DOI:
10.1039/C6RA17867G
(Paper)
RSC Adv., 2016,
6, 79681-79687
A series of new silver selenites with d0-TM cations†
Received
13th July 2016
, Accepted 15th August 2016
First published on 18th August 2016
Abstract
Systematic explorations of new phases in the Ag+–Ti4+/Zr4+/Nb5+/Ta5+–Se4+–O(F) system by hydrothermal syntheses or standard high temperature solid-state reactions resulted in four new mixed-metal silver selenites, namely, Ag3Ti3O3(SeO3)4F (1, P63), Ag2ZrF2(SeO3)2 (2, Cmca) and AgMO(SeO3)2 (M = Nb, 3; Ta, 4) in the space group Cmcm. Ag3Ti3O3(SeO3)4F features an interesting [Ti3O3(SeO3)4]2− 3D anionic framework composed of 1D chains of corner-sharing TiO6 octahedra which are further interconnected by tridentate bridging SeO32− anions, displaying 1D hexagonal channels of Ti6Se6 12-member rings (MRs) along the c-axis, filled by the Ag+ cations and isolated F− anions. More interestingly, it displays a moderate strong Second-Harmonic Generation (SHG) response about 2 times that of KH2PO4 (KDP). Compound 2 features a novel 1D [ZrF2(SeO3)2]2− anionic chain composed of edge-sharing ZrO4F4 polyhedra in which two neighboring Zr4+ cations are further bridged by a pair of selenite anions. Compounds 3 and 4 are isostructural and their structures feature 1D anionic chains of [MO(SeO3)2]− (M = Nb, Ta) which are separated by Ag+ cations, the 1D [MO(SeO3)2]− (M = Nb, Ta) chain is formed by 1D chains of corner-sharing MO6 (M = Nb, Ta) octahedra in which two neighboring metal centers are also bridged by a pair of selenite anions. Other characterizations including thermal analyses, optical and luminescence property measurements have also been performed.
Introduction
During the last decades, metal selenites and tellurites have attracted a lot of research attention due to their rich structural chemistry and useful physical properties, such as pyroelectricity, ferroelectricity, piezoelectricity and second harmonic generation (SHG). Due to the active lone-pair electrons, Se4+ and Te4+ cations can induce second order Jahn–Teller (SOJT) distortions and the formation of noncentrosymmetric (NCS) or polar structures.1,2 The d0 electronic configuration transition metals (TMs), such as Ti4+, V5+, and Mo6+ are also subject to second order Jahn–Teller (SOJT) distortions.3 Furthermore, the combinations of lone-pair cations with TMs ions with d0 electronic configuration into the same material have been proven to be a very effective synthetic route for new SHG materials due to the additive polarizations from both types of polar unit.4 The d0-TM cations may also display various coordination geometries such as MO4 tetrahedra, MO5 trigonal bipyramids and MO6 octahedra (M = Nb, V, Mo, etc.), these building units can be further interconnected into isolated clusters or extended anionic units, such as [Mo3O14]10− cluster in Ag2Mo3Te3O16, 1D [NbO5]n chain in NaNbO(SeO3)2, 2D [NbO4]n layer in InNb(TeO4)2 and 3D [NbO3]n frameworks in KNbO3, producing a rich structural chemistry.5
So far, a total of fifteen alkali metal selenites and tellurites containing Nb5+ and Ta5+ are reported, including NaNbO(SeO3)2, ATa3Te2O12 (A = K, Rb), ANb3Te2O12 (A = K, Rb), Na2Nb4O7(SeO3)4, Cs3Nb9Te4O32.5b,6 Most of them are centrosymmetric and hence are not SHG-active, except for NaNbO(SeO3)2 which is noncentrosymmetric, its structure features 1D [NbO(SeO3)2]− anionic chains that are separated by Na+ cations, and the material shows a very large SHG response of about 7.8 × KDP.5b Reports on alkaline earth metals selenites and tellurites containing Nb5+ and Ta5+ cations, are very limited, and only Ba2M6Te2O21 (M = Nb, Ta) have been reported.7 Its structure features a 3D [M6O21]n framework of corner-sharing MO6 octahedra that are decorated by TeO32− anions.7
Five rare earth metal selenites and tellurites containing Nb5+ and Ta5+ are reported, including La4M2Te6O23 (M = Nb, Ta), YNbM2O8 (M = Se, Te), and LaNbTeO6.8 It's worth mentioning that the structural of YNbTe2O8 is composed of 2D [NbO5]n layers of corner-sharing NbO6 octahedra and [YO4]n layers of edged-sharing YO8 polyhedra which are further interconnected by bridging TeO32− anions to be a complex 3D framework.8
In addition, eight post-main group metal selenites and tellurites containing Nb5+ and Ta5+ have been reported, including Pb2NbO2(SeO3)2Cl, InNb(TeO4)2, Pb4Te6M10O41 (M = Nb, Ta), and BiNbTe2O8.5c,9 They display a number of interesting anionic structures, such as 1D [NbO2(SeO3)2]3− anionic chains in Pb2NbO2(SeO3)2Cl, 2D [NbO2(TeO3)2]3− anionic layers in BiNbTe2O8 and 3D [M6O23(TeO3)6]8− frameworks in Pb4Te6M10O41 (M = Nb, Ta). More interestingly, InNb(TeO4)2 displays a moderate strong SHG response of 100× α-SiO2.9
Moreover, a few metal selenites and tellurites containing Ti4+ and Zr4+ are reported, including Ti(SeO3)2, Ti(Te3O8), Zr(Te3O8) TiF2(TeO3), Zr2Te4O11X2 (X = Cl, Br), Pb2TiOF(SeO3)2Cl, Cs(TiOF)3(SeO3)2, etc.6a,9a,10 Among these compounds, only Pb2TiOF(SeO3)2Cl and Cs(TiOF)3(SeO3)2 are NCS, displaying large strong SHG responses of about 5 × KDP and 9.6 × KDP, respectively.6a,9a The structure of Pb2TiOF(SeO3)2Cl exhibits a 3D framework composed of 1D [TiOF(SeO3)2]3− anionic chains and 1D (Pb2Cl)3+ cationic chains, whereas the structure of Cs(TiOF)3(SeO3)2 features an hexagonal tungsten oxide (HTO) layered topology consisting of a 2D [(TiOF)3(SeO3)2]− anionic layer.
The Ag+ cation has similar ionic radius with of those of Na+ and K+,11c it is able to adopt several types of coordinate geometries, the silver compounds can also display interesting luminescent properties.5a,11,17 To the best of our knowledge, no compound in Ag+–M (Ti4+, Zr4+, Nb5+, Ta5+)–Se4+/Te4+ systems has been reported. Our explorations of new phases in these systems led to the isolation of four new mixed-metal silver(I) selenites, namely, Ag3Ti3O3(SeO3)4F (1), Ag2ZrF2(SeO3)2 (2), AgNbO(SeO3)2 (3) and AgTa(SeO3)2 (4). Interestingly, Ag3Ti3O3(SeO3)4F is polar and exhibits a moderate strong SHG signal of about 2 × KDP. Herein we their syntheses, crystal structures, thermal stabilities and optical properties.
Experimental section
Materials and methods
AgNO3 (99.5+%, AR), TiO2 (99.5+%, AR), ZrO2 (99.5+%, AR), Nb2O5 (99.5+%, AR), Ta2O5 (99.5+%, AR), SeO2 (99%, AR) and hydrofluoric acid (40+%, AR) were used as received. All of the chemicals were supplied by the Shanghai Reagent Factory. The UV-Vis-NIR diffuse reflectance spectra were collected on a PE Lambda 900 UV-vis-NIR spectrophotometer in the range 190–2500 nm at room temperature. BaSO4 plates were used for a standard (100% reflectance) for the baseline correction. The absorption spectrum was converted from reflectance spectra using the Kubelka–Munk function: α/S = (1 − R)2/2R = K/S,12 where α, S, R, and K represent the absorption coefficient, scattering coefficient, reflectance, and absorption, respectively. Infrared spectra were recorded on a Magna 750 FT-IR spectrometer as KBr pellets in the range of 4000–400 cm−1 at room temperature. Differential scanning calorimetry (DSC) and thermogravimetric analysis (TGA) were carried out on a Netzch STA449C and heated at a rate of 10 °C min−1 to 800 °C under a N2 atmosphere. Powder SHG effect for 1 was measured by using the modified Kurtz- and Perry's method by using a pulsed Nd:YAG laser with a wavelength of 1064 nm.13 The samples were ground and sieved into a particle size of 75–105 μm. KDP powder Sieved in the same particle size was used as a reference material to assume the SHG effect.
Synthesis of 1 and 2
Compounds 1 and 2 were synthesized hydrothermally within Teflon-lined steel autoclaves (23 ml). The loaded starting materials are AgNO3 (110 mg, 0.65 mmol), TiO2 (40 mg, 0.5 mmol), SeO2 (220 mg, 2 mmol), a solution of ∼40% HF (0.3 ml, ∼6 mmol) and 3 ml of distilled water for Ag3Ti3O3(SeO3)4F, and AgNO3 (110 mg, 0.65 mmol), ZrO2 (60 mg, 0.5 mmol), SeO2 (166 mg 1.5 mmol), a solution of ∼40% HF (0.3 ml, ∼6 mmol) and 10 ml of distilled water for Ag2ZrF2(SeO3)2. The autoclaves were heated at 220 °C for 4 days, and cooled slowly to room temperature at 1.5 °C h−1. The crystalline products were washed with distilled water and dried in air. The yields are 76% (based on TiO2) and 52% (based on ZrO2) for 1 and 2 respectively. Microprobe elemental analyses on two crystals of each compound gave average molar ratios of Ag/M/Se/F (M = Ti or Zr) to be 2.9
:
3.0
:
3.9
:
1.1 and 2.2
:
0.9
:
1.9
:
2.3 for Ag3Ti3O3(SeO3)4F and Ag2ZrF2(SeO3)2 respectively, which are closed to those determined from single-crystal X-ray structural studies. The measured powder XRD patterns of the two compounds are in good agreement with the simulate ones from their single-crystal data (Fig. S1a and b†). IR data (KBr, cm−1): 430(s), 456(s), 506(s), 545(s), 673(s), 729(s), 858(m), 918(m) for 1, 410(s), 483(s), 516(s), 668(s), 712(s), 818(m) for 2 (Fig. S3a and b†).
Synthesis of 3 and 4
Compounds 3 and 4 were synthesized through standard high temperature solid-state reactions of Ag2O, Nb2O5 (or Ta2O5) and SeO2. The loaded compositions are as follows: Ag2O (0.070 g, 0.3 mmol), Nb2O5 (0.079 g, 0.3 mmol) (or Ta2O5 0.133 g, 0.3 mmol for 4) and SeO2 (0.222 g, 2 mmol). These mixtures were ground intimately and pressed into pellets. The reaction mixtures were introduced into quartz tubes that were subsequently evacuated. The quartz tubes containing the reaction mixtures were heated at 720 °C (for 3) or 750 °C (for 4) for 7 days and then cooled slowly to 200 °C at 5 °C h−1 before switching off the furnace. Colorless crystals of 3 and 4 were obtained in the yields of 56% and 64% (based on M2O5), respectively. Microprobe elemental analyses on two crystals of each compound gave average molar ratios of Ag/M/Se (M = Nb or Ta) of 0.9
:
0.9
:
2.3 and 0.8
:
0.9
:
2.0 for 3 and 4, respectively, which are close to those determined from single-crystal X-ray structural analyses. The measured powder XRD patterns for the two compounds are in good agreement with the ones simulated from their single-crystal data (Fig. S1c and d†). IR data (KBr, cm−1): 449(s), 581(s), 690(s), 844(m), 874(m) for 3; 448(s), 531(s), 610(s), 735(s), 845(m), 876(m) for 4 (Fig. S3c and d†).
Single-crystal structure determination
Single crystals of 1–4 were mounted on SuperNova (Mo) X-ray Source, Mo-Kα radiation (λ = 0.71073 Å) at room temperature. The data sets were corrected for Lorentz and polarization factors as well as for empirical absorption by the multi-scan method.14a All of four structures were solved by direct methods and refined by a full-matrix least-squares fitting on F2 by SHELX-97.14b The refined Flack factor of 0.0268 for compound 1 is close to zero, confirming the correctness of its absolute structure. All of four structures were also checked for possible missing symmetry with the program PLATON and none was found.14c Crystallographic data, structural refinements are summarized in Table 1 and important bond distances are listed in Table 2. More details of the crystallographic studies are given in the ESI.†
Table 1 Summary of crystal data and structural refinements for compounds 1–4
R1 = ∑||Fo| − |Fc||/∑|Fo|, wR2 = {∑w[(Fo)2 − (Fc)2]2/∑w[(Fo)2]2}1/2. |
Formula |
Ag3Ti3O3(SeO3)4F |
Ag2ZrF2(SeO3)2 |
AgNbO(SeO3)2 |
AgTaO(SeO3)2 (4) |
Fw |
1042.15 |
598.88 |
470.70 |
558.74 |
Space group |
P63 |
Cmca |
Cmcm |
Cmcm |
a (Å) |
12.8975(4) |
7.3640(5) |
10.813(5) |
10.7921(11) |
b (Å) |
12.8975(4) |
31.451(2) |
7.792(4) |
7.8283(7) |
c (Å) |
5.2180(2) |
6.2719(5) |
7.363(4) |
7.2717(6) |
V (Å3) |
751.70(4) |
1452.62(19) |
620.4(5) |
614.34(10) |
Z |
2 |
8 |
4 |
4 |
Dc (g cm−3) |
4.604 |
5.477 |
5.040 |
6.041 |
μ (mm−1) |
15.123 |
16.802 |
16.724 |
32.821 |
GOF on F2 |
1.078 |
1.097 |
1.139 |
1.132 |
Flack factor |
0.0268 |
None |
None |
None |
R1, wR2 (I > 2(σ(I))a |
0.0360, 0.0999 |
0.0237, 0.0433 |
0.0283, 0.0694 |
R1 = 0.0292, wR2 = 0.0689 |
R1, wR2 (all data)a |
0.0366, 0.1004 |
0.0335, 0.0477 |
0.0290, 0.0698 |
R1 = 0.0340, wR2 = 0.0727 |
Table 2 Selected bond distances (Å) for compounds 1–4a
Symmetry transformations used to generate equivalent atoms: for 1: #1 x − y, x, z − 1/2; #2 x − y, x, z + 1/2; #3 −x + 1, −y, z − 1/2; #4 y, −x + y, z + 1/2; #5 y, −x + y, z − 1/2; #6 −y, x − y − 1, z; #7 −x + y + 1, −x, z. For 2: #1 −x + 1/2, −y + 1/2, −z; #2 −x + 1, −y + 1/2, z + 1/2; #3 −x + 1/2, y, −z + 1/2; #4 x, −y + 1, −z; #5 x + 1/2, y, −z − 1/2; #6 −x + 1/2, −y + 1, z + 1/2. #7 x − 1/2, y, −z + 1/2; #8 x + 1/2, y, −z + 1/2; #9 −x + 1, y, z; #10 −x, y, z. For 3 and 4: #1 x, −y + 1, −z; #2 −x, y, z; #3 −x, −y + 1, −z; #4 −x, −y, −z + 1; #5 x, −y, −z + 1; #6 x, y, −z + 1/2. |
Ag3Ti3O3(SeO3)4F |
Ag(1)–O(1)#1 |
2.411(7) |
Ti(1)–O(3) |
1.956(7) |
Se(1)–O(2) |
1.716(7) |
Ag(1)–O(2) |
2.460(7) |
Ti(1)–O(4) |
1.976(8) |
Se(1)–O(3) |
1.731(7) |
Ag(1)–O(3)#2 |
2.489(7) |
Ti(1)–O(2)#4 |
2.043(7) |
Se(2)–O(4) |
1.697(7) |
Ti(1)–O(5) |
1.769(7) |
Ti(1)–O(1)#5 |
2.169(7) |
Se(2)–O(4)#6 |
1.697(7) |
Ti(1)–O(5)#3 |
1.908(8) |
Se(1)–O(1) |
1.695(7) |
Se(2)–O(4)#7 |
1.697(7) |
![[thin space (1/6-em)]](https://www.rsc.org/images/entities/char_2009.gif) |
Ag2ZrF2(SeO3)2 |
Ag(1)–O(1)#1 |
2.357(3) |
Zr(1)–O(3)#3 |
2.128(3) |
Se(1)–O(1) |
1.678(5) |
Ag(1)–O(1)#2 |
2.357(3) |
Zr(1)–O(3) |
2.128(3) |
Se(1)–O(2)#9 |
1.702(3) |
Ag(1)–O(2)#3 |
2.524(4) |
Zr(1)–O(2)#3 |
2.142(3) |
Se(1)–O(2) |
1.702(3) |
Ag(1)–O(2) |
2.524(4) |
Zr(1)–O(2) |
2.142(3) |
Se(2)–O(4) |
1.662(5) |
Ag(2)–O(3) |
2.456(3) |
Zr(1)–F(2) |
2.172(2) |
Se(2)–O(3) |
1.713(3) |
Ag(2)–O(3)#4 |
2.456(3) |
Zr(1)–F(2)#7 |
2.172(2) |
Se(2)–O(3)#10 |
1.713(3) |
Ag(2)–O(4)#5 |
2.457(3) |
Zr(1)–F(1) |
2.188(2) |
|
|
Ag(2)–O(4)#6 |
2.457(3) |
Zr(1)–F(1)#8 |
2.188(2) |
|
|
![[thin space (1/6-em)]](https://www.rsc.org/images/entities/char_2009.gif) |
AgNbO(SeO3)2 |
Ag(1)–O(1)#1 |
2.455(4) |
Nb(1)–O(3) |
1.963(2) |
Nb(1)–O(2) |
1.984(3) |
Ag(1)–O(1)#2 |
2.455(3) |
Nb(1)–O(3)#4 |
1.963(2) |
Nb(1)–O(2)#5 |
1.984(3) |
Ag(1)–O(1)#3 |
2.455(4) |
Nb(1)–O(2)#1 |
1.984(3) |
Se(1)–O(1) |
1.656(5) |
Ag(1)–O(1) |
2.455(3) |
Nb(1)–O(2)#4 |
1.984(3) |
Se(1)–O(2) |
1.742(3) |
|
|
|
|
Se(1)–O(2)#6 |
1.742(3) |
![[thin space (1/6-em)]](https://www.rsc.org/images/entities/char_2009.gif) |
AgTaO(SeO3)2 |
Ag(1)–O(1)#1 |
2.453(6) |
Ta(1)–O(3) |
1.931(4) |
Ta(1)–O(2) |
1.992(5) |
Ag(1)–O(1)#2 |
2.453(6) |
Ta(1)–O(3)#4 |
1.931(4) |
Ta(1)–O(2)#5 |
1.992(5) |
Ag(1)–O(1)#3 |
2.453(6) |
Ta(1)–O(2)#1 |
1.992(5) |
Se(1)–O(1) |
1.643(8) |
Ag(1)–O(1) |
2.453(6) |
Ta(1)–O(2)#4 |
1.992(5) |
Se(1)–O(2) |
1.737(6) |
|
|
|
|
Se(1)–O(2)#6 |
1.737(6) |
Result and discussion
Crystal structures of Ag3Ti3O3(SeO3)4F (1)
Ag3Ti3O3(SeO3)4F (1) crystallizes in a polar space group P63, and its structure features a complex 3D framework composed of 1D chains of corner-sharing TiO6 octaherda interconnected by tridentately bridging selenite groups, forming 1D tunnels of 12 membered rings (MRs) along the c-axis, which are filled by “isolated” Ag+ cations and F− anions (Fig. 1a). The asymmetric unit of Ag3Ti3O3(SeO3)4F contains one Ag+, one Ti4+, one F−, two Se and five oxygen atoms. One of the Se atom and F atom are in sites on threefold rotation axis whereas all of the remaining atoms occupy the general sites. Ti4+ cations octahedrally coordinated by four selenite oxygen atoms and two oxo anions. The six Ti–O bonds are one short (1.769(7) Å), four normal (1.909(8)–2.043(7) Å) and one long (2.169(7) Å), hence the octahedron distorted toward a corner (local C4 direction). The magnitude of distortion (Δd) is calculated to be 0.58,15 which is close to that of the TiO5F octahedron in Pb2TiOF(SeO3)2Cl.7 Both Se4+ atoms are in a distorted ψ-SeO3 tetrahedral geometry in which one apex is occupied by the lone pair. The Se(1)–O bond lengths range from 1.695(7) Å to 1.731(7) Å, whereas the three Se(2)–O bond lengths are equal (1.697(7) Å).
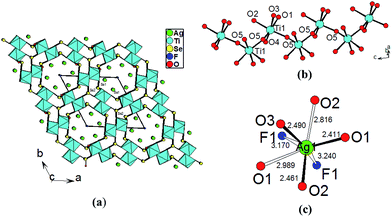 |
| Fig. 1 View of the structure of Ag3Ti3O3(SeO3)4F along the c axis (a), a 1D chain of corner-sharing TiO6 octaherda along the c-axis (b), and the coordination geometry around the silver(I) ion (c). TiO6 octahedra are shaded in cyan. Ag, Ti, Se, O and F atoms are drawn as green, cyan, yellow, red and blue circles, respectively. | |
The TiO6 octahedra are interconnected by corner-sharing oxo (O5) anions into a 1D chain along the c-axis (Fig. 1b). These 1D titanium oxide chains are further bridged by selenite anions in tridentate fashion into a [Ti3O3(SeO3)4]2− 3D anionic frameworks with 1D Ti6Se6 12-member rings (MRs) composed of six TiO6 octaherda and six SeO3 groups (Fig. 1a). The size of the channel is estimated to be 5.853 × 5.853 Å based on the structural data. The “isolated” Ag+ cations and F− anions are located at above tunnels. The Ag+ cation is coordinated by three Se(1)O3 groups in a unidentate fashion into a AgO3 trigonal pyramidal geometry with Ag atom lying on the pyramidal site, and the Ag–O bond distances range from 2.411(7) to 2.489(7) Å (Table 2), which are comparable to those in other related compounds.5a,17 Bond valence calculations gave total bond valence (BVS) values of 4.0, 3.9, 4.0 and 0.63 for Ti(1), Se(1), Se(2) and Ag+ respectively,16 revealing their oxidation states of 4+, 4+, 4+, and 1+. The large deviation of Ag+ from +1 indicates that three exist a few weak Ag–O (2.820(2) Å, 2.972(3) Å) and Ag–F bonds (3.160(3) Å, 3.220(3) Å) (Fig. 1c) in its secondary coordination sphere (Fig. 1c).
Unlike those in 1, the F− anion in both Pb2TiOF(SeO3)2Cl is involved in the coordination with the Ti4+ cation.6a It is also worth noting that selenite anions in the [TiO(SeO3)3]4− anionic chain of Pb2TiOF(SeO3)2Cl are unidentate or bidentate bridging regarding to the Ti4+ cations, whereas each selenite anion in Ag3Ti3O3(SeO3)4F connects with three titanium.
Structure of Ag2ZrF2(SeO3)2 (2)
The structure of compound 2 features 1D ZrF2(SeO3)22− anionic chains which are separated by Ag+ cations (Fig. 2a). Compound 2 crystallized in centrosymmetric space group Cmca, its asymmetric unit contains two Ag, one Zr, two Se, two F and four oxygen atoms. Ag(1), Ag(2) and Zr(1) atoms lie on two fold rotation axes whereas Se(1), Se(2), F(1), F(2), O(1) and O(4) atoms sit on a mirror plane, only O(2) and O(3) occupy the general sites. The Zr4+ cation is eight-coordinated by four oxygen atoms from four different selenite anions and four F− anions. The Zr–O bond distances are in the range of 2.128(3)–2.142(3) Å whereas Zr–F bonds fall in the range of 2.172(2)–2.188(2) Å, these bond distances are comparable to those reported in other related compounds.10 Both Se4+ cations are in the asymmetric coordination environment and adopt ψ-SeO3 trigonal pyramidal geometry with the pyramidal site occupied by the lone pair electrons. The Se–O lengths are in the range of 1.662(5)–1.713(3) Å.
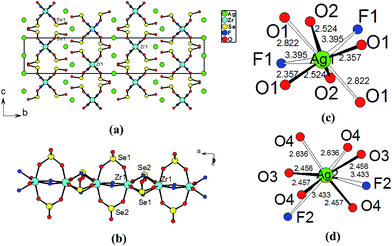 |
| Fig. 2 View of the structure of Ag2ZrF2(SeO3)2 along the a-axis (a), a 1D [ZrF2(SeO3)2]2− anionic chain along a axis (b), and coordination environments around silver(I) ions (c and d). Ag, Zr, Se, O and F atoms are drawn as green, cyan, yellow, red and blue circles, respectively. | |
Neighboring ZrO4F4 polyhedra are interconnected via edge-sharing (F(1)⋯F(2)) into a 1D chain along the a-axis, within the chain each pair of Zr4+ cations are further bridged by a pair of SeO3 groups, resulting in the formation of a 1D ZrF2(SeO3)22− anionic chain (Fig. 2b). It is interesting to note that within the 1D anionic chain, the neighboring Ag2F2 four member rings or Ag2(SeO3)2 six member rings are almost perpendicular each other.
The Ag+ cations are located in-between above 1D anionic chains, keeping charge balance. Both Ag+ cations are four-coordinated by four selenite oxygen atoms with the Ag–O bond distances ranging from 2.357(3) to 2.524(4) Å for Ag(1) and 2.456(3) to 2.457(3) for Ag(2), which are comparable to those reported in other related compounds.5a,17 The calculated total bond valences for Ag(1), Ag(2), Zr, Se(1) and Se(2) are 0.74, 0.69, 4.0, 4.1 and 4.1,16 revealing the oxidation states of +1, +1, +4, +4, +4, respectively. The large deviation of BVS of Ag(1) and Ag(2) from +1 indicates that some elongated Ag–F bonds and Ag–O bonds should be considered as secondary coordination bonds (Ag–F = 3.3395(3)–3.433(3) Å; Ag–O = 2.636(3)–2.821(5) Å) (Fig. 2c and d).
It is worth to compare the structure of 2 with those of the [Zr2Te4O11X2] (X = Cl, Br).10a The structure of [Zr2Te4O11X2] (X = Cl, Br) consists of a [Zr2Te4O11]2+ cationic layer with halide anions as inter-lamellar charge-balancing anions. The [Zr2Te4O11]2+ cationic layer is composed of {Zr2O11}n ribbons of edge-sharing or corner-sharing ZrO7 pentagonal bipyramids that are further bridged by two different tellurium(IV) oxide anions: TeO32− and Te3O84−.10a
Structure of AgMO(SeO3)2 (M = Nb, 3, Ta, 4)
Compounds 3 and 4 are isostructural and crystallized in the centrosymmetric space group Cmcm. Only the structure of compound 3 will be described in detail. The structure of compound 3 features 1D [NbO(SeO3)2]− anionic chains that are separated by Ag+ cations (Fig. 3a). The asymmetric unit of 3 contains one Ag, one Nb and one Se and three O atoms. The Nb5+ cation with a site symmetry of 2/m is octahedrally coordinated by four oxygens from four selenite anions with four equal Nb–O bond distance of 1.984(3) Å and two bridging oxo anions with equal Nb–O bond distance of 1.963(2) Å, these bond distances are comparable to those reported in other related compounds.5a,17 Se4+ lying on a mirror plane is in a ψ-SeO3 trigonal pyramidal environment with the pyramidal site occupied by the lone pair of electrons. The Se–O bond lengths range from 1.656(5) to 1.742(3) Å.
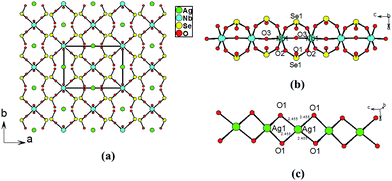 |
| Fig. 3 View of the structure of AgNbO(SeO3)2 along the c-axis (a), a 1D [NbO(SeO3)2]− chain along the c axis (b), and a 1D silver oxide chain based on AgO4 squares (c). Ag, Nb, Se and O atoms are drawn as green, cyan, yellow and red circles, respectively. | |
Neighboring NbO6 octahedra are interconnected via corner-sharing (O(3)) into a 1D niobium oxide chain along the c-axis. Within the chain, each pair of Nb5+ cations are further bridged by a pair of selenite groups, resulted in the formation of 1D [NbO(SeO3)2]− anionic chain along the c-axis (Fig. 3b). Neighboring 1D [NbO(SeO3)2]− anionic chains are separated by Ag+ cations (Fig. 3b). The Ag+ cation lying on a site with 2/m symmetry is four coordinated by four selenite oxygen atoms to form a perfect planar square. The Ag–O bond lengths are 2.455(3) Å. These AgO4 square units are edge-sharing into a 1D silver oxide chain along the c axis (Fig. 3c). The calculated total bond valences for Ag, Nb and Se are 0.70, 5.1 and 4.1,16 respectively, confirming their oxidation states of +1, +5 and +4, respectively. The large deviation of Ag+ from +1 indicates that a few elongated Ag–O bonds need be considered as secondary coordination bonds (Ag–O = 2.980(3) Å).
It is interesting to note that the structure of polar NaNbO(SeO3)2.5b Both compounds feature similar 1D [NbO(SeO3)2]− anionic chains that are separated by sodium(I) or silver(I) cations. Due to the different cationic sizes for Na+ and Ag+, the two structures also exhibit a few differences. The NbO6 octahedron in the silver compound is non-distorted whereas that in sodium compound is distorted toward a corner with the magnitude of the distortion (Δd) of 0.34.15 Furthermore, NaNbO(SeO3)2 is polar (Cmc21) and exhibits a very large SHG response of 7.8 times of KDP whereas AgNbO(SeO3)2 is structurally centric and not SHG active. Hence the size of counter-cation also has a dramatic effect on whether or not the structure of the material formed is centric or acentric. The similar phenomena are observed in ASc(SeO3)2 (A = Na, K, Rb, and Cs) system.10e However, the structure of another unpolar isomeric NaNbO(SeO3)2 exhibits a similar 1D [NbO(SeO3)2]− anionic chains that are separated by sodium(I). The reason may be that it was got at a lower reaction temperature.6d
TGA and DSC studies
Thermogravimetric analysis (TGA) has been used to investigate the thermal behavior of the three materials. Compounds 1 and 2 are stable up to 430 and 343 °C, respectively. Their DSC curves exhibit one main endothermal peaks at around 465 °C and 456 °C, respectively (Fig. S2a and b†). The total weight losses of 46.22% and 43.22% for compounds 1 and 2 correspond to the release of the fluorine and SeO2 molecules. It is in good agreement with calculated values (45.95% for 1, 43.22% for 2). The final residuals were not characterized due to their melting with the TGA bucket made of Al2O3 under such high temperature.
Both compounds 3 and 4 present one main step of weight loss in the temperature ranges of 453–800 °C and 497–673 °C, respectively. Their DSC curves exhibit one main endothermal peak at around 484 °C or 569 °C, respectively (Fig. S2c and d†). The observed total weight losses of 46.05% and 39.72% for compounds 3 and 4 correspond to the release of all selenium in the form of SeO2. They are in good agreement with calculated values (47.15% for 3, 38.85% for 4). The final residuals were not characterized due to their melting with the TGA bucket made of Al2O3 under such high temperature.
IR and UV spectral measurements
The IR spectra of four compounds were measured in 4000–400 cm−1 at 298 K (Fig. S3†). All four compounds show no absorption bands in the region 4000–1000 cm−1. They show a few absorption bands in the range of 400–1000 cm−1 related to Zr–O(F), Ti–O, Nb–O, Ta–O, Se–O, and Se–O–Se vibrations (Fig. S3†).5b,6a,9a The absorption bands from 840–950 cm−1 belong to the Ta–O, Nb–O and Ti–O vibrations, whereas the band at 818 cm−1 belongs to the Zr–O/F vibrations, which is similar to those in Cs(TaO2)3(SeO3)2, Cs(TiOF)3(SeO3)2 and NaNbO(SeO3)2.5b,6a Moreover, the bands at 400–700 cm−1 belong to ν(Se–O–Se) and ν(Se–O).5b,6a,9a
UV-vis-NIR absorption spectral studies of four compounds show no absorption in the wavelength range of 410–2000, 397–2000, 397–2000 and 349–2000 nm, respectively for compounds 1–4 (Fig. S4†). Results of the diffuse reflectance spectra reveal wide band gaps of about 3.02, 3.12, 3.12 and 3.55 eV for compounds 1–4, respectively, which is close to those for Cs(TaO2)3(SeO3)2, Pb2TiOF(SeO3)2Cl and NaNbO(SeO3)2,5b,6a,9a indicating that all of them are wide band gap materials (Fig. S4†).
Luminescent properties
The solid state luminescent properties of compounds 1–4 have been investigated at room temperature, and they show similar luminescent features. Under excitation at 280 nm, each compound displays two strong emission bands and a shoulder band. The strong emission bands appeared at 420 nm and 483 nm, whereas the shoulder band occurs at 458 nm (Fig. 4). Their luminescent properties are comparable to those of other silver compounds reported.5a,17 These emission bands may be associated with Ag+ ions and can be assigned to the metal-centered d → s transitions as well as the metal-anion interactions.5a,17 The lifetime of the luminescence is too shorter to be measured by our instrument.
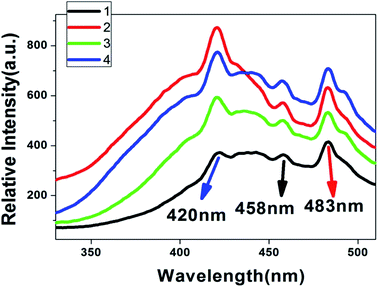 |
| Fig. 4 Emission spectra of 1 (black), 2 (red), 3 (blue), and 4 (green) under λex = 280 nm. | |
Second harmonic generation (SHG) measurements
Compound 1 is acentric with a polar space group of P63. Hence, it is worth to study its second-order NLO property. SHG measurements on a Q-switched Nd:YAG laser on the sieved-powder sample (75–105 μm) revealed that it displays a moderate strong SHG response of about 2 × KDP (Fig. 5) which is weaker than those of other two compounds Cs(TiOF)3(SeO3)2 and Pb2TiOF(SeO3)2Cl.6a,9a Unfortunately, the particle size of compound 1 is not large enough to perform phase-matching measurements. Such SHG response can be attributed to the additive polarizations of both the lone pairs of the selenites anions and the distorted TiO6 octahedra. The dipole moments for the unit cells of the compounds have been calculated.18 The dipole moments of asymmetric building units TiO6, Se(1)O3 and Se(2)O3 polyhedra are 4.073, 9.668 and 9.373 D, respectively (Table. S1†), which are close to the values reported previously.6a The dipole moments along x- and y-axes canceled out completely. Along the polar z-axis, the dipole moments for all polar units are constructively added in the unit cell to a value of 27.337 D. That is the reason why compound 1 displays a weaker SHG response than those of other two compounds Cs(TiOF)3(SeO3)2 and Pb2TiOF(SeO3)2Cl.6a,9a
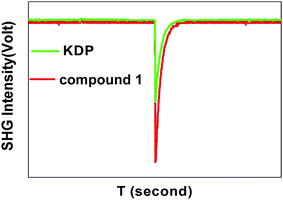 |
| Fig. 5 Oscilloscope traces of the SHG signals for the powders (75–105 μm) for KDP and Ag3Ti3O3(SeO3)4F on a 1064 nm Q-switched Nd:YAG laser. | |
Conclusions
Systematic explorations of new phases in Ag+–M (Ti4+, Zr4+, Nb5+, Ta5+)–Se4+ systems resulted in four new compounds, namely, Ag3Ti3O3(SeO3)4F (1), Ag2ZrF2(SeO3)2 (2), AgNbO(SeO3)2 (3) and AgTaO(SeO3)2 (4). Compound 1 features an interesting 3D [Ti3O3(SeO3)4]2− anionic framework with 1D large tunnels of 12-MRs channels filled by Ag+ cations and isolated F− anions. The Structures of compounds 2–4 consist of 1D [ZrF2(SeO3)2]2− or [MO(SeO3)2]− (M = Nb, Ta) anionic chains which are separated by silver(I) ions. More interestingly, compound 1 displays a moderate strong SHG response of 2 times of KDP. It is believed that other new second-order NLO materials may be discovered in Ag-d0 TM-tellurite systems and we are currently exploring these possibilities.
Acknowledgements
We thank National Natural Science Foundation of China (Grant No. 21231006, 21203197 and 91222108) for the financial support.
Notes and references
-
(a) F. Kong, S. P. Huang, Z. M. Sun, J. G. Mao and W. D. Cheng, J. Am. Chem. Soc., 2006, 128, 7750–7751 CrossRef CAS PubMed;
(b) L. Geng, Q. Li, C. Y. Meng, K. Dai, H. Y. Lu, C. S. Lin and W. D. Cheng, J. Mater. Chem. C, 2015, 3, 12290–12296 RSC;
(c) S. Pan, J. P. Smit, B. Watkins, M. R. Marvel, C. L. Stern and K. R. Poeppelmeier, J. Am. Chem. Soc., 2006, 128, 11631–11634 CrossRef CAS PubMed;
(d) X. L. Cao, F. Kong, C. L. Hu, X. Xu and J. G. Mao, Inorg. Chem., 2014, 53, 8816–8824 CrossRef CAS PubMed.
-
(a) H.-S. Ra, K. M. Ok and P. S. Halasyamani, J. Am. Chem. Soc., 2003, 125, 7764–7765 CrossRef CAS PubMed;
(b) S. H. Kim, J. Yeon and P. S. Halasyamani, Chem. Mater., 2009, 21, 5335–5342 CrossRef CAS;
(c) S. Y. Zhang, C. L. Hu, C. F. Sun and J. G. Mao, Inorg. Chem., 2010, 49, 11627–11636 CrossRef CAS PubMed;
(d) D. Phanon and I. Gautier-Luneau, Angew. Chem., Int. Ed., 2007, 46, 8488–8491 CrossRef CAS PubMed.
-
(a) P. S. Halasyamani, Chem. Mater., 2004, 16, 3586–3592 CrossRef CAS;
(b) M. R. Marvel, J. Lesage, J. Baek, P. S. Halasyamani, L. C. Stern and K. R. Poeppelmeier, J. Am. Chem. Soc., 2007, 129, 13963–13969 CrossRef CAS PubMed;
(c) H. Y. Chang, S. H. Kim, K. M. Ok and P. S. Halasyamani, Chem. Mater., 2009, 21, 1654–1662 CrossRef CAS.
-
(a) K. M. Ok, E. O. Chi and P. S. Halasyamani, Chem. Soc. Rev., 2006, 35, 710–717 RSC;
(b) S. Y. Song and K. M. Ok, Inorg. Chem., 2015, 54, 5032–5038 CrossRef CAS PubMed;
(c) J. J. Zhang, Z. H. Zhang, Y. X. Sun, C. Q. Zhang, S. J. Zhang, Y. Liu and X. T. Tao, J. Mater. Chem., 2012, 22, 9921–9927 RSC;
(d) S.-J. Oh, D. W. Lee and K. M. Ok, Inorg. Chem., 2012, 51, 5393–5399 CrossRef CAS PubMed.
-
(a) Y. Zhou, C. L. Hu, T. Hu, F. Kong and J. G. Mao, Dalton Trans., 2009, 5747–5754 RSC;
(b) X. L. Cao, C. L. Hu, F. Kong and J. G. Mao, Inorg. Chem., 2015, 54, 10978–10984 CrossRef CAS PubMed;
(c) Y. H. Kim, D. W. Lee and K. M. Ok, Inorg. Chem., 2014, 53, 5240–5245 CrossRef CAS PubMed;
(d) A. W. Hewat, J. Phys. C: Solid State Phys., 1973, 6, 2559–2572 CrossRef CAS.
-
(a) X. L. Cao, C. L. Hu, F. Kong and J. G. Mao, Inorg. Chem., 2015, 54, 3875–3882 CrossRef CAS PubMed;
(b) Q. H. Gu, C. L. Hu, J. H Zhang and J. G. Mao, Dalton Trans., 2011, 40, 2562–2569 RSC;
(c) K. M. Ok and P. S. Halasyamani, Inorg. Chem., 2005, 44, 3919–3925 CrossRef CAS PubMed;
(d) Y. K. Kim, B. S. Kim and K. M. Ok, J. Alloys Compd., 2016, 662, 381–387 CrossRef CAS.
- B. H. Mueller and B. Wedel, Z. Naturforsch., B: J. Chem. Sci., 1996, 51, 1411–1414 Search PubMed.
-
(a) Y. K. Kim, B. G. Kim, T. S. You and K. M. Ok, J. Alloys Compd., 2015, 637, 155–161 CrossRef CAS;
(b) K. M. Ok, L. Zhang and P. S. Halasyamani, J. Solid State Chem., 2003, 175, 264–271 CrossRef CAS.
-
(a) X. L. Cao, C. L. Hu, X. Xu, F. Kong and J. G. Mao, Chem. Commun., 2013, 49, 9965–9967 RSC;
(b) K. M. Ok and P. S. Halasyamani, Inorg. Chem., 2004, 43, 4248–4253 CrossRef CAS PubMed;
(c) S. Garcia-Martin, M. L. Veiga, A. Jerez, C. Pico, J. Santamaria, J. G. E. Gonzalez-Diaz and E. Iborra, Solid State Ionics, 1989, 37, 87–93 CrossRef CAS;
(d) S. Blanchandin, J. C. Champarnaud-Mesjard, P. Thomas and B. Frit, J. Alloys Compd., 2000, 306, 175–185 CrossRef CAS;
(e) P. X. Li, F. Kong, C. L. Hu, N. Zhao and J. G. Mao, Inorg. Chem., 2010, 49, 5943–5952 CrossRef CAS PubMed;
(f) I. Yakine, M. Naji, A. Chagraoui, A. Moussaoui and A. Tairi, Mater. Chem. Phys., 2012, 135, 241–248 CrossRef CAS.
-
(a) J. Lin, J. N. Cross, J. Diwu, M. J. Polinski, E. M. Villa and T. E. Albrecht-Schmitt, Inorg. Chem., 2012, 51, 11949–11954 CrossRef CAS PubMed;
(b) G. Meunier and J. Galy, Acta Crystallogr., Sect. B: Struct. Crystallogr. Cryst. Chem., 1968, 24, 602–608 Search PubMed;
(c) W. B. Aribia, M. Loukil, A. Kabadou and S. A. Ben, Powder Diffr., 2008, 23, 228–231 CrossRef;
(d) J. P. Laval and N. J. Boukharrata, Acta Crystallogr., Sect. C: Cryst. Struct. Commun., 2009, 65, i1–i6 CAS;
(e) S. Y. Song and K. M. Ok, Inorg. Chem., 2015, 54, 5032–5038 CrossRef CAS PubMed.
-
(a) J. Ling and T. E. Albrecht-Schmitt, J. Solid State Chem., 2007, 180, 1601–1607 CrossRef CAS;
(b) D. Pitzschke and M. Z. Jansen, Z. Anorg. Allg. Chem., 2007, 633, 1563–1567 CrossRef CAS;
(c) R. D. Shannon, Acta Crystallogr., Sect. A: Cryst. Phys., Diffr., Theor. Gen. Crystallogr., 1976, 32, 751–767 CrossRef.
- W. M. Wendlandt and H. G. Hecht, Reflectance Spectroscopy, Interscience, New York, 1966 Search PubMed.
- S. K. Kurtz and T. T. Perry, J. Appl. Phys., 1968, 39, 3798–3813 CrossRef CAS.
-
(a) CrystalClear Version 1.3.5, Rigaku Corp., Woodlands, TX, 1999 Search PubMed;
(b) G. M. Sheldrick, SHELXTL, Crystallographic Software Package, SHELXTL Version 5.1, Bruker-AXS, Madison, WI, 1998 Search PubMed;
(c) A. L. Spek, PLATON, Utrecht University, Utrecht, The Netherlands, 2001 Search PubMed.
-
(a) J. H. Kim, J. Baek and P. S. Halasyamani, Chem. Mater., 2007, 19, 5637–5641 CrossRef CAS;
(b) H. S. Ra, K. M. Ok and P. S. Halasyamani, J. Am. Chem. Soc., 2003, 125, 7764–7765 CrossRef CAS PubMed;
(c) E. O. Chi, K. M. Ok, Y. Porter and P. S. Halasyamani, Chem. Mater., 2006, 18, 2070–2074 CrossRef CAS.
-
(a) D. Altermatt and I. D. Brown, Acta Crystallogr., Sect. B: Struct. Sci., 1985, 41, 240–244 CrossRef;
(b) N. E. Brese and M. O'Keeffe, Acta Crystallogr., Sect. B: Struct. Sci., 1991, 47, 192–197 CrossRef.
- C. F. Sun, C. L. Hu, F. Kong, B. P. Yang and J. G. Mao, Dalton Trans., 2010, 39, 1473–1479 RSC.
-
(a) D. W. Lee, S. J. Oh, P. S. Halasyamani and K. M. Ok, Inorg. Chem., 2011, 50, 4473–4480 CrossRef CAS PubMed;
(b) H. K. Izumi, J. E. Kirsch, C. L. Stern and K. R. Poeppelmeier, Inorg. Chem., 2005, 44, 884–895 CrossRef CAS PubMed.
Footnote |
† Electronic supplementary information (ESI) available: X-ray crystallographic files in CIF format, simulated and experimental XRD powder patterns, IR spectra and UV-vis-NIR Diffuse reflectance spectra; calculated dipole moments as well as TGA and DTA curves. CCDC 431397–431399, 431444. For ESI and crystallographic data in CIF or other electronic format see DOI: 10.1039/c6ra17867g |
|
This journal is © The Royal Society of Chemistry 2016 |
Click here to see how this site uses Cookies. View our privacy policy here.