 0.1 mM methanol solution of DPPH. After a 30 min incubation at room temperature, the absorbance was measured against a blank at 517 nm. The DPPH radical scavenging activity (RSA), expressed as a percentage, was calculated using the following formula:
RSA (%) = [(control absorbance − sample absorbance)/control absorbance] × 100 |
The synthetic antioxidant reagent, butylated hydroxytoluene (BHT), was used as the positive control.
All analyses were performed in triplicate. The effective concentration, having 50% radical inhibition (EC50) expressed as μg mL−1, was determined from the graph of the RSA (%) against the essential oil concentration.
Animals and treatment
Adult male Wistar rats (weighing 220–230 g, 15 weeks old and housed five per cage) were purchased from SIPHAT (Tunis, Tunisia) and used in accordance with the local ethic committee of Tunis University for use and care of animals in conformity with the NIH recommendations. The animals were housed in standard cages (40 × 28 × 16 cm) under controlled conditions: 12
:
12 h light–dark, 20–22 °C, food and water were ad libitum. After diabetes induction, the rats were divided into 6 groups of 12 animals each:
Group I: control rats (CTR) treated with corn oil (1 mL, o.p.).
Group II: malathion treated rats (M) (200 mg kg−1 b.w., o.p) in corn oil.
Group III: malathion treated rats (200 mg kg−1 b.w., o.p) treated with AhaEO (50 mg kg−1 b.w., o.p.).
Group IV: malathion treated rats (200 mg kg−1 b.w., o.p) treated with EcEO (50 mg kg−1 b.w., o.p.).
Group V: rats treated with AhaEO (50 mg kg−1 b.w., o.p.).
Group VI: rats treated with EcEC (50 mg kg−1 b.w., o.p.).
All treatments were given orally by gavage once daily for 21 consecutive days. 24 hours after the last administration, the animals were sacrificed and blood was collected in heparinized tubes. After centrifugation at 3000 g for 15 min, the plasma was processed for biochemical parameter determinations. The testis and epididymis were rapidly excised and homogenized in saline phosphate buffer and the sperm was collected in 5cc tubes. After centrifugation at 10
000g for 10 min at 4 °C, the supernatants were used for biochemical determination.
The animals were maintained in accordance with the guidelines of the National Institute of Nutrition, Tunis, Tunisia, and approved by the institutional ethical committee (IEC) of Tunisian faculty of sciences, for the use and care of animals in conformity with the NIH recommendations.
Body and reproductive organ weights
Initial (weight at starting point) and final (weight at end point) body weights were recorded. The reproductive organs were stripped from fatty tissues and blood vessels, blotted, and their absolute weights were determined. The clinical properties of the body and reproductive organs were evaluated for toxicological criteria. To normalize the data for statistical analysis and to obtain the relative weights, data were expressed per 100 g of body weight.
Plasma acetylcholinesterase and butyrylcholinesterase activity (AChE and BChE)
The cholinesterase activity was assayed in plasma by the method of Ellman29 using acetylthiocholine iodide as a substrate. The rate of hydrolysis of acetylthiocholine was measured at 405 nm using the reaction of thiocholine with dithiobisnitrobenzoic acid to give the yellow 5-thio-2-nitrobenzoate anion. The enzyme activity was expressed as nmol of substrate hydrolyzed per min per mg protein.
Evaluation of serum testosterone
The concentration of plasma testosterone was measured using commercial enzyme-linked immuno-sorbent assays (ELISA) purchased from Demeditec (Ref, DEV 9911), radioimmunoassay and ELISA, using commercial kits as per manufacturer's instructions.
Evaluation of sperm characteristics
(i) Sperm collection. After the test period, a laparotomy was conducted for all exposed and control male rats after body weight measurement. Testis and epididymis were carefully excised. The sperm count was assessed from the right cauda epididymis, while sperm motility and morphology were analyzed from the left one. The epididymis was excised and minced in 1 mL of RPMI to obtain a spermatozoa suspension. The remaining testis and epididymis were frozen at −20 °C until use.
(ii) Sperm count. The cauda epididymal sperm count was performed according to the method of Vega et al.30 Epididymal sperm counts were expressed as the number of spermatozoa per epididymis. To minimize error, the count was repeated three times on each sample.31
(iii) Sperm motility. 10 mL of sperm suspension was layered onto a warmed microscope slide. Sperm motility was assessed by counting all progressive motile, non-progressive motile and immotile spermatozoa. The number of motile spermatozoa in each field was divided by the total number, and the average of the fields was assayed. The percentage of motile spermatozoa was thereafter determined.32
(iv) Sperm viability. Sperm viability was assessed using the eosin stain.33 The staining was performed with one drop of freshly collected semen (10 μL) and two drops of a solution (20 μL) of eosin. The living spermatozoa remained intact and therefore did not become stained, while the dead ones were purple with red-staining. The dye exclusion was evaluated in 100 spermatozoa. Sperm viability was defined as the percentage of dead sperm cells. Viability was evaluated according to WHO guidelines.34
(v) Sperm morphology. A drop of sperm suspension was smeared on a slide and air-dried and made permanent. The smeared slide was stained with 1% eosin. Morphological sperm defects were evaluated and examined on an optical microscope.35 At least 100 spermatozoa from different fields in each slide were examined and classified for criteria of morphological abnormalities (head, tail and tail-head) according to Filler.36 Abnormal sperm cells were counted and the percentage was calculated.
(vi) Assessment of sperm production. Sperm content per g was determined using the method previously described by Vega et al. and Narayana et al. with slight modifications.30,37 Briefly, after thawing at a temperature of 25–27 °C, all epididymal and testicular tissues were homogenized for 5 min in 5 mL of physiological saline (0.9% NaCl) containing 0.05% (v/v) Triton X-100, using a manual homogenizer. The homogenates were diluted with 1.5 mL of the saline solution and the spermatozoa and spermatids were counted. Three counts per sample were averaged.38 These count values were used to obtain the total number of spermatids per testis or epididymis, which was then divided by the testis or epididymis weight to determine the number per g of testis or epididymis.
Lipid peroxidation
The lipid peroxidation, estimated from the malondialdehyde (MDA) content was measured by using the method of Buege and Aust.39 Briefly, homogenates of testis, epididymis and sperm were centrifuged at 1000 g for 10 min at 4 °C to separate cell debris. The supernatants were suspended in PBS (pH = 7.4), mixed with BHT–TCA solution (1% BHT dissolved in 20% TCA w/v), centrifuged at 1000g for 35 min and finally mixed with 0.5 N HCl and 120 mM TBA (thiobarbituric acid) in 26 mM Tris and heated in a water bath at 80 °C for 10 min. After cooling, the absorbance of the resulting chromophore was measured at 532 nm. MDA levels were determined by using an extinction coefficient of 1.56 × 105 M−1 cm−1 for the MDA–TBA complex.
Thiol group measurement
The total concentration of thiol groups (–SH) was measured according to Ellman's method.40 Briefly, aliquots from liver tissue were mixed with 100 μL of 10% SDS and 800 μL of 10 mM phosphate buffer (pH 8) and the absorbance was measured at 412 nm (A0). Then, 100 μL of DTNB was added and incubated at 37 °C for 60 min. After incubation, the absorbance of the sample was measured at 412 nm (A1). The thiol group concentration was calculated using A1 − A0 subtraction using a molar extinction coefficient of 13.6 × 103 M−1 cm−1. The results were expressed as nmol of thiol groups per mg of protein.
Antioxidant activity assays
SOD activity was determined using a modified version of the epinephrine assay reported by Misra and Fridovich.41 At alkaline pH, the superoxide anion O2− causes the autoxidation of epinephrine to adenochrome. Meanwhile, SOD competes with this reaction, decreasing adenochrome formation. One unit of SOD is defined as the amount of the extract that inhibits the rate of adenochrome formation by 50%. Enzyme extract was added to a 2 mL reaction mixture containing 10 μL of bovine catalase (0.4 U μL−1), 20 μL epinephrine (5 mg mL−1) and 62.5 mM sodium carbonate/bicarbonate buffer (pH 10.2). Changes in absorbance were recorded at 480 nm. Characterization of SOD isoforms was performed using KCN (2 mM), which inhibits Cu/Zn-SOD and H2O2 (5 mM), affecting both Cu/Zn-SOD and Fe-SOD, whereas Mn-SOD was insensitive to both inhibitors.42
CAT activity was assayed by measuring the initial rate of H2O2 disappearance at 240 nm.43 The reaction mixture contained 33 mM H2O2 in 50 mM phosphate buffer (pH 7) and CAT activity was calculated using the extinction coefficient of 40 mM−1 cm−1 for H2O2.
GPx activity was measured using the procedure of Flohé and Günzler.44 Briefly, 1 mL of reaction mixture containing 0.2 mL of sample supernatant, 0.2 mL of phosphate buffer (0.1 M, pH 7.4), 0.2 mL of GSH (4 mM) and 0.4 mL of H2O2 (5 mM) was incubated at 37 °C for 1 min and the reaction was stopped by the addition of 0.5 mL TCA (5%, w/v). After centrifugation at 1500g for 5 min, an aliquot (0.2 mL) from the supernatant was mixed with 0.5 mL of phosphate buffer (0.1 M, pH 7.4) and 0.5 mL DTNB (10 mM) and the absorbance was recorded at 412 nm. GPx activity was expressed as nmol of GSH consumed per min per mg protein.
Histopathological examination
Immediately after sacrificing the rats, small pieces of testis were collected and washed with ice-cold saline. Tissue fragments were then fixed in a 10% neutral buffered formalin solution, embedded in paraffin and used for histopathological examination.
5 mm thick sections were cut, deparaffinised, hydrated and stained with hematoxylin and eosin (HE). The testis sections were examined for the control, malathion and essential oil treated groups.
Protein determination
Protein concentration was determined according to the Bradford method using BSA as the standard.45
Statistical analysis
Data were analyzed using an unpaired student's t-test or one-way analysis of variance (ANOVA) and were expressed as the mean ± standard error of the mean (SEM). Data are representative of 10 independent experiments. All statistical tests were two-tailed, and a p value of 0.05 or less was considered significant.
Results
Chemical composition of Artemisia herba-alba and Eucalyptus camaldulensis essential oils
The hydrodistillation of the air-dried aerial parts yielded pale yellowish oils in 1.18 and 1.34% (w/w, on dry weight basis) for A. herba-alba (AhaEO) and E. camaldulensis (EcEO), respectively.
The identity, retention index and composition percentages of the essential oils are listed in Table 1. A total of 39 components covering more than 99% of the total integrated GC profiles were identified (Table 1). The most plentiful constituents of AhaEO were oxygenated monoterpenes (74.25% of the total essential oil). Amongst their derivatives, β-thujone (23.92%), chrysanthenone (17.4%) and α-thujone (10.34%) were the major compounds. Monoterpene hydrocarbons were found to be the second main chemical class (20.7% of the total essential oil) with p-cymene (8.27%), sabinene (3.31%) and α-pinene (3.25%) being the main compounds. The rather small fraction of sesquiterpenes (4.6%) (both hydrocarbon and oxygenated forms) was dominated by β-caryophyllene (1.2%), germacrene-D (1.02%) and caryophyllene oxide (0.73%).
Table 1 Chemical composition (% total peak area) of the essential oils of E. camaldulensis and Artemisia herba-alba
No. |
Compounds |
RIa |
E. camaldulensis |
A. herba-alba |
RI: Retention Index on HP-5 column; values in bold indicate the main components; —: not detected. |
Monoterpene hydrocarbons |
1 |
α-Thujene |
932 |
0.12 |
— |
2 |
α-Pinene |
939 |
1.87 |
3.25 |
3 |
Sabinene |
964 |
2.3 |
3.31 |
4 |
β-Pinene |
983 |
1.34 |
1.39 |
5 |
β-Myrcene |
992 |
2.3 |
2.34 |
6 |
α-Phellandrene |
998 |
— |
0.12 |
7 |
3-Carene |
1006 |
— |
0.14 |
8 |
p-Cymene |
1015 |
7.23 |
8.27 |
9 |
α-Terpinene |
1018 |
— |
0.14 |
10 |
trans-β-Ocimene |
1038 |
0.98 |
0.99 |
11 |
γ-Terpinene |
1062 |
0.24 |
0.38 |
12 |
α-Terpinolene |
1076 |
0.16 |
0.37 |
Total |
16.54 |
20.7 |
![[thin space (1/6-em)]](https://www.rsc.org/images/entities/char_2009.gif) |
Oxygenated monoterpenes |
13 |
1,8-Cineole |
1033 |
12.58 |
9.78 |
14 |
Linalool |
1101 |
1.47 |
1.48 |
15 |
β-Thujone |
1103 |
— |
23.92 |
16 |
Camphor |
1145 |
1.19 |
— |
17 |
β-Terpineol |
1146 |
0.35 |
— |
18 |
Terpinen-4-ol |
1164 |
0.23 |
5.23 |
19 |
p-Menth-1-en-8-ol |
1183 |
5.12 |
4.7 |
20 |
Cryptone |
1189 |
9.38 |
— |
21 |
α-Thujone |
1194 |
— |
10.34 |
22 |
p-Cymen-8-ol |
1196 |
1.72 |
— |
23 |
Chrysanthenone |
1253 |
— |
17.4 |
24 |
Geraniol |
1255 |
0.13 |
— |
25 |
cis-Chrysanthenyl acetate |
1263 |
— |
0.12 |
27 |
Neryl acetate |
1356 |
0.19 |
1.28 |
Total |
32.36 |
74.25 |
![[thin space (1/6-em)]](https://www.rsc.org/images/entities/char_2009.gif) |
Sesquiterpene hydrocarbons |
26 |
β-Cubebene |
1349 |
— |
0.05 |
28 |
β-Elemene |
1389 |
0.61 |
0.57 |
29 |
β-Caryophyllene |
1420 |
1.23 |
1.2 |
30 |
β-Farnesene |
1453 |
0.05 |
0.11 |
31 |
α-Humulene |
1456 |
0.09 |
0.09 |
32 |
Germacrene-D |
1477 |
1.01 |
1.02 |
33 |
α-Amorphene |
1504 |
— |
0.06 |
Total |
2.99 |
3.1 |
![[thin space (1/6-em)]](https://www.rsc.org/images/entities/char_2009.gif) |
Oxygenated sesquiterpenes |
34 |
Elemol |
1547 |
0.6 |
0.55 |
35 |
Ledol |
1563 |
0.23 |
0.22 |
36 |
Caryophyllene oxide |
1569 |
2.38 |
0.73 |
37 |
Spathulenol |
1576 |
22.66 |
— |
38 |
Globulol |
1603 |
2.87 |
— |
39 |
γ-Eudesmol |
1629 |
18.42 |
— |
Total |
47.16 |
1.5 |
![[thin space (1/6-em)]](https://www.rsc.org/images/entities/char_2009.gif) |
Miscellaneous |
40 |
n-Nonadecanoic acid |
2220 |
0.05 |
— |
Total identified |
99.1 |
99.55 |
In EcEO, the main contributors were found to be oxygenated sesquiterpenes (47.16% of the total essential oil). In this fraction, spathlenol (22.66%) and γ-eudesmol (18.42%) were the basic constituents. Oxygenated monoterpenes represented 32.36% of the oil and consisted mainly of 1,8-cineole (12.58%), cryptone (9.38%) and p-menth-1-en-8-ol (5.12%). Monoterpene hydrocarbons constituted 16.54% of the total essential oil, the main compounds being p-cymene (7.23%), myrcene (2.3%) and sabinene (2.3%). A lower amount of sesquiterpene hydrocarbons (2.99%) and a trace amount of miscellaneous compounds (0.05%) were also detected in EcEO.
Free radical scavenging activity of AhaEO and EcEO
Concerning the antioxidant capacity, we showed that AhaEO and EcEO present an important RSA (EC50 = 121.43 ± 4.27 and 254.69 ± 2.12 μg mL−1, respectively) but this is less than the standard BHT (EC50 = 108.24 ± 3.78 μg mL−1) (Table 2).
Table 2 EC50 values of DPPH radical-scavenging activity in AHAEO, ECEO and BHT
|
AHAEO |
ECEO |
BHT |
EC50 is the effective concentration of sample that can decrease DPPH concentration by 50%. |
EC50a of DPPH-RSA (μg mL−1) |
121.43 ± 4.27 |
254.69 ± 2.12 |
108.24 ± 3.78 |
Mortality and macroscopic symptoms of toxicity
In the present study, no mortality was noted until the end of the experiments in the control and treated groups. Concerning the testis and the reproductive accessories, no macroscopic alterations were found throughout the experiment. However, cholinergic signs of adverse toxicological effects such as sluggishness, muscular tremors, irregular movements, and abdominal trembling were observed in the malathion-treated group. The progression of these signs proceeded to the last week of treatment. AhaEO and EcEO administration reversed all adverse cholinergic effects induced by malathion intoxication.
Body and reproductive relative organ weights
Data from Table 3 shows that the malathion-treated rats demonstrated a significant decrease in body weight, in addition to a decrease in the relative weights of the testis, epididymis, prostate and seminal vesicles. Administration of AhaEO and EcEO prevented malathion-induced body weight decrease significantly (p < 0.05), while also causing an increase in the relative weight of the reproductive organs.
Table 3 Sub-acute effect of AHAEO and ECEO on malathion induced changes in body weight as well as testis, epididymis, prostate and seminal vesicle relative weights. Animals were treated for 21 days with AHAEO (50 mg kg−1 b.w., o.p.) or ECEO (50 mg kg−1 b.w., o.p.). Assays were carried out in triplicate
|
CTR |
M |
AHAEO |
ECEO |
M + AHAEO |
M + ECEO |
p < 0.05 compared to control (CTR) group. p < 0.05 compared to Malathion (M) group. |
Initial body weight (g) |
230 ± 1.82 |
232 ± 1.43 |
231 ± 2.78 |
229 ± 2.29 |
232 ± 2.25 |
232 ± 2.61 |
Final body weight (g) |
271 ± 2.63 |
243 ± 2.39a |
274 ± 1.62 |
269 ± 2.63 |
264 ± 2.71b |
265 ± 1.39b |
Epididymis (g/100 g b.w.) |
0.57 ± 0.17 |
0.39 ± 0.13a |
0.56 ± 0.15 |
0.59 ± 0.14 |
0.51 ± 0.09b |
0.49 ± 0.12b |
Testis (g/100 g b.w.) |
1.69 ± 0.12 |
1.38 ± 0.09a |
1.73 ± 0.07 |
1.72 ± 0.15 |
1.66 ± 0.07b |
1.63 ± 0.19b |
Prostate (g/100 g b.w.) |
0.42 ± 0.02 |
0.33 ± 0.03a |
0.43 ± 0.02 |
0.42 ± 0.01 |
0.39 ± 0.03b |
0.38 ± 0.03b |
Seminal vesicle (g/100 g b.w.) |
1.25 ± 0.23 |
1.11 ± 0.21a |
1.26 ± 0.07 |
1.25 ± 0.16 |
1.20 ± 0.18b |
1.19 ± 0.13b |
Evaluation of reproductive performance quality
The effects of malathion and essential oils on the reproductive performance quality are reported in Table 4. As expected, malathion administration induced a decrease in the epididymal spermatozoa count and testicular spermatid enumeration, as well as a reduction of sperm motility, while the number of dead sperm increased significantly. The AhaEO and EcEO treatment reversed all sperm parameters.
Table 4 Subacute effect of AHAEO and ECEO on malathion induced changes in reproductive performance quality. Animals were treated for 21 days with AHAEO or ECEO (50 mg kg−1 b.w., o.p.) in corn oil. Assays were carried out in triplicate
|
CTR |
M |
AHAEO |
ECEO |
M + AHAEO |
M + ECEO |
p < 0.05 compared to control (CTR) group. p < 0.05 compared to Malathion (M) group. |
Sperm concentration (106/mL) |
59.16 ± 1.14 |
35.28 ± 1.08a |
57.19 ± 2.04 |
57.15 ± 3.18 |
53.13 ± 2.63b |
52.18 ± 1.39b |
Motility (%) |
86.14 ± 2.21 |
47.18 ± 2.43a |
86.26 ± 2.03 |
84.83 ± 2.47 |
69.76 ± 1.93b |
65.47 ± 1.86b |
Viability (%) |
87.31 ± 1.04 |
45.17 ± 1.26a |
87.23 ± 1.66 |
86.12 ± 1.92 |
71.16 ± 1.83b |
73.14 ± 1.56b |
Morphology changes (%) |
5.23 ± 0.47 |
19.34 ± 1.42a |
4.28 ± 0.83 |
4.25 ± 0.62 |
16.27 ± 2.32b |
17.63 ± 2.72b |
Sperm count/(g) epididymis (106) |
118.74 ± 5.34 |
89.42 ± 5.71a |
114.54 ± 4.40 |
114.6 ± 4.20 |
106.26 ± 3.50b |
104.28 ± 5.95b |
Spermatid count/g testis (106) |
58.21 ± 4.66 |
34.14 ± 3.66a |
59.49 ± 5.52 |
56.26 ± 3.23 |
52.17 ± 4.31b |
51.19 ± 4.74b |
Evaluation of testosterone levels as well as AChE and BChE activity
In addition to the biochemical findings, we were also interested in cholinesterase activity and the testosterone levels in the plasma compartment (Table 5). We showed that malathion treatment significantly (p < 0.05) reduced the plasma AChE and BChE activity as well as the testosterone levels. Administration of AhaEO and EcEO had a beneficial effect on the levels of AChE and BChE activity and testosterone, which were found to be elevated in the essential oil-treated groups when compared with the malathion-treated one.
Table 5 Subacute effect of AHAEO and ECEO on malathion-induced changes in plasma glucose, testosterone and cholinesterase activity. Animals were treated for 21 days with AHAEO or ECEO (50 mg kg−1 b.w., o.p.) in corn oil. Assays were carried out in triplicate
|
CTR |
M |
AHAEO |
ECEO |
M + AHAEO |
M + ECEO |
p < 0.05 compared to control (CTR) group. p < 0.05 compared to Malathion (M) group. |
Glucose (mM) |
6.72 ± 0.66 |
16.87 ± 1.21a |
5.45 ± 0.84 |
5.17 ± 0.6 |
7.22 ± 0.45b |
7.35 ± 0.84b |
Testosterone (ng mL−1) |
8.73 ± 1.3 |
4.39 ± 0.63a |
8.93 ± 1.06 |
8.54 ± 1.19 |
6.41 ± 0.96b |
7.08 ± 0.86b |
AChE activities (nmol mg−1 protein) |
64.36 ± 5.73 |
33.69 ± 2.13a |
63.08 ± 4.66 |
62.67 ± 5.42 |
50.27 ± 3.19b |
49.78 ± 5.37b |
BChE activities (nmol mg−1 protein) |
53.89 ± 2.54 |
28.41 ± 1.81a |
57.74 ± 2.92 |
55.38 ± 3.43 |
43.67 ± 2.62b |
40.28 ± 4.56b |
Lipid peroxidation, thiol group content and H2O2 levels
The effects of malathion and essential oils on oxidative status expressed in terms of MDA, H2O2 and thiol groups in the testis, epididymis and sperm are shown in Fig. 1–3. As observed, malathion treatment was accompanied by a significant (p < 0.05) increase in MDA and H2O2 concentration versus a decrease in the thiol group concentration. Treatment with AhaEO and EcEO protected against lipoperoxidation and the increase in hydrogen peroxide and decrease in –SH groups, induced by the malathion treatment.
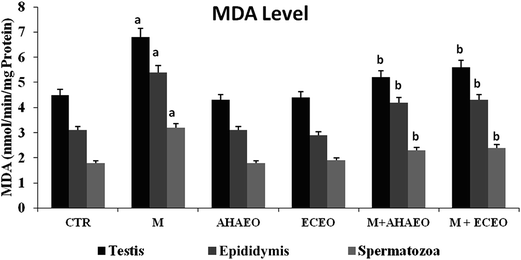 |
| Fig. 1 Subacute effect of AhaEO and EcEO on subacute malathion-induced changes in malondialdehyde. Animals were treated for 21 days with AHAEO (50 mg kg−1 b.w., o.p.), ECEO (50 mg kg−1 b.w., o.p.). Assays were carried out in triplicate. (a) p < 0.05 compared to healthy control (CTR) group. (b) p < 0.05 compared to malathion treated (M) group. | |
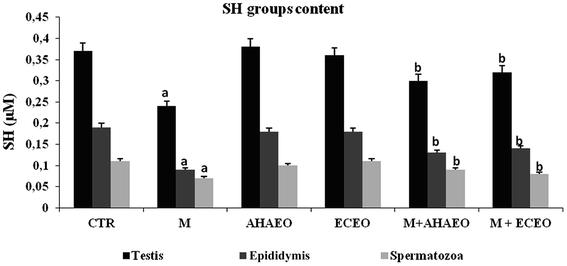 |
| Fig. 2 Subacute effect of AhaEO and EcEO on subacute malathion-induced changes in sulfhydril group levels. Animals were treated for 21 days with AHAEO (50 mg kg−1 b.w., i.p.), ECEO (50 mg kg−1 b.w., o.p.). Assays were carried out in triplicate. (a) p < 0.05 compared to healthy control (CTR) group. (b) p < 0.05 compared to malathion treated (M) group. | |
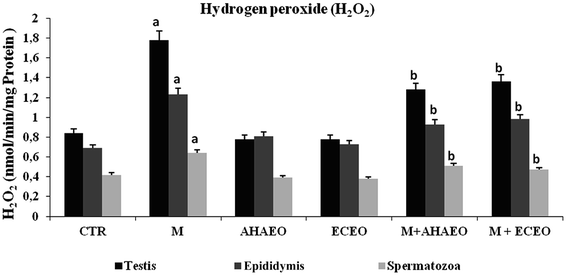 |
| Fig. 3 Subacute effect of AhaEO and EcEO on subacute malathion-induced changes in hydrogen peroxide levels. Animals were treated for 21 days with AHAEO (50 mg kg−1 b.w., o.p.), ECEO (50 mg kg−1 b.w., o.p.). Assays were carried out in triplicate. (a) p < 0.05 compared to healthy control (CTR) group. (b) p < 0.05 compared to malathion treated (M) group. | |
Antioxidant enzyme activity
The effects of malathion and essentials oils on antioxidant enzyme activity are reported in Fig. 4–7. Malathion per se significantly (p < 0.05) decreased the activity of GPx, CAT, total SOD, Cu/Zn-SOD, and Mn-SOD in the testis, epididymis and spermatozoa. The subacute treatment with AhaEO and EcEO protected against the depletion of antioxidant enzyme activity induced by malathion intoxication. AhaEO and EcEO alone significantly (p < 0.05) increased antioxidant enzyme activity.
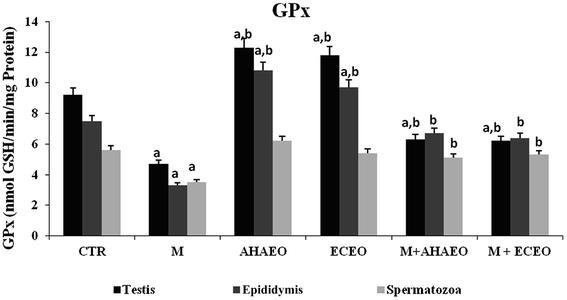 |
| Fig. 4 Subacute effect of AhaEO and EcEO on subacute malathion-induced changes in glutathione peroxidise activity. Animals were treated for 21 days with AHAEO (50 mg kg−1 b.w., o.p.), ECEO (50 mg kg−1 b.w., o.p.). Assays were carried out in triplicate. (a) p < 0.05 compared to healthy control (CTR) group. (b) p < 0.05 compared to malathion treated (M) group. | |
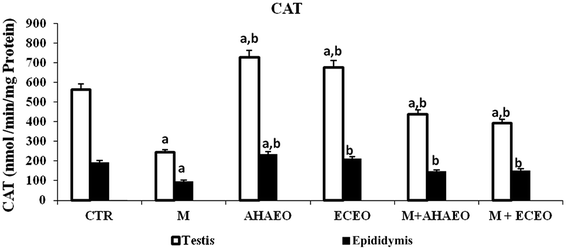 |
| Fig. 5 Subacute effect of AhaEO and EcEO on subacute malathion-induced changes in catalase activity. Animals were treated for 21 days with AHAEO (50 mg kg−1 b.w., o.p.), ECEO (50 mg kg−1 b.w., o.p.). Assays were carried out in triplicate. (a) p < 0.05 compared to healthy control (CTR) group. (b) p < 0.05 compared to malathion treated (M) group. | |
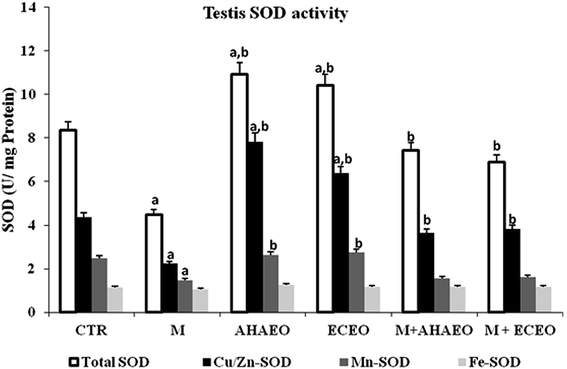 |
| Fig. 6 Subacute effect of AhaEO and EcEO on subacute malathion-induced changes in testis superoxide dismutase and the activities of its isoforms. Animals were treated for 21 days with AHAEO (50 mg kg−1 b.w., o.p.), ECEO (50 mg kg−1 b.w., o.p.). Assays were carried out in triplicate. (a) p < 0.05 compared to healthy control (CTR) group. (b) p < 0.05 compared to malathion treated (M) group. | |
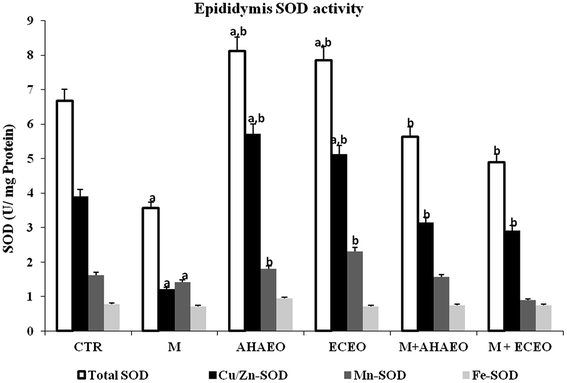 |
| Fig. 7 Subacute effect of AhaEO and EcEO on subacute malathion-induced changes in epididymis superoxide dismutase and the activities of its isoforms. Animals were treated for 21 days with AHAEO (50 mg kg−1 b.w., o.p.), ECEO (50 mg kg−1 b.w., o.p.). Assays were carried out in triplicate. (a) p < 0.05 compared to healthy control (CTR) group. (b) p < 0.05 compared to malathion treated (M) group. | |
Testis and epididymis histopathology
The histological results reported in the current study confirmed the biochemical results. In light microscopic examinations, malathion exposed rats showed severe histopathological changes in the testis, such as atrophic seminiferous tubules (Fig. 8B). The affected tubules were lined by fewer spermatogenic cells. In addition, signs of spermiotoxicity as revealed by the appearance of multinucleated giant cells, vacuolization in Sertoli cells and loss of germ cells were observed (Fig. 8C). However, the essential oil administration to the malathion-treated rats normalized the testis histopathological alterations induced by malathion treatment (Fig. 9A–D). The testes of the control group (Fig. 9A) and the animals administrated with essential oils (Fig. 9C and D) showed a normal histological structure.
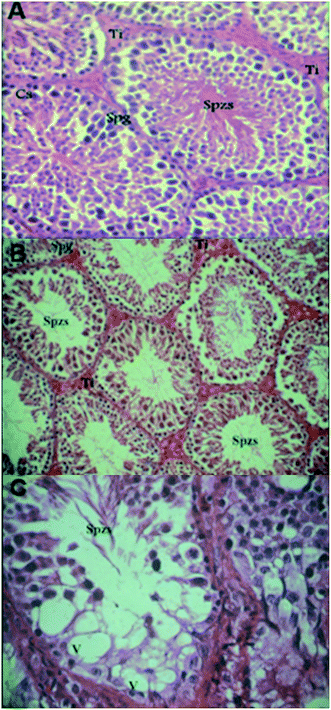 |
| Fig. 8 Testicular sections of control rat that show normal spermatogenesis (A) note the normal cell arrangement in the seminiferous tubules. The interstitial spaces also appear normal: Ti, interstitium; Sg, spermatogonia; Sd, spermatid; Spzs, spermatozoa; SC, Sertoli cell (×200 H&E). Testicular sections of rat treated with 200 mg per kg per day of malathion: note the atrophic seminiferous tubules (B) (×400 H&E). Sloughing of germ cells into tubular lumen, V, vacuolization in Sertoli cells and (arrow) multinucleated giant cell (C) (×1000 H&E). | |
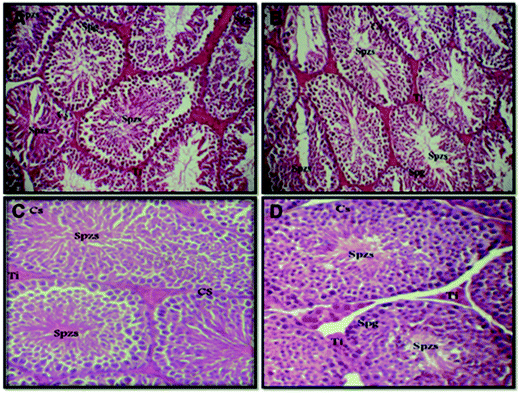 |
| Fig. 9 Testicular sections of rat treated with 200 mg per kg per day of malathion and 50 mg kg−1 AHAEO (A) or malathion + ECEO (B) note the increase of germ cells in the seminiferous tubules. The interstitial spaces also appear normal; Ti, interstitium; Sg, spermatogonia; Sd, spermatid; Spzs, spermatozoa; SC, Sertoli cell (×400 H&E). Testicular sections of rat treated with AHAEO (C) or ECEO (D) note the normal cell arrangement in the seminiferous tubules. The interstitial spaces also appear normal: Ti, interstitium; Sg, spermatogonia; Sd, spermatid; Spzs, spermatozoa; SC, Sertoli cell (×400 H&E). | |
Discussion
In the present investigation, the chemical composition and the protective effects of AhaEO and EcEO against malathion-induced damage and oxidative stress on the reproductive function of male rats were studied.
The analytical data revealed that oxygenated monoterpenes were the main contributors in AhaEO and the chief components of the oil were β-thujone, chrysanthenone and α-thujone. The oxygenated sesquiterpenes, spathulenol and γ-eudesmol, as well as the oxygenated monoterpene cryptone, were found to be the basic constituents of EcEO. When compared with earlier compositional investigations, the main components identified have already been reported in both species, but with different percentage composition due to differences in the genetic background, plant part, growing region, season, storage time and extraction and analytical procedures.46,47
Evaluation of the free radical scavenging activity of the tested essential oils shows that AhaEO and EcEO exhibited a good activity against the DPPH radical. The high DPPH scavenging capacity of both oils can be attributed to their high content of oxygenated compounds (mono- and sesquiterpenes) (e.g. 1,8-cineole, linalool, camphor, terpinen-4-ol, and spathulenol, among others), recognized for their potent antioxidant activity.46 When compared with earlier studies, the EC50 value observed for AhaEO was nearly the same as those reported for A. herba-alba (EC50 = 140 μg mL−1) collected in southern Tunisia, where chrysantenyl acetate and sabinene acetate were the major components.48 However, it was much lower than that reported (5030 μg mL−1) for a β-thujone chemotype collected in central Tunisa.49 For EcEO, the EC50 value obtained was much lower than those observed for a p-cymene-rich oil of E. camaldulensis from Thailand, where values ranging from 1750 to 12
620 μg mL−1 were recorded.50 In addition to the differences in the chemical composition of the essential oils and the possible antagonistic and synergistic interactions of additives, the experimental protocol (solvent, pH, incubation time etc.) and the measurement methodology may explain the observed discrepancies.
The in vitro assessment of AChE and BChE activity revealed that malathion treatment reduced both parameters. The decrease of cholinesterase activity might be due, at least in part, to the inhibition of the enzyme synthesis by the altered brain cells.51 Moreover, the reduction of AChE and BChE activity is likely responsible for the observed cholinergic signs induced by malathion, which include sluggishness, muscular tremors, irregular movements and convulsion in the malathion-treated group. Another point to be considered is that the inhibition of AChE and BChE can drastically decrease the sperm motility and eventually lead to cell death as observed in this study.52
In contrast, the administration of AhaEO and EcEO restores the malathion-induced inhibition of plasma AChE and BChE. At this point, it seems that the antioxidant properties of both essential oils were responsible for the observed protective effects on AChE and BChE. Support for this assumption is given by Muthuvel et al., who found that ascorbic acid restores parachlorobenzene (PCB)-induced AChE inhibition in albino rats.53 One year later, the protective effects of Satureja khuzestanica essential oil on AChE was also confirmed in malathion-induced red blood cell AChE inhibition.54 The hazardous effects of malathion also include a reduction in testosterone levels. As an immediate consequence of testosterone depletion, a drastic reduction in body and reproductive organ weight was recorded. Such symptoms seem to be typical to malathion exposure as evidenced in many studies. In this context, it has been found that the loss of body and reproductive organ weight in response to malathion or organophosphorus compound exposure were attributed to the suppression of food intake or hormonal imbalance (LH, FSH and testosterone) in the hypothalamo-hypophysial-testicular axis.55
In confirmation of these observations, microscopic examinations pinpointed serious histological alterations and disturbance of spermatogenesis in malathion-treated rats, as evidenced by the decrease of epididymal sperm count, motility and viability, increase of abnormal sperm morphology, vacuolization in Sertoli cells and loss of germ cells. These results were mainly attributed to the reduction in testosterone levels, and therefore, an imbalance in the testis regulation and consequent reduction in the secretion of pituitary gonadorophins (FSH and LH).56,57 It has previously been reported that the reduction of reproductive organ weight and their histopathological alterations in response to malathion administration are associated, on one hand, with disturbed activity of testicular enzymes, such as acid phosphatase (ACP), lactate dehydrogenase (LDH), succinate dehydrogenase (SDH) and γ-glutamyl transpeptidase (γ-GT) and, on the other hand, with the induction of apoptosis-related gene expression, which leads ultimately to the reduction of spermatogenic cells and consequently the reduction in sperm count, motility and viability, as observed herein.56,57
These symptoms were exacerbated by a remarkable increase in lipid peroxidation (increased MDA content) and the generation of H2O2 in all reproductive organs, with a concomitant depletion of antioxidant enzymes, namely SOD, CAT and GPx. These results are consistent with previously reported data, where it has been found that the reduction in male reproductive performance (sperm, histopathological and cytopathological parameters) in response to malathion and other organophosphorus compounds is positively correlated with oxidative stress.52,55 The administration of AhaEO and EcEO restores all of these parameters, indicating their protective effects against oxidative stress induced by malathion treatment. Moreover, the administration of AhaEO and EcEO normalized testosterone levels, presumably via their direct effects on the central nervous system and gonadal tissues, or their effects on the hypothalamo-hypophysial-testicular axis as reported for other essential oils such as marjoram and lavender.58 The increased testosterone levels may be responsible for the restoration of spermatogenesis and histopathological parameters.
It is well known that sperm cell membranes are rich in polyunsaturated fatty acids and are very susceptible to free radical attack. Lipid peroxidation of sperm cell membranes is one type of cell damage induced by ROS, which causes an increase in membrane permeability, an interruption in the respiratory chain, and ATP production, as well as a decrease in the phosphorylation of axonemal proteins.59 Malathion-induced decrease of sperm quality might be due to the depletion of antioxidant enzyme activity and/or ROS accumulation. The free radical-induced decease in sperm motility was presumably due to a rapid loss of intracellular ATP, which altered axoneme structure and caused tail abnormality.60 Injury of cell DNA induced by excessive ROS led to the production of some peroxidation products such as 8-oxo-7,8-dihydroxyguanosine, which causes fragmentation and has a mutagenic effect.61 High levels of sperm DNA damage have been reported to decrease male fertility, while antioxidants could be used to alleviate male infertility.62
These effects previously described were significantly mitigated by co-treating with 50 mg kg−1 b.w. essential oils, compared with M-treated group. However, in the essential oil supplemented groups, the extent of lipid peroxidation and the concentration of protein SH groups were significantly reduced, compared with the malathion-treated group. Our results show that malathion induced oxidative stress in the rat testis may be successfully treated with AhaEO and EcEO, due to their antioxidant effects.63 In this setting, essential oils prevented testicular oxidative damage, reduced lipid peroxidation and improved total sperm motility, viability and morphology in rat spermatozoa. These effects are similar to that observed for other antioxidants like ascorbic acid and vitamin E.64
To the best of our knowledge, this study is the first to investigate the in vivo antioxidant effect of AhaEO and EcEO on rat sperm kinematic parameters.
Conclusion
In conclusion, our data confirm the deleterious effects of malathion on male reproduction and clearly demonstrate that AhaEO and EcEO exert protective effects through their potential antioxidant activity, by increasing AChE, BChE, antioxidant enzyme activity, and testosterone levels. Consequently, the use of these oils as potential therapeutics for the treatment of male infertility associated with malathion exposure may be a promising alternative.
Acknowledgements
Financial support of the Tunisian Ministry of Higher Education and Scientific Research is gratefully acknowledged. Financial disclosures: none declared.
References
- I. Figa-Talamanca, M. E. Traina and E. Urbani, London: occupational exposures to metals, solvents and pesticides: recent evidence on male reproductive effects and biological markers, Occup. Med., 2001, 51, 174–188 CrossRef CAS PubMed.
- H. Wang, S. F. Wang, H. Ning, Y. L. Ji, C. Zhang, Y. Zhang, T. Yu, X. H. Ma, X. F. Zhao, Q. Wang, P. Liu, X. H. Meng and D. X. Xu, Maternal cypermethrin exposure during lactation impairs testicular development and spermatogenesis in male mouse offspring, Environ. Toxicol., 2011, 26(4), 382–394 CrossRef CAS PubMed.
- S. Selmi, S. El-Fazaa and N. Gharbi, Oxidative stress and alteration of biochemical markers in liver and kidney by malathion in rat pups, Toxicol. Ind. Health, 2013, 1–7 Search PubMed.
- F. G. Uzun, S. Kalender, D. Durak, F. Demir and Y. Kalender, Malathion-induced testicular toxicity in male rats and the protective effect of vitamins C and E, Food Chem. Toxicol., 2009, 47, 1903–1908 CrossRef CAS PubMed.
- M. D. Saulsbury, S. O. Heyliger, K. Wang and D. J. Johnson, Chlorpyrifos induces oxidative stress in oligodendrocyte progenitor cells, Toxicology, 2009, 259, 1–9 CrossRef CAS PubMed.
- F. Demir, F. G. Uzun, D. Durak and Y. Kalender, Subacute chlorpyrifos-induced oxidative stress in rat erythrocytes and the protective effects of catechin and quercetin, Pestic. Biochem. Physiol., 2011, 99, 77–81 CrossRef CAS.
- E. Mutch and F. M. Williams, Diazinon, chlorpyrifos and parathion are metabolised by multiple cytochromes P450 in human liver, Toxicology, 2006, 224, 22–32 CrossRef CAS PubMed.
- R. Rezg, B. Mornagui, S. El-Fazaa and N. Gharbi, Biochemical evaluation of hepatic damage in subchronic exposure to malathion in rats: effect on superoxide dismutase and catalase activities using native PAGE, C. R. Biol., 2008, 331, 655–662 CrossRef CAS PubMed.
- S. Selmi, S. El-Fazaa and N. Gharbi, Oxidative stress and cholinesterase inhibition in plasma, erythrocyte and brain of rats' pups following lactational exposure to malathion, Environ. Toxicol. Pharmacol., 2012, 34(3), 753–760 CrossRef CAS PubMed.
- K. Randerath, E. Randerath, C. V. Smith and J. Chang, Structural origins of bulky oxidative DNA adducts (type II I-compounds) as deduced by oxidation of oligonucleotides of known sequence, Chem. Res. Toxicol., 1996, 9, 247–254 CrossRef CAS PubMed.
- R. J. Mallis, J. E. Buss and J. A. Thomas, Oxidative modification of Hras: S-thiolation and S-nitrosylation of reactive cysteines, J. Biochem., 2001, 355, 145–153 CrossRef CAS.
- L. Morel, G. Lescoat, J. Cillard, N. Pasdeloup, P. Brissot and P. Cillard, Kinetic evaluation of free malondialdehyde and enzyme leakage as indices of iron damage in rat hepatocyte cultures. Involvement of free radicals, Biochem. Pharmacol., 1990, 39, 1647–1655 CrossRef PubMed.
- F. Bakkali, S. Averbeck, D. Averbeck and M. Idaomar, Biological effects of essential oils – a review, Food Chem. Toxicol., 2008, 46, 446–475 CrossRef CAS PubMed.
- R. Belhattab, L. Amor, J. G. Barroso, L. G. Pedro and A. Cristina Figueiredo, Essential oil from Artemisia herba-alba Asso grown wild in Algeria: variability assessment and comparison with an updated literature survey, Arabian J. Chem., 2014, 7, 243–251 CrossRef CAS.
- G. Vernin, O. Merad, G. M. F. Vernin, R. M. Zamkotsian and C. Parkanyi, GC-MS analysis of Artemisia herba alba Asso essential oils from Algeria, Dev. Food Sci., 1995, 37, 147–205 CrossRef.
- C. Bailey and A. Danin, Bedouin plant utilization in Sinai and the Negev, Econ. Bot., 1981, 35, 145–162 CrossRef.
- H. Jouad, M. Haloui, H. Rhiouani, J. El Hilaly and M. Eddouks, Ethnobotanical survey of medicinal plants used for the treatment of diabetes, cardiac and renal diseases in the North centre region of Morocco (Fez-Boulemane), J. Ethnopharmacol., 2001, 77, 175–182 CrossRef CAS PubMed.
- J. Yashphe, R. Segal, A. Breuer and G. Erdreich-Naftali, Antibacterial activity of Artemisia herba-alba, J. Pharm. Sci., 1979, 68, 924–925 CrossRef CAS PubMed.
- S. Hatimi, M. Boudouma, M. Bichichi, N. Chaib and N. G. Idrissi, Evaluation in vitro of antileishmanien activity of Artemisia herba-alba Asso. Franco-African meeting of pediatrics, 2000, pp. 57–70 Search PubMed.
- E. L. Ghisalberti, Bioactive acylphloroglucinol derivatives from Eucalyptus species, Phytochemistry, 1996, 41, 7–22 CrossRef CAS PubMed.
- A. Y. Leung and S. Foster, Encyclopedia of Common Natural Ingredients Used in Food, Drugs, and Cosmetics, John Willey & Sons, New York, NY, USA, 2nd edn, 1996 Search PubMed.
- K. Cimanga, K. Kambu, L. Tona, S. Apers, T. de Bruyne, N. Hermans, J. Totté, L. Pieters and A. J. Vlietinck, Correlation between chemical composition and antibacterial activity of essential oils of some aromatic medicinal plants growing in the Democratic Republic of Congo, J. Ethnopharmacol., 2002, 79, 213–220 CrossRef CAS PubMed.
- J. Silva, W. Abebe, S. M. Sousa, V. G. Duarte, M. I. L. Machado and F. J. A. Matos, Analgesic and anti-inflammatory effects of essential oils of Eucalyptus, J. Ethnopharmacol., 2003, 89, 277–283 CrossRef CAS PubMed.
- P. Siramon, Y. Ohtani and H. Ichiura, Biological performance of Eucalyptus camaldulensis leaf oils from Thailand against the subterranean termite Coptotermes formosanus Shiraki, J. Wood Sci., 2009, 55, 41–46 CrossRef CAS.
- S. S. Cheng, C. G. Huang, Y. J. Chen, J. J. Yu, W. J. Chen and S. T. Chang, Chemical compositions and larvicidal activities of leaf essential oils from two Eucalyptus species, Bioresour. Technol., 2009, 100, 452–456 CrossRef CAS PubMed.
- K. Watanabe, Y. Shono, A. Kakimizu, A. Okada, N. Matsuo, A. Satoh and H. Nishimura, New mosquito repellent from Eucalyptus camaldulensis, J. Agric. Food Chem., 1993, 41, 2164–2166 CrossRef CAS.
- P. Siramon and Y. Ohtani, Antioxidative and antiradical activities of Eucalyptus camaldulensis leaf oils from Thailand, J. Wood Sci., 2007, 53, 498–504 CrossRef CAS.
- I. Grzegorczyk, A. Matkowski and H. Wysokinska, Antioxidant activity of extracts from in vitro cultures of Salvia officinalis L, Food Chem., 2007, 104, 536–541 CrossRef CAS.
- G. A. Ellman, New and rapid colorimetric determination of acetylcholinesterase activity, Biochem. Pharmacol., 1961, 7, 88–95 CrossRef CAS PubMed.
- S. G. Vega, P. Guzman, L. Garcia, J. Espinosa and C. C. De Nava, Sperm shape abnormality and urine mutagenicity in mice treated with niclosamide, Mutat. Res., 1988, 204, 269–276 CAS.
- WHO, Laboratory manual for the examination of human semen and sperm cervical mucus interaction, Cambridge University Press, Cambridge, United Kingdom, 4th edn, 1999 Search PubMed.
- U. Kvist and L. Bjorndahl, Manual on basic semen analysis. ESHRE Monographs 2, Oxford University Press, Oxford, 2002 Search PubMed.
- S. Tardif, J. P. Laforest, N. Comier and J. L. Bailey, The importance of porcine sperm parameters on fertility in vivo, Theriogenology, 1999, 52, 447–459 CrossRef CAS PubMed.
- WHO, Laboratory manual for the examination of human semen and semen–cervical mucus interaction, University Press, Cambridge, New York, 4th edn, 2001 Search PubMed.
- J. Seed, R. E. Chapin and E. D. Clegg, Methods for assessing sperm motility, morphology, and counts in the rat, rabbit, and dog: a consensus report. ILSI risk science institute expert working group on sperm evaluation, Reprod. Toxicol., 1996, 10, 237–244 CrossRef CAS PubMed.
- R. Filler, Methods for evaluation of rats epididymal sperm morphology, in Male reproductive toxicology, ed. R. E. Chapin and J. H. Heindel, Academic Press Inc., San Diego, CA, 1993, pp. 334–43 Search PubMed.
- K. Narayana, U. J. A. D'Souza and K. P. S. Rao, Ribavirin induced sperm shape abnormalities
in Wistar rat, Mutat. Res., 2002, 513, 193–196 CAS.
- J. M. Lobet, M. T. Colomina and J. J. Sivent, Reproductive toxicology of aluminium in male mice, Fundam. Appl. Toxicol., 1995, 25, 45–51 CrossRef.
- J. A. Buege and S. D. Aust, Microsomal lipid peroxidation, Methods Enzymol., 1978, 52, 302–310 CAS.
- M. L. Hu and C. J. Dillard, Plasma SH and GSH measurement, Methods Enzymol., 1994, 233, 385–387 Search PubMed.
- H. P. Misra and I. Fridovich, The role of superoxide anion in the autoxidation of epinephrine and simple assay for superoxide dismutase, J. Biol. Chem., 1972, 247, 3170–3175 CAS.
- S. Azhar, L. Cao and E. Reaven, Alteration of the adrenal antioxidant defense system during aging in rats, J. Clin. Invest., 1995, 96, 1414–1424 CrossRef CAS PubMed.
- H. Aebi, Catalase in vitro, Methods Enzymol., 1984, 105, 121–126 CAS.
- L. Flohé and W. A. Günzler, Assays of glutathione peroxidase, Methods Enzymol., 1984, 105, 114–121 Search PubMed.
- M. M. Bradford, A rapid and sensitive method for the quantitation of microgram quantities of protein utilizing the principal of protein-dye binding, Anal. Biochem., 1976, 72, 248–254 CrossRef CAS PubMed.
- M. S. Abu-Darwish, C. Cabral, M. J. Gonçalves, C. Cavaleiro, M. T. Cruz, T. Efferth and L. Salgueiro, Artemisia herba-alba essential oil from Buseirah (South Jordan): chemical characterization and assessment of safe antifungal and anti-inflammatory doses, J. Ethnopharmacol., 2015, 174, 153–160 CrossRef CAS PubMed.
- P. Knezevic, V. Aleksic, N. Simin, E. Svircev, A. Petrovic and N. Mimica-Dukic, Antimicrobial activity of Eucalyptus camaldulensis essential oils and their interactions with conventional antimicrobial agents against multi-drug resistant Acinetobacter baumannii, J. Ethnopharmacol., 2016, 178, 125–136 CrossRef CAS PubMed.
- S. Zouari, N. Zouari, N. Fakhfakh, A. Bougatef, M. A. Ayadi and M. Neffati, Chemical composition and biological activities of a new essential oil chemotype of Tunisian Artemisia herba alba Asso, J. Med. Plants Res., 2010, 4, 871–880 CAS.
- M. Younsi, M. Chakroun and A. Nafla, Robust analysis of the determinants of healthcare expenditure growth: evidence from panel data for low-, middle- and high-income countries, Int. J. Health Plann. Manag., 2016 DOI:10.1002/hpm.2358.
- P. Siramon and Y. Ohtani, Antioxidative and antiradical activities of Eucalyptus camaldulensis leaf oils from Thailand, J. Wood Sci., 2007, 53, 498–504 CrossRef CAS.
- E. Giacobini, Do Cholinesterase Inhibitors Have Disease-Modifying Effects in Alzheimer's Disease?, CNS Drugs, 2001, 15(2), 85–91 CrossRef CAS PubMed.
- M. Betancourt, A. Reséndiz and E. C. Fierro, Effect of two insecticides and two herbicides on the porcine sperm motility patterns using computer-assisted semen analysis (CASA) in vitro, Reprod. Toxicol., 2006, 22(3), 508–512 CrossRef CAS PubMed.
- R. Muthuvel, P. Venkataraman, G. Krishnamoorthy, D. N. Gunadharini, P. Kanagaraj, A. Jone Stanley, N. Srinivasan, K. Balasubramanian, M. M. Aruldhas and J. Arunakaran, Antioxidant effect of ascorbic acid on PCB (Aroclor 1254) induced oxidative stress in hypothalamus of albino rats, Clin. Chim. Acta, 2006, 365(1–2), 297–303 CrossRef CAS PubMed.
- A. Basiri, S. Behjati, S. Zand and S. M. Moghaddam, Laparoscopic pyeloplasty in secondary ureteropelvic junction obstruction after failed open surgery, J. Endourol., 2007, 21(9), 1045–1051 CrossRef CAS PubMed.
- E. K. Dirican and Y. Kalender, Dichlorvos-induced testicular toxicity in male rats and the protective role of vitamins C and E, Exp. Toxicol. Pathol., 2012, 64(7–8), 821–830 CrossRef CAS PubMed.
- X. Geng, H. Shao, Z. Zhang, J. C. Ng and C. Peng, Malathion-induced testicular toxicity is associated with spermatogenic apoptosis and alterations in testicular enzymes and hormone levels in male Wistar rats, Environ. Toxicol. Pharmacol., 2015, 39(2), 659–667 CrossRef CAS PubMed.
- S. Selmi, H. Tounsi, I. Safra, A. Abdellaoui, M. R. Rjeibi, S. El-Fazaa and N. Gharbi, Histopathological, biochemical and molecular changes of reproductive function after malathion exposure of prepubertal male mice, RSC Adv., 2015, 5, 13743–13753 RSC.
- I. M. El-Ashmawy, S. Amal and O. M. Salama, Acute and long term safety evaluation of Origanum majorana essential oil, Alexandria J. Pharm. Sci., 2007, 21, 29–35 CAS.
- C. O. Flaherty, E. De Lamirande and C. Gagnon, Positive role of reactive oxygen species in mammalian sperm capacitation: triggering and modulation of phosphorylation events, Free Radicals Biol. Med., 2006, 41, 528–540 CrossRef PubMed.
- P. Syntin and B. Robaire, Sperm structural and motility changes during aging in the brown Norway rat, J. Androl., 2001, 22, 235–244 CAS.
- Y. J. Menezo, A. Hazout, G. Panteix, F. Robert, J. Rollet, P. Cohen-Barcrie, F. Chapuis, P. Clement and M. Benkhalifa, Antioxidants to reduce sperm DNA fragmentation: an unexpected adverse effect, Reprod. BioMed. Online, 2007, 14, 418–421 CrossRef CAS PubMed.
- E. Greco, M. Iacobelli, L. Rienzi, F. Ubaldi, S. Ferrero and J. Tesarik, Reduction of the incidence of sperm DNA fragmentation by oral antioxidant treatment, J. Androl., 2005, 26, 349–353 CrossRef CAS PubMed.
- S. C. Milka and M. Simovi, Antioxidant activity of essential oil and aqueous extract of Pelargonium graveolens L'Her, Food Control, 2012, 23(263), 267 Search PubMed.
- O. Haruyuki and Y. Hideyo, Suppression of neutrophil recruitment in mice by geranium essential oil, Mediators Inflammation, 2004, 13(1), 21–24 CrossRef PubMed.
Footnote |
† Electronic supplementary information (ESI) available. See DOI: 10.1039/c6ra18268b |
|
This journal is © The Royal Society of Chemistry 2016 |