DOI:
10.1039/C6RA17499J
(Paper)
RSC Adv., 2016,
6, 87433-87439
Ultra-low dielectric constant materials with hydrophobic property derived from polyhedral oligomeric silsequioxane (POSS) and perfluoro-aromatics
Received
8th July 2016
, Accepted 2nd September 2016
First published on 5th September 2016
Abstract
A series of poly(arylene ether)s were synthesized with a diphenol POSS (2OH-DDSQ) and perfluoro-aromatics including decafluorobiphenyl, decaflurobenzophenone and decafluorodiphenyl sulfone via a two-step nucleophilic aromatic substitution polymerization. The chemical structures of the polymers were confirmed by 19F-NMR and 1H-NMR. All the polymers exhibit not only low dielectric constants in the range of 1.85–2.37 at 1 MHz at room temperature but also low dielectric losses, hydrophobic properties, low water uptake and good thermal properties. In particular, the DDSQ-DFPK film showed the ultra-low dielectric constant of 1.83 at 1 MHz. With increasing polarity of the perfluoro-aromatics, the water contact angles decrease from 108° to 102°.
Introduction
For exploring electronics in the microelectronics industry, materials with low dielectric constant while maintaining a low dielectric loss are urgently demanded to overcome signal delays and crosstalk noise between the metal interconnections within integrated circuits.1–8 For the novel intermetallic phase dielectrics, not only the dielectric constant, dielectric loss, moisture uptake, solubility, adhesion to Si, SiO2, Al, Cu and the other inorganic materials, and so on are also import issues.9 Especially, the large surface area of materials is sufficiently sensitive to moisture, which can occur during any exposure to air in the processing. For exploring low dielectric constant materials, the hydrophobic property and low moisture uptakes are particularly important because H2O is a polar molecule and is detrimental to the dielectric constant of materials. And the hydrophobic property and low moisture uptake have a synergistic effect on protecting the materials from water and maintaining the stability of the dielectric constants of the materials.
According to the Debye eqn (1), polymers with low dielectric constant could be achieved mainly in two ways: one is to increase the molar volume by introducing bulky pendant groups,10 nanopores11–13 etc. and another is to decrease the molar polarizability by the incorporation of the least polarizable groups like the fluorine groups.14–19 A wide of fluorinated polymers have been developed for low dielectric constant materials due to the relatively low dielectric constant and meantime, highly thermal and chemical stability, low surface energy.20–22 Among them, fluorinated poly(arylene ether)s exhibited a great potential of achieving relatively high fluorine content. Especially, poly(arylene ether)s containing perfluorinated aromatic units have been studied extensively recent years.23 Compared with some fluorinated materials of which the fluorine groups are nonsymmetrical substituents, poly(arylene ether)s containing perfluorinated aromatic tend to achieve a lower dielectric constant because of the symmetrical substitution of fluorine groups should not result in an increase in the dipole moment and the dielectric constant.16 However, there are barely fluorinated poly(arylene ether)s without nanopores of which the dielectric constant are below 2 at 1 MHz.24 To lower the dielectric constant further, polyhedral oligomeric silsesquioxane (POSS) can be introduced into the fluorinated poly(arylene ether)s for its bulky core structure and low levels of free energy which can greatly increase the molar volume and decrease the molar polarizable.
POSS, having an inner with an inorganic silicon and oxygen framework which is covered externally by organic substituents that can be functionalized with a variety of reactive groups making it suitable for the synthesis of POSS-containing materials. In the most reported cases, the POSS-based materials containing the dendronized polymers,25 grafted polymers,26–30 main-chain shaped polymers9,31 and cross-linked polymers32,33 all exhibited lower dielectric constants than the host polymers and the dielectric constants can be turned by adjusting the content of POSS. Moreover, the incorporation of POSS into polymers have not only led to low dielectric constant34,35 but also the enhancement in the properties such as thermal and mechanical stability,36,37 nonflammability,38,39 oxidative resistance,40 low water sorption41 etc.
Based on the number and type of functional groups on POSS, kinds of POSS-based materials have been made. Among numerous POSS-based materials, linear polymers containing POSS in the main chains possess better solubility, flexibility in adjustability of the chemical structure, and chain regularity than the cross-linked polymers and the grafted polymers.42 In this paper a diphenol POSS was used to make liner POSS-containing materials because of the precisely two reactive groups.43
So in this work, fluorinated poly(arylene ether)s containing POSS in the main chains with low dielectric constant were designed and prepared by the copolymerization of highly fluorinated aromatics and 2OH-DDSQ. Additionally, the dielectric constants, dielectric losses, water sorption, crystallinities, thermal properties and surface properties of the polymers were evaluated and the synergistic effects of fluorine and POSS on these properties were also discussed.
Experimental section
Materials
The diphenol POSS (2OH-DDSQ) was synthesized in our laboratory according to the literature.12 The perfluoro-monomers were abbreviated as DFPXs. Decafluorobiphenyl (DFP) and decaflurobenzophenone (DFPK) were purchased from Sigma-Aldrich and purified by recrystallization from 2-propanol prior to use. Decafluorodiphenyl sulfone (DFPS) was prepared according to reported methods.7 All other chemicals were obtained from commercial sources and used without further purification.
Methods
Thermogravimetric analysis (TGA) was performed to assess the thermal stability of membranes using a Perkin Elmer Pryis 1 TGA thermal analyzer under air atmosphere. Before performing the analysis, the samples were dried and kept inside the TGA furnace, which was isothermal at 100 °C for 20 min. Then, the samples were reheated up to 800 °C at 10 °C min−1, and the temperatures that resulted in 5% and 10% weight losses were recorded for each sample. Differential Scanning Calorimeter (DSC) measurements were performed using a Mettler Toledo DSC821e instrument at a heating rate of 10 °C min−1 from 40 °C to 250 °C for DDSQ-DFPXs under nitrogen atmosphere. IR spectra (KBr pellets or films) were conduct on a Nicolet Impact 410 Fourier Transform Infrared Spectrometer (FTIR) at room temperature (25 °C). The samples were scanned within the range of 400–3200 cm−1. 1H NMR and 19F NMR spectra was obtained using Brüker Advance 400 spectrometer (400 MHz). Gel permeation chromatograms were obtained using a Waters 410 instrument with dimethylformamide (DMF) as an eluant, at a flow rate of 1 mL min−1 using polystyrene as a standard. The dielectric constants of the polymer films (coated with silver through vacuum evaporation method) were obtained using a Hewlett-Packard 4285A apparatus at room temperature and at frequency of 103 to 106 Hz. The crystallization behavior of hybrid polymers were studied using a Rigaku D/max-2500 X-ray diffractometer with CuKα radiation (λ = 0.154 nm) as the X-ray source. Contact angle (CA) measurements were obtained at room temperature using a POWEREACH/JC2002C2 CA meter with pure water as probe liquid. The water uptake of the polymer was measured by immersion of the polymer films in the deionized water at 100 °C until the weight of the polymer film is no longer increased, followed by wiping with tissue paper for removal of any water on the surface. The water uptakes were calculated from the difference in the weights before and after this procedure.
Polymer synthesis
As shown in Scheme 1, DDSQ-DFP was synthesized via a two-step nucleophilic aromatic substitution procedure. The other two polymers were prepared from the corresponding decafluorodiphenyl monomers and 2OH-DDSQ with the ratio of [DFPX]/[2OH-DDSQ] = 2.01/2 using the same procedure as described above with the reaction time and reaction temperature listed in Table 1. The yield was above 90%. The molecular weights of the polymers were measured with GPC and listed in Table 1. A three-necked flask equipped with a mechanical stirrer, a nitrogen inlet, and a Dean–Stark trap with a condenser was added 2OH-DDSQ (1.4822 g, 1 mmol), DFP (0.3341 g, 1 mmol), anhydrous K2CO3 (0.1658 g, 1.2 mmol), DMAc (9 mL) and toluene (4.5 mL). The system was allowed to reflux for 3 h, and then the toluene was removed. After removing toluene, the reaction mixture was cooled to 40 °C and then DFP was added. The temperature was maintained for 2 h before the polymerization completed. The viscous solution was then poured into 50 mL of ethanol. The polymer was refluxed in deionized water and ethanol several times to remove the salts and solvents and was dried at 120 °C for 24 h (90% yield). 1H NMR (400 MHz, DMSO-d6), δ(ppm): 0.14 (m), 0.63 (m), 1.56 (m), 2.42 (s), 3.68 (s), 7.37 (m). 19F NMR (400 MHz, DMSO-d6), δ(ppm): −139.3 (4F), −155.43 (4F), IR (KBr cm−1): 2927 (C–H stretching), 1643, 1507 (phenyl ring), 1304 (C–F stretching), 1071, 480 (Si–O stretching), 725 (Si–C stretching).
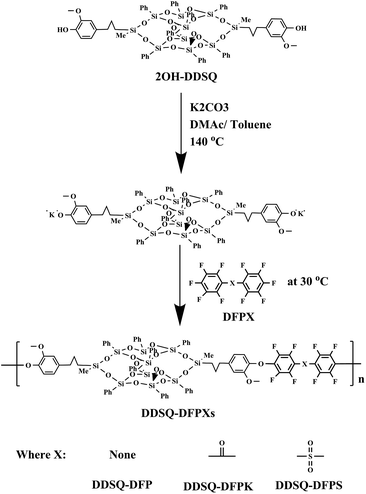 |
| Scheme 1 Reaction route for the preparation of DDSQ-DFPXs. | |
Table 1 The preparation and characterization of the DDSQ-PAEKs
DDSQ-DFPXs |
Timea (h) |
Temperaturea (°C) |
Mnb (kDa) |
PDIc |
The polymerization was conducted in DMAc at different temperatures. Mn values were determined by GPC in DMF with PS as standard. PDI values (Mw/Mn) were determined by GPC in DMF with PS as standard. |
DDSQ-DFP |
2 |
40 |
25.3 |
2.13 |
DDSQ-DFPK |
6 |
30 |
21.6 |
2.25 |
DDSQ-DFPS |
4.5 |
30 |
24.8 |
2.31 |
The other two polymers were prepared from the corresponding decafluorodiphenyl monomers and 2OH-DDSQ with the ratio of [DFPX]/[2OH-DDSQ] = 2.01/2 using the same procedure as described above with the reaction time and reaction temperature listed in Table 1. The molecular weights of the polymers were measured with GPC and listed in Table 1.
DDSQ-DFPK (87% yield). 1H NMR (400 MHz, DMSO-d6), δ(ppm): 0.12 (m), 0.64 (m), 1.55 (m), 2.36 (s), 3.55 (s), 7.376 (m). 19F NMR (400 MHz, DMSO-d6), δ(ppm): −143.3 (4F), −155.4 (4F). IR (KBr cm−1): 2925 (C–H stretching), 1639, 1490 (phenyl ring), 1302 (C–F stretching), 1069, 475 (Si–O stretching), 720 (Si–C stretching).
DDSQ-DFPS (89% yield). 1H NMR (400 MHz, DMSO-d6), δ(ppm): 0.11 (m), 0.65 (m), 1.51 (m), 2.35 (s), 3.77 (s), 7.37 (m). 19F NMR (400 MHz, DMSO-d6), δ(ppm): −138.7 (4F), −154.5 (4F). IR (KBr cm−1): 2920 (C–H stretching), 1635, 1483 (phenyl ring), 1298 (C–F stretching), 1069, 478 (Si–O stretching), 720 (Si–C stretching).
Film preparation for the measurement
The polymer solutions were dissolved in DMAc (1 g/10 mL). A Teflon syringe filter with a pore size of 0.12 μm was used to filter the solution onto an 2 cm × 5 cm smooth Teflon plate with a programmed procedure (60 °C, 12 h; 80 °C, 12 h; 120 °C, 6 h). The temperature was maintained at 120 °C for another 6 h, wherein certain level of transparency, smoothness, and toughness were obtained on the films.
Results and discussion
Polymer synthesis
The perfluoro-monomers studied in this paper have a general formula as illustrated in Scheme 1. The X linkages of the monomers are none for DFP, ketone for decafluorobenzone (DFPK) and sulfone for decafluorodiphenyl sulfone (DFPS). The polymerization of DFPX and 2OH-DDSQ was carried out in a two-step nucleophilic aromatic substitution procedure. In this approach, the 2OH-DDSQ was first converted to phenoxide and then further reacted with perfluoro-monomers at a low temperature. The reactions are displayed in Scheme 1 and analyzed by 19F NMR in Fig. 1. It can be seen that the produced polymers have clean 19F NMR spectra and the two major peaks are assigned to the fluorine atoms on the main chain as indicated in the Fig. 1. The polymers were also identified by 1H NMR spectroscopy. Fig. 2 shows the 1H NMR of DDSQ-DFPXs. Signals of proton at 0.12, 0.71–2.46 and 3.53 ppm are assigned to the protons of methyl, methylene, and methoxy. The peaks at 6.2–8.5 ppm are assigned to the protons of aromatic ring.
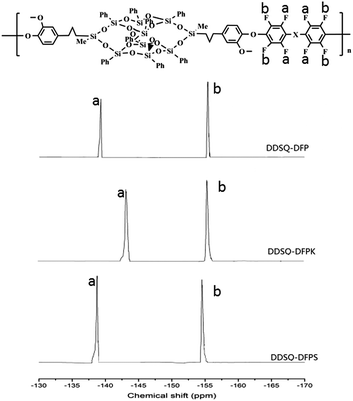 |
| Fig. 1 19F NMR spectra of the DDSQ-DFPXs in DMSO-d6. | |
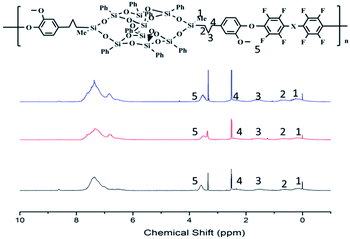 |
| Fig. 2 1H NMR spectra of DDSQ-DFPXs in DMSO-d6. | |
Water contact angles and water sorption
Hydrophobic property and low water sorption are especially important for low dielectric constant materials because H2O is a molecule with strong polar which is detrimental to the dielectric constant of materials. The hydrophobic property and low moisture sorption have a synergistic effect on protecting the materials from water and maintaining the stability of the dielectric constants of the materials.
The wettabilities of the resulting surfaces were examined by measuring static water CAs and are listed in Table 2 and shown in Fig. 3. All the surfaces exhibit hydrophobic properties. And compared with the corresponding non-fluorinated polymers, the water CAs of the DDSQ-DFPXs are much higher13 confirming the significance of a high fluorine content in the polymer for reducing the surface tension of the polymer films. Because of low polarity of POSS and fluorine, POSS and fluorine have a synergistic effect on the low surface tension. Among all the polymers, DDSQ-DFP shows the highest value of the contact angle of 108° which is close to that of Teflon type materials. With increasing polarity of the DFPXs from DFPK to DFPS, the water CAs decreased from 105° to 102°.
Table 2 Summary of the properties of DDSQ-DFPXs
DDSQ-DFPXs |
Tga (°C) |
Td5b (°C) |
Td10b (°C) |
Residued (%) |
Dielectric constantc |
Dielectric lossc |
Water uptakee (%) |
CA (deg.) |
DSC at a heating rate of 10 °C min−1 in nitrogen. Temperature at which 5% and 10% weight losses were recorded by thermogravimetry at a heating rate of 10 °C min−1 in air. Dielectric constant and dielectric loss were measured at 1 MHz. The yields of degradation residues were obtained at 800 °C in air. Calculated from the difference in weights before and after immersion of the polymer films in water. |
DDSQ-DFP |
158 |
417 |
434 |
32.9 |
1.99 |
6 × 10−3 |
0.09 |
108 |
DDSQ-DFPK |
144 |
408 |
440 |
31.9 |
1.83 |
1.7 × 10−2 |
0.17 |
105 |
DDSQ-DFPS |
151 |
358 |
380 |
31.2 |
2.37 |
2 × 10−2 |
0.28 |
102 |
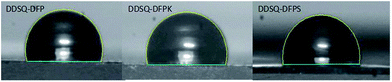 |
| Fig. 3 Plot of surface water contact angles of DDSQ-DFPXs films. | |
As shown in Table 2, the water sorption increases with the increasing polarity of the DFPXs. All the polymers exhibit appreciably low water sorption in the range of 0.09–0.28% which should be attributed to the low polarity of POSS molecules. The hydrophobic properties of the surfaces also help with the low water sorption. As mentioned above, the polymers exhibited the water sorption as DDSQ-DFP (0.09%) lower than the DDSQ-DFPK (0.17%) and DDSQ-DFPS (0.28%).
Crystallinities and solubility
The crystallinities of DDSQ-DFPXs were examined by the wide-angle X-ray diffraction (WAXRD) and all WAXRD curves show two broad peaks, about 7.1–7.3° and 18.5–19.0° for the DDSQ-DFPXs (Fig. 4). The results indicate that all DDSQ-DFPXs are amorphous in nature, which is mainly due to the nanoscale dimensions of bulky POSS, which disrupts the regularity of the molecule chains and inhibits the close packing of the polymer chains.
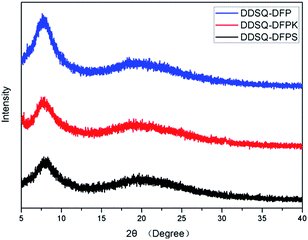 |
| Fig. 4 WAXRD profiles of DDSQ-DFPXs (the data is offset for clarity in intensity). | |
The solubility of the DDSQ-DFPXs in selected solvents are listed in Table 3. All the DDSQ-DFPXs could completely dissolve in polar solvents such as DMAc, DMF and NMP. It is apparent that the good solubility of these polymers is mostly dependent on the presence of bulky POSS in the polymer backbone, which increases the chain distance, inhibits the chain packing, and decreases the interaction of the polymer chains. The good solubility of DDSQ-DFPXs makes it easier for the preparation of films and further device fabrication.
Table 3 Solubility of the DDSQ-DFPXsa,b
PAEKs |
DMF |
DMAC |
NMP |
DMSO |
THF |
Chloroform |
Solubility under a mass of liquid: 0.1 g mL−1. ++, soluble at room temperature; −−, insoluble even on heating; NMP, N-methyl-2-pyrrolidone; DMAc, N,N-dimethylacetamide; DMF, N,N-dimethylformamide; DMSO, dimethylsulfoxide; THF, tetrahydrofuran. |
DDSQ-DFP |
++ |
++ |
++ |
++ |
−− |
++ |
DDSQ-DFPK |
++ |
++ |
++ |
++ |
−− |
−− |
DDSQ-DFPS |
++ |
++ |
++ |
++ |
−− |
−− |
Thermal properties
The thermal properties of the DDSQ-DFPXs were evaluated by DSC and TGA, and the data are listed in Table 2. No melting endotherms are observed in DSC traces for the DDSQ-DFPXs (Fig. 5), which suggests their amorphous nature. Among the polymers, DDSQ-DFP shows the highest Tg value 160 °C because of the effect of the rigid biphenylene polymer backbone which hinders the movement of the chain segment. With the increasing polarity of the DFPXs, DDSQ-DFPS exhibits a higher Tg than DDSQ-DFPK, reflecting a higher intermolecular force in the polymer. Meanwhile, the polymers don't show very high Tgs. Though the rigid molecular structure of POSS can lead a higher Tg, both the greatly increased free volume of DDSQ-DFPXs and the flexible aliphatic chains possessed by 2OH-DDSQ results in the easier movement of the chain segment.
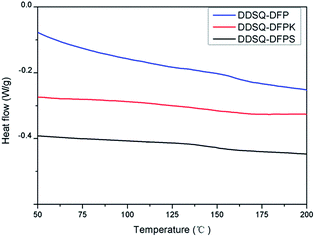 |
| Fig. 5 DSC curves of the second heating scan after the DDSQ-DFPXs were quenched into liquid nitrogen from 200 °C (the data is offset for clarity in intensity). | |
Seen from the TGA data Table 2 and Fig. 6, the temperatures at 5% weight loss (Td5) are in the range of 358–417 °C, the 10% weight loss (Td10) temperatures of 480–444 °C in air for the DDSQ-DFPXs, indicating a fairly high thermal stability of the polymers. The DDSQ-DFPXs display two steps in degradation progress. The first step originated from the degradation of active alkyl chain and the second step originated from the degradation of frameworks, such as the aromatic ring and POSS cage. The DDSQ-DFPXs display high residuals of degradations which are all above 30%. The high yields of degradation residues are ascribed to the ceramic formation from POSS moiety during the thermal decomposition.
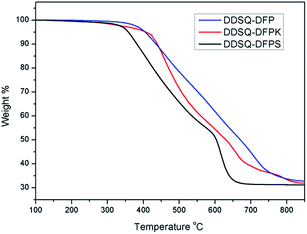 |
| Fig. 6 TGA curves of the DDSQ-DFPXs. | |
Dielectric constant
The dielectric constants of the DDSQ-DFPXs as shown in Fig. 7, measured on the thin films, are in the range of 1.83–2.37 at 1 MHz. Among the polymers, DDSQ-DFPK exhibits a ultra-low dielectric constant of 1.83 at 1 MHz which is the lowest dielectric constant.
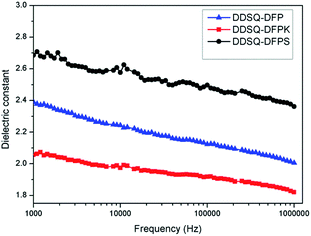 |
| Fig. 7 Frequency dependence of dielectric constant of DDSQ-DFPXs films. | |
The quantitative relation between the dielectric constant and properties of the molecules is described by the Debye eqn (1):
|
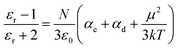 | (1) |
with
N the number of molecules per m
3,
μ the orientation polarizability,
k the Boltzmann constant,
T the temperature in K,
εr dielectric constant of materials and
ε0 the dielectric constant of vacuum. The terms
αe and
αd represent the electronic and distortion polarization in the molecule, while the term
μ2/3
kT stems from the thermal averaging of permanent electric dipole moments in the presence of an applied field.
Seen from the Debye equation, it can be found that the incorporation of the low polarizable groups C–F and Si–O–Si which decrease αe contributes to the ultra-low dielectric constants. The DFPS has a higher polarity than the DFPX, expressing a higher μ2/3kT and αe values for the DDSQ-DFPS. Especially, the bond angle of the X linkage in DDSQ-DFP is 180°, different from the 122° for ketone in the DDSQ-DFPK and 104° for sulfone in the DDSQ-DFPS, suggesting the highest degree of symmetry. And it means that DDSQ-DFP gets the lowest μ2/3kT. However, with the rigid biphenylene added, the molecular chains of the DDSQ-DFP tend to be more regular and the free volume is relatively decreased reflecting a higher N value and the higher dielectric constant than the DDSQ-DFPK.
It can also be seen that the effect of the density N on the dielectric constants of the polymers is stronger than the effect of polarizability since reducing density allows reducing the dielectric constants to the extreme value. And the incorporation of bulky core structured POSS molecule greatly enhance the free volume of the polymers resulting in the low density N of the polymers. To clarify it further, the dielectric constant of porous materials is described by the following eqn (2):
|
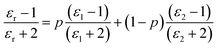 | (2) |
In this equation ε1 is the dielectric constant inside the pores, ε2 is dielectric constant of the polymer skeleton, and p is the polymer porosity. And because of the nanopores POSS possessed, the term p(ε1 − 1)/(ε1 + 2) is almost equal to zero. And according to this equation, the low polarizability of the POSS skeleton also attributes to the low dielectric constant of the materials.
Seen from the Fig. 8, the dielectric loss factors at 1 MHz for the DDSQ-DFPXs are at a very low scale, which is between 6 × 10−3 and 2 × 10−2. Because of the highest degree of symmetry, DDSQ-DFP exhibits the lowest dielectric loss of 6 × 10−3.
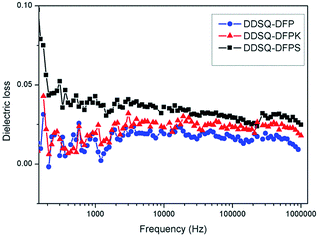 |
| Fig. 8 Frequency dependence of dielectric loss of DDSQ-DFPXs films. | |
Conclusion
A series of DDSQ-DFPXs with low dielectric constants were prepared from 2OH-DDSQ and DFPXs in DMAc at a low temperature via a two-step nucleophilic aromatic substitution procedure. The polymers are shown to be with high molecular weights and well-defined linear structure. As low dielectric constant materials against the advanced microelectronic applications, all the polymers exhibit low dielectric constant, low dielectric loss, low water uptake and hydrophobic properties. With a relatively low polarity and small free volume, DDSQ-DFPK demonstrates the lowest dielectric constant of 1.83 at 1 MHz. With the highest degree of symmetry and the lowest the polarity, DDSQ-DFP exhibits the lowest dielectric loss of 6 × 10−3, the highest water CA of 108° and the lowest water uptake of 0.09%. With increasing polarity of the DFPXs from ketone to sulfone, the water CAs decreased from 105° to 102°.
References
- P. M. Hergenrother, B. J. Jensen and S. J. Havens, Polymer, 1988, 29, 358–369 CrossRef CAS.
- K. H. Gardner, B. S. Hsiao, R. R. Matheson and B. A. Wood, Polymer, 1992, 33, 2483–2495 CrossRef CAS.
- S. R. Sheng, Q. Y. Luo, Y. Huo, Z. Liu, X. L. Liu and C. S. Song, J. Appl. Polym. Sci., 2008, 107, 683–687 CrossRef CAS.
- X. C. Ge, Y. Xu, M. Xiao, Y. Z. Meng and A. S. Hay, Eur. Polym. J., 2006, 42, 1206–1214 CrossRef CAS.
- A. S. More, S. K. Pasale, P. N. Honkhambe and P. P. Wadgaonkar, J. Appl. Polym. Sci., 2011, 121, 3689–3695 CrossRef CAS.
- I. Y. Jeon, L. S. Tan and J. B. Baek, J. Polym. Sci., Part A: Polym. Chem., 2009, 47, 3326–3336 CrossRef CAS.
- A. Fortney and E. Fossum, Polymer, 2012, 53, 2327–2333 CrossRef CAS.
- X. L. Zhao, Q. Zhang, L. X. Xue, Q. R. Shi and K. Tao, J. Appl. Polym. Sci., 2013, 130, 1058–1063 CrossRef CAS.
- S. Wu, T. Hayakawa and H. Oikawa, Macromolecules, 2008, 41, 3481–3487 CrossRef CAS.
- G. B. Wang, Z. Geng, X. L. Zhu, S. L. Zhang and X. Liu, High Perform. Polym., 2010, 22, 779–798 CrossRef CAS.
- H. R. Kyuyoung, J. Brian, C. Kyeung-Keun and R. Moonhor, RSC Adv., 2015, 5, 87084–87089 RSC.
- K. Ohno, S. Sugiyama, K. Koh, Y. Tsujii, T. Fukuda, M. Yamahiro, Y. Yamamoto, N. Ootake and K. Watanabe, Macromolecules, 2004, 37, 8517–8522 CrossRef CAS.
- W. H. Zhang, J. D. Xu, X. S. Li, G. H. Song and J. X. Mu, J. Polym. Sci., Part A: Polym. Chem., 2014, 52, 780–788 CrossRef CAS.
- F. W. Mercer, M. M. Fone, V. N. Reddy and A. A. Goodwin, Polymer, 1997, 38, 1989–1995 CrossRef CAS.
- K. Kimura, Y. Tabuchi, Y. Yamashita, P. E. Cassidy, J. W. Fitch III and Y. Okumura, Polym. Adv. Technol., 2000, 11, 757–765 CrossRef CAS.
- B. J. Liu, G. B. Wang, W. Hu, Y. H. Jin, C. H. Chen, Z. H. Jiang, W. J. Zhang, Z. W. Wu and Y. Wei, J. Polym. Sci., Part A: Polym. Chem., 2002, 40, 3392–3398 CrossRef CAS.
- B. J. Liu, W. Hu, C. H. Chen and Z. H. Z. W. Wu, Polymer, 2004, 45, 3241–3247 CrossRef CAS.
- Y. M. Niu, X. L. Zhu, L. Z. Liu, Y. Zhang, G. B. Wang and Z. H. Jiang, React. Funct. Polym., 2006, 66, 559–566 CrossRef CAS.
- H. L. Yao, S. R. Sheng, J. W. Jiang, X. L. Liu and C. S. Song, J. Fluorine Chem., 2012, 144, 176–181 CrossRef.
- J. M. Desimone, Z. Guan and C. S. Elsbernd, Science, 1992, 257, 945–947 CAS.
- J. W. Martin, M. M. Smithwick and B. M. Braune, Environ. Sci. Technol., 2004, 38, 373–380 CrossRef CAS PubMed.
- T. R. Dargaville, G. A. George and D. J. T. Hill, Prog. Polym. Sci., 2003, 28, 1355–1376 CrossRef CAS.
- J. Ding, X. Du and M. Day, Macromolecules, 2007, 40, 3145–3153 CrossRef CAS.
- I. Fisher and M. Eizenberg, Thin Solid Films, 2008, 516, 4111–4121 CrossRef CAS.
- Z. S. Ge, D. Wang and Y. M. Zhou, Macromolecules, 2009, 42, 2903–2910 CrossRef CAS.
- C. M. Leu, G. M. Reddy, K. H. Wei and C. F. Shu, Chem. Mater., 2003, 15, 2261–2265 CrossRef CAS.
- C. M. Leu, Y. T. Chang and K. H. Wei, Macromolecules, 2003, 36, 9122–9127 CrossRef CAS.
- C. M. Leu, Y. T. Chang and K. H. Wei, Chem. Mater., 2003, 15, 3721–3727 CrossRef CAS.
- Y. W. Chen and E. T. Kang, Mater. Lett., 2004, 58, 3716–3719 CrossRef CAS.
- Y. W. Chen, L. Chen, H. R. Nie and E. T. Kang, J. Appl. Polym. Sci., 2006, 99, 2226–2232 CrossRef CAS.
- S. Wu, T. Hayakawa, R. Kikuchi, S. J. Grunzinger and M. Kakimoto, Macromolecules, 2007, 40, 5698–5705 CrossRef CAS.
- Y. S. Ye, W. Y. Chen and Y. Z. Wang, J. Polym. Sci., Part A: Polym. Chem., 2006, 44, 5391–5402 CrossRef CAS.
- M. A. Wahab, K. Y. Mya and C. B. He, J. Polym. Sci., Part A: Polym. Chem., 2008, 46, 5887–5896 CrossRef.
- Y. J. Lee, J. M. Huang, S. W. Kuo, J. S. Lu and F. C. Chang, Polymer, 2005, 46, 173–181 CrossRef CAS.
- Z. Geng, M. X. Huo, J. X. Mu, S. L. Zhang, Y. N. Lu, J. S. Luan, P. F. Huo, Y. L. Du and G. B. Wang, J. Mater. Chem. C, 2014, 2, 1094–1103 RSC.
- H. Y. Xu, S. W. Kuo, J. S. Lee and F. C. Chang, Macromolecules, 2002, 35, 8788–8793 CrossRef CAS.
- H. Y. Xu, B. H. Yang, J. F. Wang, S. Y. Guang and C. Li, Macromolecules, 2005, 38, 10455–10460 CrossRef CAS.
- Q. He, L. Song, Y. Hu and S. Zhou, J. Mater. Sci., 2009, 44, 1308–1316 CrossRef CAS.
- W. C. Zhang, X. M. Li and R. J. Yang, J. Appl. Polym. Sci., 2012, 124, 1848–1857 CrossRef CAS.
- B. H. Augustine, W. C. Hughes, K. J. Zimmermann, A. J. Figueiredo, X. W. Guo, C. C. Chusuei and J. S. Maidment, Langmuir, 2007, 23, 4346–4350 CrossRef CAS PubMed.
- S. M. Lee and T. H. Kwon, J. Micromech. Microeng., 2007, 17, 687–692 CrossRef CAS.
- J. D. Xu, W. H. Zhang, Q. Y. Jang, J. X. Mu and Z. H. Jiang, Polymer, 2015, 62, 77–85 CrossRef CAS.
- J. Choi, A. F. Yee and R. M. Laine, Macromolecules, 2004, 37, 3267–3276 CrossRef CAS.
|
This journal is © The Royal Society of Chemistry 2016 |