DOI:
10.1039/C6RA17491D
(Paper)
RSC Adv., 2016,
6, 81013-81016
Elemental sulfur mediated synthesis of benzoxazoles, benzothiazoles and quinoxalines via decarboxylative coupling of 2-hydroxy/mercapto/amino-anilines with cinnamic acids†
Received
8th July 2016
, Accepted 18th August 2016
First published on 19th August 2016
Abstract
An easy and practical method has been developed for the synthesis of 2-benzylbenzoxazoles and 2-benzylbenzothiazoles using sulfur mediated decarboxylative coupling of cinnamic acids with 2-hydroxyanilines and 2-mercaptoanilines respectively under metal- and solvent-free conditions. However, the reaction of 2-aminoanilines with cinnamic acids leads to the formation of 2-arylquinoxalines under the same set of reaction conditions. The transformation is versatile and compatible with a number of functional groups.
Introduction
The past few years have witnessed the emergence of transition metal- and photo-catalyzed decarboxylative cross-coupling reactions as a powerful tool to construct carbon–carbon and carbon–heteroatom bonds, with an enormous impact on organic synthesis, medicinal chemistry and material science.1 Carboxylic acids and their derivatives are widely used coupling partners as they are non-toxic, inexpensive, stable and readily available in great structurally diversity.2 The decarboxylative C–N coupling strategy involving the synthesis of complex molecules is a fascinating and challenging theme, yet only a few reports do exist involving this approach.3
Benzazoles are privileged heterocyclic scaffolds found in many natural products with various pharmaceutical applications. Particularly, 2-substituted benzazoles are widely present in drugs to combat various diseases.4 Traditional methods for the synthesis of 2-substituted benzazoles involve the reaction of o-hydroxy/mercapto anilines with acylhalides,5 acids,6 aldehydes,7 β-diketones,8 alcohols,9 nitriles,10 amines,11 and methylarenes12 under high temperature/strong oxidative conditions. Recently, 2-benzylbenzoxazoles have been synthesized by the reaction of benzoxazoles with benzyl halides13 or benzyl carbonates,14 whereas 2-aryl/aroyl-benzothiazoles have been prepared by the reaction of 2-aminothiophenols with acetophenones, styrenes, and phenylacetylenes.15,16 Quinoxalines constitute another important heterocycle frequently present in biologically and pharmaceutically active compounds.17 The commonly used methods for the synthesis of quinoxalines involve the direct cyclization reaction of o-phenylenediamines with 1,2-dicarbonyl derivatives,18 α-hydroxy ketones,19 α-haloketones,20 vicinal diols,21 diazoketones,22 and phenylacetylene.23 Recently the synthesis of quinoxalines has been achieved by the reaction of o-nitroanilines with phenethylamines,24 and oxidative azidation/cyclization of N-arylenamines/N-aryl ketimines with azides under mild conditions,25 albeit using transition metal catalysts. However, these methods are now and then cumbersome and require metal salts, harsh conditions, and longer reaction time. Therefore, development of an efficient and direct protocol for the synthesis of 2-benzylbenzazoles and quinoxalines by using simple and readily available starting materials under metal-free condition is highly exigent.
In light of the above and as a part of our recent interest in sulfur mediated reactions26 and other protocols,27 we disclose herein a new strategy involving elemental sulfur mediated decarboxylative coupling of 2-hydroxy/mercapto/amino-anilines with cinnamic acids/phenylpropiolic acid for the synthesis of 2-benzylbenzoxazoles, 2-benzylbenzothiazoles and 2-arylquinoxalines respectively under metal- and solvent-free conditions (Scheme 1). To the best of our knowledge, this is the first report in which aromatic amines undergo the Willgerodt–Kindler rearrangement.
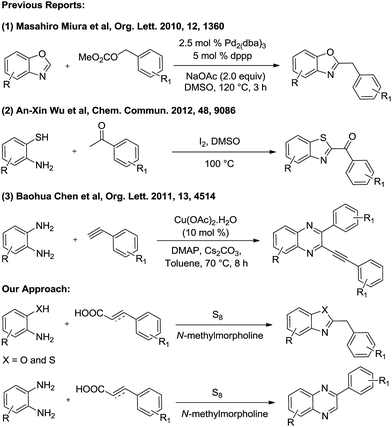 |
| Scheme 1 Previous reports and present strategy. | |
Results and discussion
The study was envisioned to achieve the synthesis of 2-substituted benzoxazole by the reaction of cinnamic acid with 2-aminophenol. To start with, a model reaction involving 2-aminophenol (1a) and cinnamic acid (3a) was undertaken in the presence of elemental sulfur and N-methylmorpholine in DMSO at 120 °C for 15 h. To our utmost delight, the desired product 2-benzylbenzoxazole (4a) was formed in 35% (Table 1, entry 1).
Table 1 Optimization of the reaction conditionsa

|
Entry |
Base (x equiv.) |
Solvent |
Temp (°C) |
Yieldb (%) |
Conditions: 2-aminophenol 1a (1 mmol), cinnamic acid 3a (1 mmol), elemental sulfur (3 mmol, 96 mg), 15 h. Isolated yield. 20 h. 10 h. Sulfur (4 mmol). Sulfur (2 mmol). |
1 |
N-Methylmorpholine (2) |
DMSO |
120 |
35 |
2 |
N-Methylmorpholine (2) |
DMSO |
130 |
51 |
3 |
N-Methylmorpholine (2) |
DMSO |
140 |
48 |
4 |
N-Methylmorpholine (2) |
DMSO |
130 |
50c |
5 |
N-Methylmorpholine (2) |
DMSO |
130 |
43d |
6 |
DABCO (2) |
DMSO |
130 |
25 |
7 |
DMAP (2) |
DMSO |
130 |
27 |
8 |
Pyridine (2) |
DMSO |
130 |
15 |
9 |
— |
DMSO |
130 |
0 |
10 |
K2CO3 (2) |
DMSO |
130 |
0 |
11 |
Na2CO3 (2) |
DMSO |
130 |
0 |
12 |
K3PO4 (2) |
DMSO |
130 |
0 |
13 |
t-BuOK (2) |
DMSO |
130 |
0 |
14 |
NaHCO3 (2) |
DMSO |
130 |
0 |
15 |
CH3COONa (2) |
DMSO |
130 |
0 |
16 |
N-Methylmorpholine (2) |
— |
130 |
67 |
17 |
N-Methylmorpholine (3) |
— |
130 |
72 |
18 |
N-methylmorpholine (4) |
— |
130 |
71 |
19 |
N-Methylmorpholine (4) |
— |
130 |
70e |
20 |
N-Methylmorpholine (3) |
— |
130 |
65f |
Increasing the reaction temperature to 130 °C increased the product yield to 51% (entry 2). However, a further increase in the reaction temperature (140 °C) and time (20 h) did not improve the product yield again (entries 3 & 4), but a decrease in the reaction time (10 h) diminished the product yield to 43% (entry 5). To further advance the product yield, different organic bases such as DABCO, DMAP and pyridine were screened (entries 6–8), but none of them could match the efficacy of N-methylmorpholine. Notably, the formation of the product was not observed in the absence of the base (entry 9). Moreover, the use of inorganic bases like K2CO3, Na2CO3, K3PO4, t-BuOK, NaHCO3 and CH3COONa in the solvent DMSO remained futile (entries 10–15). Interestingly, when the reaction was conducted under solvent-free conditions, the yield of the product was improved to 67% (entry 16). Increasing the molar ratio of the base (3 equiv.) brought about some improvement in the yield (entry 17). Further increase in the molar equivalence of base and elemental sulfur did not make any positive change (entries 18 & 19), but decreasing the molar ratio of sulfur (2.0 equiv.) lowered down the product yield slightly (entry 20).
Having established the optimum reaction conditions (entry 17), the scope and limitations of the reaction were investigated in detail by using different cinnamic acids and 2-aminophenols. The outcome is summarized in Scheme 2. Cinnamic acids having substituent such as chloro, bromo, methyl, and methoxy at different positions were made to react with 2-aminophenol, 2-amino-4-methylphenol and 2-amino-4-chlorophenol under the established set of reaction conditions to afford the desired products 4a–j in reasonably good yields. 3-(Thiophen-2-yl)acrylic acid also underwent the reaction smoothly to give the product 4e in 59% yield. Nevertheless, 4-nitrocinnamic acid failed to provide the product 4k under the stipulated conditions. After ascertaining the viability of sulfur mediated decarboxylative coupling of cinnamic acids with 2-aminophenols, we next turned our attention to the synthesis of 2-substituted benzothiazoles by using 2-aminothiophenols. As anticipated, when the reaction was conducted using cinnamic acid and 2-aminothiophenol under the same conditions, the product 2-benzylbenzothiazole (5a) was formed in 71% yield. The versatility of the reaction was further explored by using different cinnamic acids with 2-aminothiophenol and 2-amino-4-chlorobenzenethiol under the identical conditions to afford the desired products 5a–h in high yields (cf. Scheme 2). The heteroaromatic 3-(thiophen-2-yl)acrylic acid also offered the product 5e in 51% yield. It is worth noting that the disubstituted 3-(2,4-dichlorophenyl)acrylic acid also reacted nicely giving 5f in 55% yield.
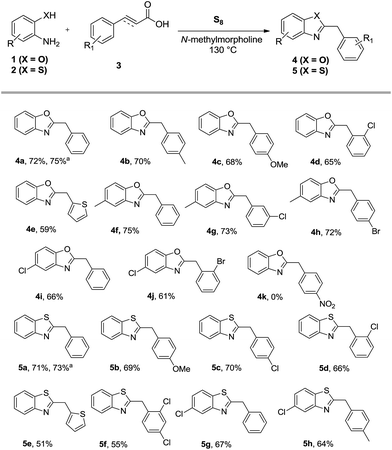 |
| Scheme 2 The scope of the reaction between cinnamic acids and 2-hydroxy/mercapto anilines. Conditions: 1/2 (1 mmol), cinnamic acid 3 (1 mmol), N-methylmorpholine (3 mmol), sulfur (3 mmol, 96 mg) for 15 h. aWith phenylpropiolic acid. | |
Finally, the process was extended to the reaction of cinnamic acids and o-phenylenediamines with an aim to achieve the synthesis of 2-benzylbenzimidazoles. Surprisingly, the formation of 2-phenylquinoxalines 7a–g instead of expected 2-benzylbenzimidazoles was observed in good yields by the reaction of different cinnamic acids with 2-aminoanilines under the established conditions (Scheme 3).
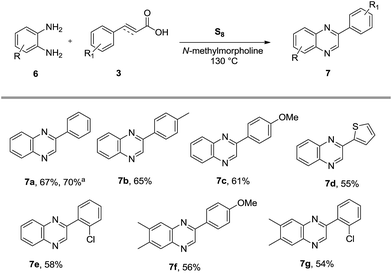 |
| Scheme 3 The scope of the reaction between cinnamic acids and 1,2-phenylenediamines. Conditions: 2-aminoaniline 6 (1 mmol), cinnamic acid 3 (1 mmol), N-methylmorpholine (3 mmol), sulfur (3 mmol, 96 mg) for 15 h. aWith phenylpropiolic acid. | |
After concluding the above results, the reaction was also carried out under the same set of reaction conditions by replacing cinnamic acid with phenylpropiolic acid. Notably, the representative reaction between 2-hydroxy/mercapto/amino-anilines and phenylpropiolic acid under the established conditions led to the formation of 2-benzylbenzoxazole (4a), 2-benzylbenzothiazole (5a) and 2-arylquinoxaline (7a) in 75%, 73% and 70% yields respectively.
In order to gain an insight into the mechanistic pathway, a typical reaction was undertaken in the presence of a radical scavenger TEMPO (Scheme 4). As a result, the reaction proceeded well without any effect, thereby excluding the possibility of involvement of any radical intermediate.
 |
| Scheme 4 Radical trapping experiment. | |
Based on the radical trapping experiment and existing literature,28 a plausible mechanism is outlined in Fig. 1.
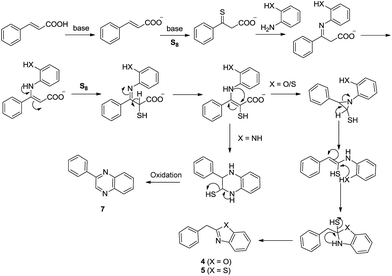 |
| Fig. 1 Plausible mechanism. | |
Conclusion
In summary, a practical approach has been developed to accomplish 2-benzylbenzoxazoles, 2-benzylbenzothiazoles and 2-arylquinoxalines by the reaction of cinnamic acids and 2-hydroxy/mercapto/amino-anilines via decarboxylative C–N coupling followed by cyclization. Aromatic amines have been successfully employed for the first time in Willgerodt–Kindler type rearrangement. The methodology is operationally simple, uses readily available starting materials, and is free from the use of metals, solvents and external oxidants.
Acknowledgements
We are thankful to the Department of Science and Technology, Government of India, for providing financial assistance. T. G., R. V. and S. K. are thankful to the CSIR, New Delhi for providing research fellowships.
Notes and references
-
(a) O. Baudoin, Angew. Chem., Int. Ed., 2007, 46, 1373 CrossRef CAS PubMed;
(b) J. D. Weaver, A. Recio, A. J. Grenning and J. A. Tunge, Chem. Rev., 2011, 111, 1846 CrossRef CAS PubMed;
(c) N. Rodríguez and L. J. Goossen, Chem. Soc. Rev., 2011, 40, 5030 RSC;
(d) A. J. Borah and G. Yan, Org. Biomol. Chem., 2015, 13, 8094 RSC;
(e) Q. Song, Q. Feng and M. Zhou, Org. Lett., 2013, 15, 5990 CrossRef CAS PubMed;
(f) J. Liu, Q. Liu, H. Yi, C. Qin, R. Bai, X. Qi, Y. Lan and A. Lei, Angew. Chem., Int. Ed., 2014, 53, 502 CrossRef CAS PubMed.
-
(a) H. Beyer and W. Walter, Lehrbuch der Organischen Chemie, 24 Aufl., Hirzel Verlag, Stuttgart, 2004 Search PubMed;
(b) K. P. C. Vollhardt and N. E. Schore, Organische Chemie, 3 Aufl., Wiley-VCH, Weinheim, 2000, p. S. 893 Search PubMed.
-
(a) W. Jia and N. Jiao, Org. Lett., 2010, 12, 2000 CrossRef CAS PubMed;
(b) Y. Zhu, X. Li, X. Wang, X. Huang, T. Shen, Y. Zhang, X. Sun, M. Zou, S. Song and N. Jiao, Org. Lett., 2015, 17, 4702 CrossRef CAS PubMed;
(c) R. Kancherla, T. Naveen and D. Maiti, Chem.–Eur. J., 2015, 21, 8723 CrossRef CAS PubMed.
-
(a) I. Ćaleta, M. Kralj, M. Marjanović, B. Bertoša, S. Tomić, G. Pavlović, K. I. Pavelić and G. Karminski-Zamola, J. Med. Chem., 2009, 52, 1744 CrossRef PubMed;
(b) W. S. Saari, J. M. Hoffman, J. S. Wai, T. E. Fisher, C. S. Rooney, A. M. Smith, C. M. Thomas, M. E. Goldman and J. A. O'Brien, J. Med. Chem., 1991, 34, 2922 CrossRef CAS PubMed;
(c) S. Aiello, G. Wells, E. L. Stone, H. Kadri, R. Bazzi, D. R. Bell, M. F. G. Stevens, C. S. Matthews, T. D. Bradshaw and A. D. Westwell, J. Med. Chem., 2008, 51, 5135 CrossRef CAS PubMed;
(d) A. D. Westwell and A. A. Weekes, Curr. Med. Chem., 2009, 16, 2430 CrossRef;
(e) M. C. V. Zandt, M. L. Jones, D. E. Gunn, L. S. Geraci, J. H. Jones, D. R. Sawicki, J. Sredy, J. L. Jacot, A. T. DiCioccio, T. Petrova, A. Mitschler and A. D. Podjarny, J. Med. Chem., 2005, 48, 3141 CrossRef PubMed;
(f) W. A. Skinner, F. Gualtiere, G. Brody and A. H. Fieldsteel, J. Med. Chem., 1971, 14, 546 CrossRef CAS.
- S. Rudrawar, A. Kondaskar and A. K. Chakraborti, Synthesis, 2005, 15, 2521 Search PubMed.
-
(a) H. Sharghi and O. Asemani, Synth. Commun., 2009, 39, 860 CrossRef CAS;
(b) X. Wen, J. El Bakali, R. Deprez-Poulain and B. Deprez, Tetrahedron Lett., 2012, 53, 2440 CrossRef CAS.
-
(a) X. Fan, Y. He, Y. Wang, Z. Xue, X. Zhang and J. Wang, Tetrahedron Lett., 2011, 52, 899 CrossRef CAS;
(b) R. Shelkar, S. Sarode and J. Nagarkar, Tetrahedron Lett., 2013, 54, 6986 CrossRef CAS.
-
(a) M. S. Mayo, X. Yu, X. Zhou, X. Feng, Y. Yamamoto and M. Bao, Org. Lett., 2014, 16, 764 CrossRef CAS PubMed;
(b) M. M. Shareef, X. Yu, X. Zhou, X. Feng, Y. Yamamoto and M. Bao, J. Org. Chem., 2014, 79, 6310 CrossRef PubMed.
- A. Khalafi-Nezhad and F. Panahi, ACS Catal., 2014, 4, 1686 CrossRef CAS.
-
(a) Y. Sun, H. Jiang, W. Wu, W. Zeng and X. Wu, Org. Lett., 2013, 15, 1598 CrossRef CAS PubMed;
(b) H. Tan, C.-X. Pan, Y.-L. Xu, H.-S. Wang and Y.-M. Pan, J. Chem. Res., 2012, 36, 370 CrossRef CAS.
- T. B. Nguyen, L. Ermolenko, W. A. Dean and A. Al-Mourabit, Org. Lett., 2012, 14, 5948 CrossRef CAS PubMed.
- L. J. Gu, C. Jin, J. M. Guo, L. Z. Zhang and W. Wang, Chem. Commun., 2013, 49, 10968 RSC.
- L. Ackermann, S. Barfüsser, C. Kornhaass and A. R. Kapdi, Org. Lett., 2011, 13, 3082 CrossRef CAS PubMed.
- T. Mukai, K. Hirano, T. Satoh and M. Miura, Org. Lett., 2010, 12, 1360 CrossRef CAS PubMed.
- Y. Liao, H. Qi, S. Chen, P. Jiang, W. Zhou and G. J. Deng, Org. Lett., 2012, 14, 6004 CrossRef CAS PubMed.
-
(a) Y. P. Zhu, F. C. Jia, M. C. Liu and A. X. Wu, Org. Lett., 2012, 14, 4414 CrossRef CAS PubMed;
(b) Y. P. Zhu, M. Lian, F. C. Jia, M. C. Liu, J. J. Yuan, Q. H. Gao and A. X. Wu, Chem. Commun., 2012, 48, 9086 RSC.
-
(a) G. Sakata, K. Makino and Y. Kurasawa, Heterocycles, 1988, 27, 2481 CrossRef CAS;
(b) A. Dell, D. H. Williams, H. R. Morris, G. A. Smith, J. Feeney and G. C. K. Roberts, J. Am. Chem. Soc., 1975, 97, 2497 CrossRef CAS PubMed;
(c) L. E. Seitz, W. J. Suling and R. C. Reynolds, J. Med. Chem., 2002, 45, 5604 CrossRef CAS PubMed.
-
(a) Z. Zhao, D. D. Wisnoski, S. E. Wolkenberg, W. H. Leister, Y. Wang and C. W. Lindsley, Tetrahedron Lett., 2004, 45, 4873 CrossRef CAS;
(b) M. Lian, Q. Li, Y. Zhu, G. Yin and A. Wu, Tetrahedron, 2012, 68, 9598 CrossRef CAS.
-
(a) S. Y. Kim, K. H. Park and Y. K. Chung, Chem. Commun., 2005, 1321 RSC;
(b) V. Jeena and R. S. Robinson, Beilstein J. Org. Chem., 2009, 5, 24 Search PubMed;
(c) A. Shaabani and A. Maleki, Chem. Pharm. Bull., 2008, 56, 79 CrossRef CAS PubMed.
-
(a) L. Nagarapu, R. Mallepalli, G. Arava and L. Yeramanchi, Chem.–Eur. J., 2010, 1, 228 CrossRef CAS;
(b) A. Kumar, A. Verma, G. Chawla and Vaishali, Int. J. ChemTech Res., 2009, 1, 1177 CAS.
- C. S. Cho and S. G. Oh, Tetrahedron
Lett., 2006, 47, 5633 CrossRef CAS.
- L. J. Martin, A. L. Marzinzik, S. V. Ley and I. R. Baxendale, Org. Lett., 2011, 13, 320 CrossRef CAS PubMed.
-
(a) S. Chandrasekhar, N. K. Reddy and V. P. Kumar, Tetrahedron Lett., 2010, 51, 3623 CrossRef CAS;
(b) W. Wang, Y. Shen, X. Meng, M. Zhao, Y. Chen and B. Chen, Org. Lett., 2011, 13, 4514 CrossRef CAS PubMed;
(c) K. S. Vadagaonkar, H. P. Kalmode, K. Murugan and A. C. Chaskar, RSC Adv., 2015, 5, 5580 RSC.
- T. B. Nguyen, P. Retailleau and A. Al-Mourabit, Org. Lett., 2013, 15, 5238 CrossRef CAS PubMed.
-
(a) H. Ma, D. Li and W. Yu, Org. Lett., 2016, 18, 868 CrossRef CAS PubMed;
(b) A. Sagar, S. Vidaycharan, A. H. Shinde and D. S. Sharada, Org. Biomol. Chem., 2016, 14, 4018 RSC;
(c) T. Chen, X. Chen, J. Wei, D. Lin, Y. Xie and W. Zeng, Org. Lett., 2016, 18, 2078 CrossRef CAS PubMed.
-
(a) T. Guntreddi, R. Vanjari and K. N. Singh, Org. Lett., 2014, 16, 3624 CrossRef CAS PubMed;
(b) R. Vanjari, T. Guntreddi, S. Kumar and K. N. Singh, Chem. Commun., 2015, 51, 366 RSC;
(c) T. Guntreddi, R. Vanjari and K. N. Singh, Org. Lett., 2015, 17, 976 CrossRef CAS PubMed.
-
(a) R. Vanjari, T. Guntreddi and K. N. Singh, Org. Lett., 2013, 15, 4908 CrossRef CAS PubMed;
(b) R. Singh, B. K. Allam, N. Singh, K. Kumari, S. K. Singh and K. N. Singh, Org. Lett., 2015, 17, 2656 CrossRef CAS PubMed;
(c) R. Vanjari, T. Guntreddi and K. N. Singh, Chem.–Asian J., 2016, 11, 696 CrossRef CAS PubMed.
-
(a) J. H. Poupaert, S. Duarte, E. Colacino, P. Depreux, C. R. McCurdy and D. L. Lambert, Phosphorus, Sulfur Silicon Relat. Elem., 2004, 179, 1959 CrossRef CAS;
(b) L. D. Priebbenow and C. Bolm, Chem. Soc. Rev., 2013, 42, 7870 RSC.
Footnote |
† Electronic supplementary information (ESI) available. See DOI: 10.1039/c6ra17491d |
|
This journal is © The Royal Society of Chemistry 2016 |
Click here to see how this site uses Cookies. View our privacy policy here.