DOI:
10.1039/C6RA17091A
(Paper)
RSC Adv., 2016,
6, 93694-93706
Modulating the release of Escherichia coli in double W1/O/W2 emulsion globules under hypo-osmotic pressure†
Received
4th July 2016
, Accepted 26th September 2016
First published on 27th September 2016
Abstract
Double water-in-oil-in-water (W1/O/W2) emulsions have the potential to encapsulate bacteria and their structure if destabilised can facilitate bacterial release in a time controlled manner. The description of release mechanisms of bacteria in W1/O/W2 emulsions is limited to hyper-osmotic conditions; however, the mechanism responsible for bacterial release in hypo-osmotic conditions is different and the presence of bacteria may affect the emulsion and vice versa. In this work, the stability and release properties of W1/O/W2 emulsions were studied with or without the presence of GFP-tagged Escherichia coli (E. coli-GFP) in the inner aqueous phase (W1). The impact of altering the osmotic balance by diluting the emulsion in a hypo-osmotic solution was also determined. W1/O/W2 emulsion preparation and E. coli-GFP encapsulation was achieved using a two-step homogenisation process and structure was changed by altering the concentration of hydrophilic surfactant (Tween80, 0.5–10%), lipophilic surfactant (PGPR, 1–8%) and W1 (20% and 40%). The release of E. coli-GFP was monitored by culture and observed using fluorescence microscopy. The release of E. coli-GFP was significantly (P < 0.05) increased when the osmotic balance was altered and at high amounts of W1 and low concentrations of Tween80 or PGPR. Bacterial release occurred due to oil globule bursting and the viability of bacteria was unaffected by the release mechanism. The concentration of bacteria affected the double emulsion structure during the first step of emulsification but had no effect on emulsion structure during osmotic balance alteration.
1. Introduction
Water-in-oil-in-water (W1/O/W2) emulsions are systems created by dispersing a water-in-oil (W1/O) emulsion in a second outer aqueous phase (W2).1 In recent years W1/O/W2 emulsions have gained a lot of interest as they have the ability to encapsulate and release hydrophilic substances in a time controlled manner making them promising for drug delivery, cosmetics and food products.2
The purpose of encapsulating microbial species in W1/O/W2 emulsions varies widely from trying to protect bacteria such as Lactobacillus spp. probiotics from gastric juices,3,4 bile salts,5 prolonged chilled storage6 and during cheese manufacturing and melting7 to utilising them as a 3D microenvironment for biofilm formation8 and as analytical tools for cultivation, genetic activation and quorum sensing.9 In such applications the formulation of a highly stable W1/O/W2 emulsion is desirable. However, W1/O/W2 emulsions are kinetically unstable due to the presence of two thermodynamically unstable interfaces10 and their stability requires a balance between the Laplace and osmotic pressures.11,12 By destabilising their structure release of hydrophilic compounds is possible and this can either be thermally-induced13,14 or osmotically-induced.15
The alteration of osmotic pressure in W1/O/W2 emulsion will destabilise the structure of the emulsion and can either lead to swelling or shrinkage of the oil globules depending on whether the concentration of solutes is higher in W1 or W2. Manipulating the osmotic pressure in W1/O/W2 emulsions can be used for delivering microbial species in vitro or in vivo which have great potential for applications within the fermentation, agricultural and medical industries. Recently we have reported the mechanism by which Escherichia coli is released from W1/O/W2 emulsion globules in the presence of NaCl in W2 and we found that by changing the emulsion structure this mechanism can be controlled.16 However, there is a lack of studies investigating the release of bacteria from the W1 phase of W1/O/W2 emulsions in hypo-osmotic conditions which as opposed to hyper-osmotically triggered release formulations provides a sustained release of salts as well as bacterial cells over a period of time in a controlled manner which is desirable in certain applications.
It was shown that by diluting W1/O/W2 emulsions in hypo-osmotic solution they swell and breakdown to release antiseptics.17 The concentration of the lipophilic (in the oil phase stabilising the W1/O interface) and hydrophilic (in the W2 phase stabilising the O/W2 interface) surfactants play a major role in controlling the release of the water-soluble substances from the W1 phase of W1/O/W2 emulsion globules under hypo-osmotic pressure.18,19 When the W1 volume fraction was increased the release of solutes from the oil globules in hypo-osmotic solution become higher.15 Also in a hypo-osmotic solution the release of solutes from W1/O/W2 globules was higher when the osmotic pressure gradient between the W1 and W2 phases was increased.15,18,19 However, in these studies the mechanism behind the release has not been clear and was described as “thinning of the oily phase” until breakdown of the oil globules occured.20 Furthermore, it is difficult to relate the release of water-soluble substances to one mechanism and many processes can be involved including:
• coalescence of the W1 droplets with O/W2 interface,21
• rupture of the O/W2 interfacial film,18
• migration of the water and water-soluble substances through the oil phase via surfactant-facilitated transport through reverse micelles,22 hydrated surfactant and/or diffusion through thin surfactant lamellae.23
We have recently shown that the release of bacteria from W1/O/W2 globules under hyper-osmotic pressure resulted from bursting of the oil globules independent of diffusion mechanisms.16 Moreover, investigating the mechanism of release by swelling using insoluble particles such as bacteria as opposed to water-soluble substances in W1 is advantageous when trying to understand how release occurs during the swelling process of the W1/O/W2 globules under hypo-osmotic pressure. This is because the release of water-soluble substances from W1/O/W2 globules can be the result of various mechanisms whereas bacterial release will only result from the rupturing of the interfacial film and bursting of the oil globules. We therefore need to investigate the mechanism responsible for the release of bacteria from W1/O/W2 globules in a hypo-osmotic solution and subsequently try to control this mechanism by changing the structure of the W1/O/W2 emulsion.
Bacteria can either destabilise24,25 or stabilise26,27 the structure of a single emulsion. Bacterial cells possess physiochemical surface properties which are determined by the chemistry of the cell surface important in cell attachment to surfaces.24 Moreover these properties can have an impact on the stability of an emulsion. For example, bacteria that have surface charge opposite to the oil globules were shown to localise at the O/W2 interface of the oil globules destabilising the structure of the emulsion.26 On the other hand bacteria were shown to prevent coalescence of the oil globules and increase the stability of the emulsion.26,27 Also the structure of emulsion can affect the growth of bacteria. For example, pathogenic E. coli 0157:H7 cells grown in emulsions as opposed to liquid media produced more curli (surface structures involved in bacterial attachment to surfaces) and were more heat resistant.28 Also it was shown that Gram negative and Gram positive bacteria were constrained to grow as colonies instead of as single cells when the volume of the oil phase was increased29 and when the oil globule size was small.30 When encapsulated in water droplets of a single W/O emulsion microbial cells can excrete waste products leading to osmotic mismatch that causes droplet shrinkage at rates depending on the strain, species and number of the microbial cells.31 Moreover, all these studies involved single emulsions and there is a lack in studies investigating the stability of double emulsions with bacteria.
By altering the osmotic balance between the W1 and W2 phases the stability and physical properties of the W1/O/W2 emulsion change. Deliberate destabilisation of W1/O/W2 emulsions is desirable in certain applications and if controlled can be useful for the release of water-soluble substances from the W1 phase at the desired time. Thus, W1/O/W2 emulsion oil globules need to be sufficiently stable so that the encapsulated water-soluble substances stay within the W1 phase but at the same time breakable so that release can be triggered.15 However, there is a lack of studies investigating how insoluble particles such as bacteria can be delivered from the inner to the outer phase of W1/O/W2 emulsions in hypo-osmotic conditions. In this study, the effect of varying the structure of W1/O/W2 emulsion on the release of E. coli from the oil globules facilitated by osmotic pressure was investigated and the mechanism of this release was microscopically visualised. Also the amount of inner W1 phase, hydrophilic surfactant (in W2 phase) and lipophilic surfactant (in oil phase) were changed and their effect on W1/O/W2 emulsion stability in the presence of GFP-tagged E. coli was analysed. Furthermore, we investigated the effects of encapsulation, release, prolonged storage and chemistry of emulsion on E. coli. We also studied the effect of the presence of E. coli (in W1 phase) on stability of the double emulsion and the encapsulation efficiency of the bacteria was monitored during storage.
2. Experimental
2.1. Materials
The water soluble emulsifier polysorbate 80 (Tween80) was purchased from Sigma-Aldrich (United Kingdom). The oil soluble emulsifier polyglycerol polyricinoleate (PGPR) was provided by Danisco (Denmark). Sunflower oil (food grade) was purchased from a local retailer (United Kingdom). Sodium chloride (NaCl) 99% was purchased from Sigma-Aldrich (Germany). The two stains 2-(4-amidinophenyl)-1H-indole-6-carboxamidine (DAPI) and bis-(1,3-dibutylbarbituric acid) trimethaine oxanol [DiBAC4 (3)] were purchased from Sigma-Aldrich (United Kingdom). Nucleopore black polycarbonate membrane (13 mm diameter; 2.0 μm pore size) and stainless steel 13 mm Swinney filter holder was purchased from Millipore (United Kingdom). Tryptic soy agar (Oxoid Ltd. CM0131) and broth (Oxoid Ltd. CM0129) were purchased from Fisher Scientific (United Kingdom).
2.2. Microbial cultures
Escherichia coli strain K-12, MG1655 (ATCC 47076) and its derivative E. coli SCC1 (MG1655 PA1/04/03-gfpmut3*, expressing green fluorescent protein (GFP) from the chromosome and subsequently referred to as E. coli-GFP)32 were maintained on tryptic soy agar Petri dishes at 4 °C. For obtaining cells in the exponential phase cells were harvested by centrifugation (10
000g, 10 minutes) and washed in de-ionised water twice. Cells were re-suspended in 10 ml of de-ionised water or NaCl solution and used for encapsulation.
2.3. Rheological measurements
Rheological characterisation of emulsion separate components was done by measuring the viscosity of the oil phase without or with varying concentrations of PGPR (2%, 4%, 6% or 8%), W2 phase without or with varying concentrations of Tween80 (0.5%, 1%, 5% or 10%) and W1/O emulsion with different W1 volume fraction (20% or 40% W1) in the presence of E. coli-GFP was performed at 25 °C using AR-G2 rheometer (TA instruments, New Castle, Delaware USA) on a parallel plate geometry (diameter 40 mm). The apparent viscosity was measured over a shear rate 0.1–100 s−1.
2.4. Preparation of W1/O/W2 emulsions
W1/O/W2 emulsions were prepared using a high shear mixer homogeniser (Silverson L5M) at room temperature using a two-step emulsification (Fig. S1†). In the first step primary W1/O emulsions were made. An oil phase was prepared by dissolving PGPR (1%, 2%, 4%, 6% or 8 wt%) in sunflower oil. The inner aqueous phase (W1) consisting of de-ionised water was emulsified (120 seconds) into the oil phase (W1
:
O phase ratio of 20
:
80 or 40
:
60). For microbial-encapsulation de-ionised water or NaCl solution (final concentration of 0.02 M, 0.04 M, 0.085 M or 0.17 M) containing the washed E. coli cells (5 × 107 CFU ml−1) was used as W1. The rotational speeds for homogenising the different formulations of primary W1/O and W1/O/W2 emulsions are shown in Table S1.† In the second step a W1/O/W2 emulsion was made. The outer aqueous phase (W2) was prepared by dissolving Tween80 (0.5%, 1%, 5% or 10 wt%) in de-ionised water at 60 °C for approximately 15 minutes. The previously prepared primary W1/O emulsion was emulsified (60 seconds) into W2 to form the W1/O/W2 emulsion (W1/O
:
W2 ratio of 20
:
80). From a volume of 50 ml W1/O/W2 emulsion the cream layer containing the oil globules was separated using a syringe and the serum phase was discarded. The cream layer was transferred into a sterile 500 ml conical flask containing Tween80 (0.5%, 1%, 5% or 10 wt%) dissolved in either de-ionised water or NaCl solution (final concentration of 0.02 M, 0.04 M, 0.085 M or 0.17 M) and incubated at 25 °C for 45 minutes or over a 180 minute period shaking at 100 rpm to ensure homogenised mixing.
2.5. Characterisation of W1/O/W2 emulsion stability
Measurement of oil globule size of double W1/O/W2 emulsions. The particle size distribution of oil globules in the W1/O/W2 emulsion was measured immediately after preparation and as a function of storage time using a laser diffraction particle size analyser (Malvern Mastersizer 2000, Malvern Instrument Ltd, Worcestershire, UK), equipped with a He–Ne laser (λ = 633 nm). The conditions of analysis conducted are described in ESI Methods S1.†
Observation of phase separation. The cream height fraction of the W1/O/W2 emulsions was measured immediately after preparation and as a function of storage time in accordance to our previous method.16
2.6. Microscopic observation of the W1/O/W2 emulsions
Video time-lapse for observation of oil globule bursting and tracking of E. coli-GFP. For video microscopy the sample was placed on a microscope slide and the video was recorded under objective lens 40× magnification with a Moticam 10 camera via Motic Images Plus video acquisition software at 17 fps. The light source used to excite the GFP was a light-emitting diode (LED) and the emission was observed at 509 nm.
Fluorescent and optical imaging of the W1/O/W2 emulsions and E. coli-GFP. The double W1/O/W2 emulsions with or without E. coli-GFP was observed using optical and fluorescent microscopy (Zeiss Axioplan) at room temperature as described in ESI Methods S2.†
2.7. Quantifying the release of E. coli-GFP during storage
The number of E. coli cells in the serum phase was measured immediately after preparation and as a function of storage time. Five millilitre sample of W1/O/W2 emulsion was collected and the serum phase was removed using a syringe. E. coli cell counts were made using serial dilutions in PBS (phosphate buffered saline) buffer solution and plating on tryptic soy agar using the Miles & Misra technique.33 Colony forming units per millilitre (CFU ml−1) were then calculated. As the serum phase contains no oil globules only unencapsulated (or those released when oil globules burst) viable bacteria were able to grow to colony forming units which could be counted.
2.8. Determining the total cell count and health of E. coli (MG1655) after encapsulation and release using epifluorescence microscopy
E. coli MG1655 in serum phase or de-ionised water (control) was stained by adding DAPI (4 μl ml−1) and [DiBAC4 (3)] (50 ng ml−1) and incubated in the dark for 30 minutes. The serum phase was injected through a nucleopore black polycarbonate membrane filter with 0.2 μm pore size and 13 mm diameter placed inside a Swinnex filter holder (Millipore) and the cells were counted using a fluorescent microscope (Zeiss Axiolab). The procedure is described in detail in ESI Methods S3.†
2.9. Determining the viability of E. coli (MG1655) and E. coli-GFP during incubation
E. coli cell counts were made immediately after preparation and as a function of storage time using serial dilutions in PBS (phosphate buffered saline) buffer solution and plating on tryptic soy agar using the Miles & Misra technique.
2.10. Encapsulation efficiency (%) measurements
The encapsulation efficiency and release of E. coli-GFP were calculated by the following equations:
Encapsulation efficiency = ((N0 − N)/N0) × 100% |
Release = (log10 N − log10 NT) |
where N is the number of unencapsulated viable bacterial cells immediately after forming the W1/O/W2 emulsion, N0 displays the free viable bacterial cells before encapsulation and NT displays the unencapsulated viable bacterial cells after incubation period.
2.11. Statistical analysis
Each experiment was conducted in triplicate (N = 3). The generated results were collected in Excel (Microsoft Corp.) for calculating means, standard deviations and error bars. For student's t-test to compare two means or one-way analysis of variance (ANOVA) and the Tukey's HSD post hoc test to compare several means were used for checking whether there is significant difference among samples using IBM SPSS Statistics software version 21. Differences were considered significant at P < 0.05.
3. Results
3.1. Primary W1/O emulsion characterization
The size of the W1 droplets can influence the stability of W1/O/W2 emulsions.34 In this study we ensured that the homogenisation conditions were such that the average mean size distribution [D(4, 3)] of W1 droplets was comparable (3–5 μm; Fig. S2 and S3†) in all primary W1/O formulations. E. coli-GFP was successfully encapsulated within the W1 droplets of the primary W1/O emulsion (Fig. S4†). When the primary W1/O emulsion contained 40% W1 and 2% PGPR, the presence of a high concentration of E. coli-GFP (109 CFU ml−1) prevented emulsion at 40% W1 and 2% PGPR W1/O emulsion to form; however, primary W1/O emulsions were able to form at low concentration of E. coli-GFP (108 CFU ml−1) (Fig. 1A). Again the formation of primary W1/O emulsion did not occur at a high concentration of E. coli-GFP (109 CFU ml−1) with 40% W1 but was able to form at 20% W1 (Fig. 1B). When the PGPR concentration was reduced to 1%, the W1/O emulsion did not form at low concentration of E. coli-GFP (108 CFU ml−1) but was able to form without the presence of E. coli-GFP (Fig. 1C). To find out whether this phenomenon was due to the metabolic activity of bacteria, dead E. coli-GFP cells (109 CFU ml−1) were added to 40% W1 and homogenised with the oil phase containing 2% PGPR. In the presence of dead E. coli-GFP cells the formation of primary W1/O emulsion did not occur at 109 CFU ml−1 but were able to form at 108 CFU ml−1 (Fig. 1D) showing that metabolic activity plays no role in destabilising the primary W1/O emulsion. These results suggest that the presence of E. coli cells (live or dead) within the W1 phase affects the formation of the primary W1/O emulsion during the first step of the homogenisation process and this depends on the concentration of E. coli-GFP and/or PGPR.
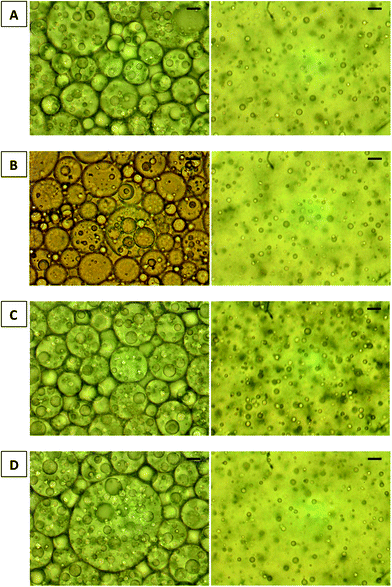 |
| Fig. 1 Single W1/O emulsions prepared with different concentrations of PGPR or concentrations of E. coli-GFP. The W1/O emulsions were prepared with inner-phase (W1) volume percentage of 40% or 20% containing E. coli-GFP and stabilised with PGPR. The formulations were as follows: (A) 2% PGPR containing live cells at 109 CFU ml−1 in 40% W1 (left) and 108 CFU ml−1 in 40% W1 (right); (B) 2% PGPR containing live cells at 109 CFU ml−1 in 40% W1 (left) and in 20% W1 (right); (C) 1% PGPR containing live cells at 108 CFU ml−1 in 40% W1 (left) and no E. coli-GFP in 40% W1 (right); (D) 2% PGPR containing dead cells at 109 CFU ml−1 in 40% W1 (left) and 108 CFU ml−1 in 40% W1 (right); Scale bar: 10 μm. | |
3.2. Influence of osmotic balance alteration on release of E. coli-GFP
To understand how the release of bacteria during osmotic pressure alteration is affected by varying the W1/O/W2 emulsion composition, the release of E. coli-GFP over time was quantified while altering the osmotic pressure gradient (Fig. 2A), the concentration of Tween80 (Fig. 2B), PGPR (Fig. 2C) and the W1 phase (Fig. 3A and B).
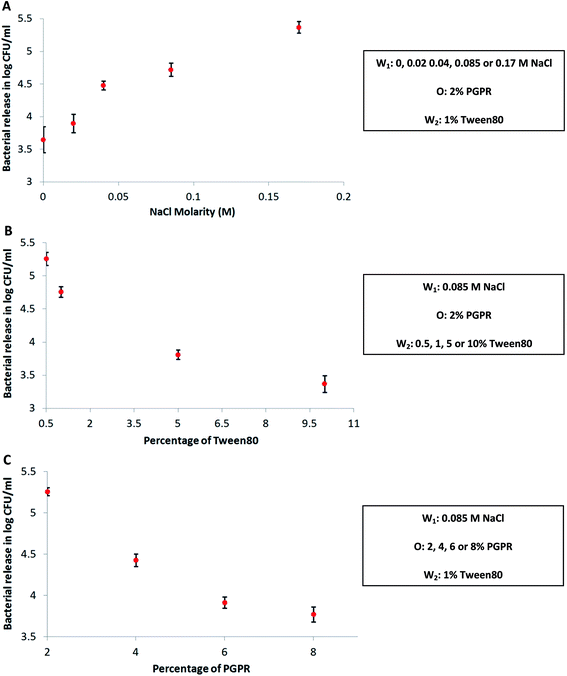 |
| Fig. 2 Effect of varying the structure of the W1/O/W2 emulsion on the release of E. coli-GFP. The amount of released bacteria in the outer W2 phase of the W1/O/W2 emulsions was measured after 45 minutes incubation at 25 °C was quantified. The W1/O/W2 emulsions were prepared: (A) 40% W1 containing E. coli-GFP and varying concentrations of NaCl (0.02 M, 0.04 M, 0.085 M or 0.17 M) in W1 and stabilised with 1% Tween80 in W2 and 2% PGPR; (B) 40% W1 containing E. coli-GFP and 0.085 M NaCl in W1 and stabilised with varying concentrations of Tween80 (0.5%, 1%, 5% or 10%) in W2 and 2% PGPR in the oil phase; (C) 40% W1 containing E. coli-GFP and 0.085 M NaCl in W1 and stabilised with 1% Tween80 in W2 and varying concentrations of PGPR (2%, 4%, 6% or 8%) in the oil phase. Bars represent mean ± SEM taken from a minimum of 3 independent experiments. The data was analysed with one-way ANOVA. | |
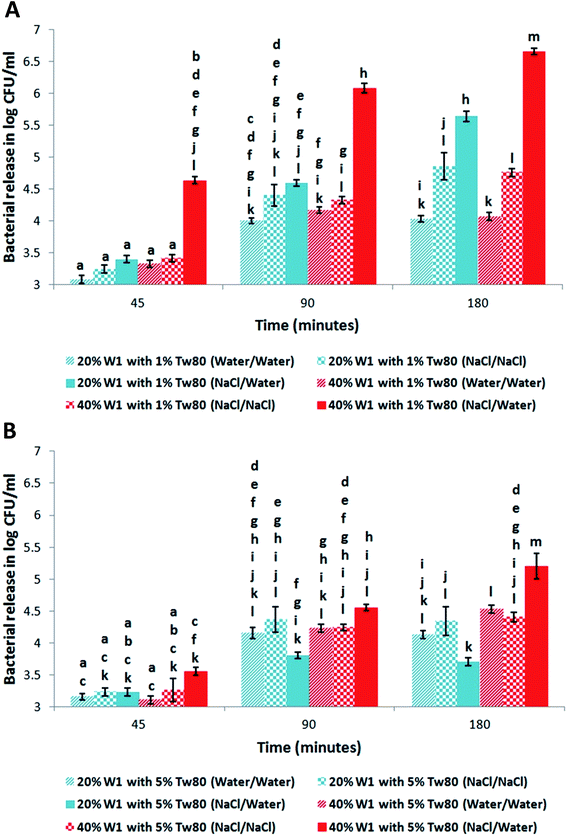 |
| Fig. 3 Effect of varying the structure of the W1/O/W2 emulsion on the release of E. coli-GFP. The amount of released bacteria in the outer W2 phase of the W1/O/W2 emulsions after 45, 90 and 180 minutes incubation at 25 °C was quantified. The W1/O/W2 emulsions were prepared with different inner W1 phase volume percentage of 20% or 40% containing E. coli-GFP with or without 0.085 M NaCl in W1 or W2 and stabilized with 1% (A) or 5% (B) Tween80 in W2 and 2% PGPR in the oil phase. Bars represent mean ± SEM taken from a minimum of 3 independent experiments. Mean values with different letters are significantly different (P < 0.05). The data was analysed with one-way ANOVA. | |
With the formulation containing 40% W1 and stabilised with 1% Tween80 the release of E. coli-GFP was significantly (P < 0.05) increased when osmotic balance was altered compared to when unaltered (Fig. 2A). The release of E. coli-GFP was significantly (P < 0.05) increased at higher (0.17 M and 0.085 M) compared to lower concentrations (0.04 M and 0.02 M) of NaCl (Fig. 2A). During osmotic balance alteration the release of E. coli-GFP was significantly (P < 0.05) higher at low concentrations of Tween80 (0.5% and 1%) compared to high concentrations of Tween80 (5% and 10%) (Fig. 2B). During osmotic balance alteration the release of E. coli-GFP was significantly (P < 0.05) higher at low concentrations of PGPR (2% and 4%) compared to high concentrations of PGPR (6% and 8%) (Fig. 2C).
The effect of varying the concentration of W1 at 1% and 5% Tween80 on the release of E. coli-GFP with and without altering the osmotic balance over time was quantified (Fig. 3A and B respectively). At 20% W1 and 1% Tween80 there was no significant difference in release of E. coli-GFP when osmotic balance was altered compared when unaltered after 45 and 90 minutes but became significantly (P < 0.05) higher after 180 minutes (Fig. 3A). However, at 40% W1 and 1% Tween80 the release of E. coli-GFP was significantly higher when the osmotic balance was altered compared to unaltered after 45, 90 and 180 minutes. Also at 1% Tween80 the release of E. coli-GFP was significantly (P < 0.05) higher at 40% W1 compared to 20% W1 after 45, 90 and 180 minutes (Fig. 3B). In contrast, at 40% W1 and 5% Tween80 there was no significant difference in release of E. coli-GFP when osmotic balance was altered compared to unaltered after 45 and 90 minutes but was significantly (P < 0.05) higher after 180 minutes. Also there was no significant difference in release of E. coli-GFP at 20% W1 and 5% Tween80 when osmotic balance was altered compared to unaltered after 45, 90 and 180 minutes. Also at 5% Tween80 the release of E. coli-GFP was significantly (P < 0.05) higher at 40% W1 compared to 20% W1 after 90 and 180 minutes. These results suggest that the release of E. coli-GFP is affected by the concentration of NaCl, Tween80 and PGPR during osmotic balance alteration.
3.3. Changes in oil globule size during osmotic balance alteration and storage
To understand how the stability of W1/O/W2 globules encapsulating E. coli-GFP is affected during osmotic pressure alteration we measured the change in oil globule size (D(4, 3)) over time by varying the osmotic pressure gradient (Fig. 4A),changing the concentration of Tween80 (Fig. 4B) and PGPR (Fig. 4C). The effect of varying the concentration of W1 and Tween80 on the size (D(4, 3)) of the oil globules with and without altering the osmotic balance in the presence or absence of E. coli-GFP in W1 (Tables 1 and S2†) was measured.
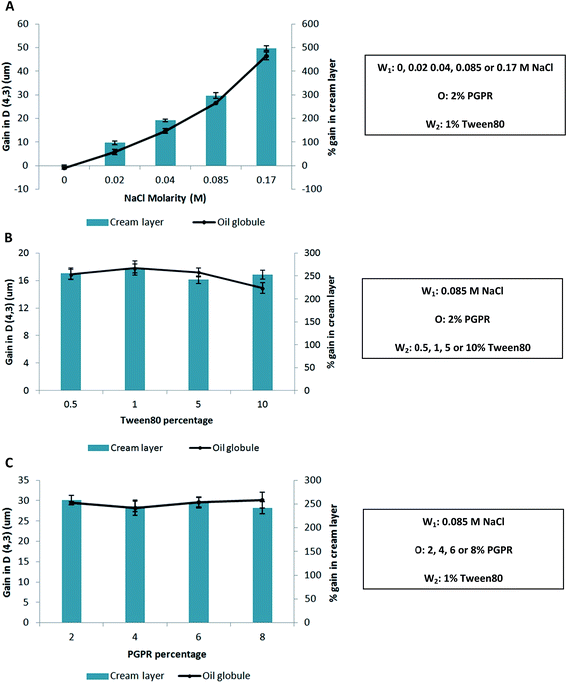 |
| Fig. 4 Reduction in mean oil globule diameter size (μm) by light scattering [D(4, 3)] and percentage gain in cream layer thickness of W1/O/W2 emulsions in hypo-osmotic solution after 45 minutes relative to time 0 minutes incubation at 25 °C. The W1/O/W2 emulsions were prepared: (A) 40% W1 containing E. coli-GFP and varying concentrations of NaCl (0.02 M, 0.04 M, 0.085 M or 0.17 M) in W1 and stabilised with 1% Tween80 in W2 and 2% PGPR; (B) 40% W1 containing E. coli-GFP and 0.085 M NaCl in W1 and stabilised with varying concentrations of Tween80 (0.5%, 1%, 5% or 10%) in W2 and 2% PGPR in the oil phase; (C) 40% W1 containing E. coli-GFP and 0.085 M NaCl in W1 and stabilised with 1% Tween80 in W2 and varying concentrations of PGPR (2%, 4%, 6% or 8%) in the oil phase. Results are taken from a minimum of 3 independent experiments. The data was analysed with one-way ANOVA. | |
Table 1 Change in mean oil globule diameter size (μm) of W1/O/W2 emulsions during osmotic pressure alteration measured by light scattering [D(4, 3)] after 45, 90 and 180 minutes relative to time 0 minutes incubation at 25 °C. The W1/O/W2 emulsions were prepared with different inner-phase (W1) volume percentage of 20% or 40% in the presence or absence of E. coli-GFP with 0.085 M NaCl in the W1 phase and stabilised with 1% or 5% Tween80 in W2 and 2% PGPR in the oil phase. Results are taken from a minimum of 3 independent experimentsa
|
W1/O/W2 emulsion formulations |
45 minutes |
90 minutes |
180 minutes |
The data was analysed with one-way ANOVA. a,b,c,d,e,f means ± standard deviation with different letters are significantly different. |
No E. coli-GFP |
20% W1, 1% Tw80, 0.085 M NaCl in W1 only |
7.44 ± 0.4a |
13.66 ± 0.3a |
24.58 ± 1.2b |
20% W1, 5% Tw80, 0.085 M NaCl in W1 only |
9.6 ± 2.6a |
11.7±1a |
21.8 ± 0.3b |
40% W1, 1% Tw80, 0.085 M NaCl in W1 only |
22.6 ± 1.3b |
42.8 ± 2.5c |
50.94 ± 2.6d |
40% W1, 5% Tw80, 0.085 M NaCl in W1 only |
24.8 ± 0.7b |
40.72 ± 0.8c |
50.38 ± 1.6d |
With E. coli-GFP |
20% W1, 1% Tw80, 0.085 M NaCl in W1 only |
7.86 ± 1ab |
13.21 ± 1.4ab |
24.76±3c |
20% W1, 5% Tw80, 0.085 M NaCl in W1 only |
7.18 ± 0.7ab |
10.25 ± 1.3a |
21.11 ± 1.2c |
40% W1, 1% Tw80, 0.085 M NaCl in W1 only |
21.2 ± 1.1d |
37.2 ± 0.9e |
50.9 ± 2.6f |
40% W1, 5% Tw80, 0.085 M NaCl in W1 only |
19.9 ± 1.9bd |
35.9±2e |
47.9±2f |
The presence of bacteria in W1 had no effect on the D(4, 3) during the incubation period (Tables 1 and S4†). After alteration of the osmotic balance the D(4, 3) significantly (p < 0.05) increased (Fig. 4A and Table 1) over time. When the concentration of NaCl in W1 was high (0.085 M and 0.17 M) the increase in D(4, 3) was significantly (P < 0.05) higher compared to when the concentration of NaCl in W1 was low (0.02 M and 0.04 M) (Fig. 4A). During osmotic balance alteration there was no significant difference in D(4, 3) at different concentrations of Tween80 (Fig. 4B) or PGPR (Fig. 4C) but the D(4, 3) was significantly (p < 0.05) higher at 40% W1 compared to 20% W1 (Table 1). There was no significant difference in the D(4, 3) when the osmotic balance was unaltered in between W1 and W2 (Table S4†). These results indicate that the D(4, 3) is affected by the concentration of NaCl and W1 during osmotic balance alteration whilst the concentration of Tween80 and PGPR had no effect on D(4, 3).
The presence of E. coli-GFP in W1 had no effect on the change in D(4, 3) of W1/O/W2 emulsions during 7 days storage. There was no significant difference in the D(4, 3) with E. coli-GFP compared to without E. coli-GFP after 3 and 7 days (Table S3†).
3.4. Changes in creaming behaviour during osmotic balance alteration and storage
Percentage gain in cream layer thickness over time was measured in order to characterise the creaming behaviour of W1/O/W2 emulsions containing E. coli-GFP in W1 as an effect of varying the osmotic pressure gradient (Fig. 4A), concentration of Tween80 (Fig. 4B), and PGPR (Fig. 4C). The effect of varying the concentration of W1 and Tween80 on the percentage gain in cream layer thickness of the W1/O/W2 emulsions with and without altering the osmotic balance in the presence or absence of E. coli-GFP in W1 (Fig. 5A and B and Table S4†) was measured.
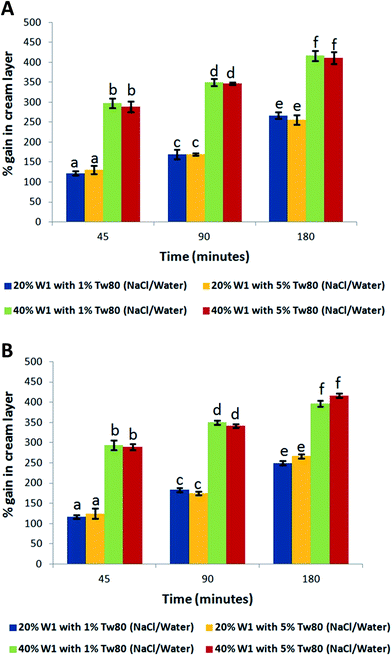 |
| Fig. 5 Change of percentage in cream layer thickness of W1/O/W2 emulsions during osmotic balance alteration after 45, 90 and 180 minutes relative to time 0 minutes incubation at 25 °C. The W1/O/W2 emulsions were prepared with different inner-phase (W1) volume percentage of 20% or 40% with (A) or without (B) E. coli-GFP with 0.085 M NaCl in the W1 phase stabilised with 1% or 5% Tween80 in W2 and 2% PGPR in the oil phase. Bars represent mean ± SEM taken from a minimum of 3 independent experiments. Mean values with different letters are significantly different (P < 0.05). The data was analysed with one-way ANOVA. | |
The presence of bacteria in W1 had no effect on the cream layer thickness during the incubation period (Fig. 5A, B and Table S4†). The percentage gain in cream layer thickness was significantly (P < 0.05) increased over time when the osmotic balance was altered compared to when unaltered (Fig. 4A). With the formulation containing 40% W1 and stabilised with 1% Tween80 and during osmotic balance alteration the gain in cream layer thickness was significantly (P < 0.05) increased when the concentration of NaCl in W1 was high (0.085 M and 0.17 M) compared to when the concentration of NaCl in W1 was low (0.02 M and 0.04 M) (Fig. 4A). During osmotic balance alteration there was no significant change in cream layer thickness at different concentrations of Tween80 (Fig. 4B) or PGPR (Fig. 4C). During no osmotic balance alteration and regardless of W1 and Tween80 concentration there were no significant changes in cream layer thickness (Table S4†). During osmotic balance alteration the gain in cream layer thickness was significantly (P < 0.05) higher at 40% W1 compared to 20% W1 regardless of Tween80 concentration (Fig. 5A and B). These results indicate that creaming behaviour is affected by the concentration of NaCl and W1 during osmotic balance alteration whilst the concentration of Tween80 and PGPR had no effect.
The presence of E. coli-GFP in W1 had no effect on the creaming behaviour of W1/O/W2 emulsions during 7 days storage. There was no significant difference on the cream layer thickness with E. coli-GFP compared to without E. coli-GFP after 3 and 7 days (Table S3†).
3.5. Microscopic observation of W1/O/W2 emulsions and E. coli-GFP release
The W1/O/W2 emulsions were observed using fluorescence microscopy to investigate the effect of altering the osmotic balance and changing the concentration of W1 and Tween80 on the structure of the W1/O/W2 emulsion and the release of E. coli-GFP from W1 to W2. After osmotic balance alteration an increase in size of the W1/O/W2 globules was observed as well as the presence of E. coli-GFP in both W1 and W2 phases. During osmotic balance alteration and at 40% and 1% Tween80 the oil globules showed swelling (Fig. S5A†) and E. coli-GFP cells were present in the W1 phase and also in the W2 phase (Fig. S6A†). During osmotic balance alteration and at 20% W1 and 1% Tween80 the oil globules showed swelling (Fig. S5B†) whilst E. coli-GFP cells were mainly present in the W1 phase with a few present in the W2 phase (Fig. S6B†). During no osmotic balance alteration the oil globules showed no swelling (Fig. S5C†) whilst E. coli-GFP cells mainly remained within the W1 phase (Fig. S6C†).
3.6. Effect of encapsulation and release on E. coli viability
To understand the effect of W1/O/W2 emulsion structure and the release mechanism on E. coli we studied the viability of bacteria by culture on media and microscopic assessment of bacterial cells stained for injury. There was no significant difference in the number of E. coli cells obtained from the plating method compared to microscopic enumeration (Fig. 6). Also after release into the W2 phase, there were no DiBAC4 (3) positive E. coli cells (Fig. S7†) suggesting that encapsulation and release had no effect on E. coli membrane potential.
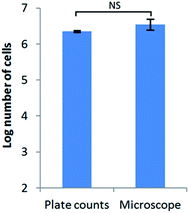 |
| Fig. 6 log number of released E. coli cells in serum phase quantified by plate counting and microscopic enumeration after 180 minutes incubation at 25 °C. The W1/O/W2 emulsion was made with 40% W1 with E. coli with 0.085 M NaCl in the W1 phase stabilised with 1% Tween80 in W2 and 2% PGPR in the oil phase. Bars represent mean ± SEM taken from a minimum of 3 independent experiments. The data was analysed with student's t-test. | |
4. Discussion
The stability of the primary W1/O emulsion at different concentrations of E. coli-GFP cells was studied with varying PGPR and W1 concentration. The primary W1/O emulsion did not form at high concentration of E. coli-GFP cells (109 CFU ml−1) when the concentration of W1 was high (40%) and PGPR was low (1%). Moreover, the same also occurred when the cells were dead showing that the disruption of W1/O emulsion formation was not due to any metabolic activity of the cells. It was also observed that when the W1/O emulsions were not able to form, multiple W/O/W/O emulsion morphology appeared. This type of emulsion was described as a super multiple emulsion35 and appeared as part of the emulsion morphology evolution during catastrophic phase inversion which occurs when one of formulation variables (e.g. surfactant affinity) or composition variables (e.g. oil/water ratio) is changed during the homogenising process.35 We believe that this occurred due to the lipophilic nature of PGPR allowing it to adsorb to the bacterial lipid membrane16 reducing its concentration within the oil phase. However, more investigation is required to understand how the presence of E. coli-GFP could lead to a super emulsion morphology formation.
In agreement with previous studies,36–38 we found that when the W1/O/W2 emulsion was diluted in a hypo-osmotic solution the oil globules became larger in size. We confirmed that this occurred not as a result of coalescence but due to actual migration water from W2 to W1 by following the evolution of a single oil globule in hypo-osmotic solution using microscopy (see ESI Results S1 and Fig. S8†). As water migrates from W2 to W1 the swelling of the oil globules diminishes because of the reduction in the concentration gradient. The swelling of the oil globules due to the presence of NaCl in the W2 phase was shown to be concentration dependent as the oil globules were larger at higher concentrations (0.085 M and 0.17 M) compared to lower concentrations of NaCl (0.04 M and 0.02 M). These findings correlate with a study by Chong-Kook et al. concluding that the oil layer of W1/O/W2 emulsion globules acts as a water-permeable membrane between the two aqueous phases of the W1/O/W2 emulsion under the osmotic pressure gradient.39 This phenomenon can be related to the osmotic pressure gradient created between the W2 and W1 phases during osmotic balance alteration.15 Thus, at concentrations of 0.085 M and 0.17 M NaCl in W2 an osmotic pressure of 42 atm and 84.2 atm is obtained respectively, and at concentrations of 0.02 M and 0.04 M NaCl in W2 an osmotic pressure of 10.52 atm and 21 atm is obtained respectively. It has been well demonstrated that water migration in W1/O/W2 emulsions occurs due to interfacial processes control the transport of water in W1/O/W2 emulsions rather than bulk diffusion.38 When the W1 droplets and oil globule interfaces are not physically in contact mechanisms that control the transport of water between W1 and W2 phases include spontaneous emulsification and reverse micellisation.37 Another mechanism controls water transport when the W1 and oil globule interfaces are physically in contact and that involves the hydration of the surfactant molecule at one interface, its diffusion through the oil phase to dehydrate at the other interface that is in contact with the phase of higher solute concentration.40 In this study we found that the concentrations of Tween80 (in the W2 phase) or PGPR (in the oil phase) had no significant effect on the oil globule size.
In the video-microscopy results (see ESI Results S2, Fig. S9 and Video S1†) we observed that after altering the osmotic balance with the formulation containing low concentration of Tween80 and high amount of the W1 phase the oil globule bursts releasing W1 droplets and E. coli-GFP into W2. We also observed that the W1 droplets persisted within the W2 phase upon release. In a recent study we have reported the same observation.16 However; more investigation is needed to understand the nature of these W1 droplets. In systems where the osmotic pressure was unaltered we found minimal release of E. coli-GFP probably due to mechanical breakdown (i.e. mixing) of the W1/O/W2 emulsion globules. The release of E. coli-GFP was higher when osmotic balance was altered compared to when unaltered which was NaCl concentration-dependent. During osmotic balance alteration and at low concentration of Tween80 release of E. coli cells from W1/O/W2 globules was increased regardless of W1 concentration. Jager-Lezer et al. found that when W1/O/W2 emulsions were diluted in a hypo-osmotic solution the W1 droplets increased in size leading to swelling of the oil globules until a critical size is reached.38 Moreover, the authors claim that beyond the critical size the oil globules will burst and release their inner contents into W2.
The mechanism of interfacial film rupture is determined by the characteristics of the continuous phase (e.g. viscosity) and the interfacial layers surrounding the oil globules (e.g. thickness, interfacial tension).41,42 Above a certain critical film thickness, film rupture of the oil globule is improbable.43 However, film rupturing becomes more probable once the film has thinned to the critical thickness.43 The thinner the film the lower the energy of activation required to form a hole that grows leading to film rupture and oil globule bursting.41 Tween80 molecules are able to keep the oil globules apart as well as provide a barrier against rupture. When present at higher concentrations in the W2 phase, hydrophilic surfactants tend to form multilayer arrangement of interdigitated surfactant chains that surrounds and “wraps” the oil globules44 resulting in an increased interfacial film strength.38 When Tween80 is present in W2 at high amounts it adsorbs at the O/W2 interface creating more condensed interfacial films which are able to resist hole creation and propagation that leads to film rupture.45 Gaps that are formed at the O/W2 interface due to incomplete surface coverage of the interface by the surfactant molecules or due to uneven distribution of surfactant molecules on the interface will lead to hole formation.46 If there are insufficient surfactant molecules in the system to saturate the O/W1 interface there will be gaps in the interfacial film.46 Also during swelling the interfaces of the oil globules are stretched and pressed against each other and as this happens the emulsifier molecules will be dragged along the interface leaving regions with more and regions with fewer surfactant molecules at the O/W2 interface.47 Moreover, if the adsorption of surfactant molecules to the O/W2 interface to cover these gaps is slow the interfacial film becomes more susceptible to rupture.46 During swelling of W1/O/W2 globules the expansion of the O/W2 interface results in decreased interfacial film strength and therefore making it more susceptible to rupture.18,19 We believe that as the interface expands the distance between the Tween80 molecules at the interface increases and gaps will form. Moreover, excess Tween80 molecules in W2 start to adsorb at the interface to fill up the free spaces caused by swelling and therefore maintain the O/W2 interface and prevent the oil globule from bursting. The adsorption of Tween80 at the interface occurs more rapidly when the excess Tween80 concentration in W2 is high which prevents or slows down the rupture of the interfacial film. In contrast when the concentration of excess Tween80 in W2 is low the adsorption of Tween80 at the interface is slower resulting in an expanding film that becomes progressively thinner and more susceptible to rupture. Also an increase in continuous phase viscosity increases the time required for the interfacial films to drain to their critical thickness and therefore prolong its resistance to rupture.41 The viscosity of the continuous phase was shown to increase when the amount of Tween80 was increased.48 However, at the concentrations used in our study we found that increasing the amount of Tween80 had no significant effect on viscosity of the continuous phase.
The release of E. coli-GFP was significantly higher at lower concentrations of PGPR. Geiger et al. found that by increasing the lipophilic surfactant concentration in the oil phase more rigidified O/W2 interface is formed measured as an increase in shear elastic modulus and interfacial tension.19 Furthermore, the authors suggested that an increase in lipophilic surfactant concentration increases the swelling capacity of the oil globule which delays breakdown and release of solutes. They argued that this occurred as a result of excess lipophilic surfactant in the oil phase diffusing to the O/W2 interface to fill up free spaces caused by the swelling.19 The viscosity of the oil phase will increase when more lipophilic surfactant is present in the oil phase.47 We found that the viscosity of the oil phase significantly (P < 0.05) increased at higher amounts of PGPR (see ESI Results S3 and Table S5†). Jiao et al. showed that by increasing the concentration of Span80 (a lipophilic surfactant) the viscosity of the oil phase increased resulting in the ability of the oil globules to resist deformation upon applying force (by placing a coverslip on top of the W1/O/W2 emulsion).49,50 Moreover, when present in excess in the oil phase, the lipophilic surfactant was found to increase the visco-elasticity of the interfacial film of the oil globule.51
With the formulation containing 20% W1 and 1% Tween80 There was no significant difference in the release of E. coli-GFP between the different W1/O/W2 emulsions when the osmotic balance was unaltered after 45 and 90 minutes, however the release became higher with compared to without NaCl in W2 after 180 minutes (Fig. 3A). NaCl can interact unfavourably with Tween80 and reduce the interfacial film strength of the W1/O/W2 globule50 and this was shown to be concentration dependent.52 In comparison to sodium salicylate that was added to the W2 phase of W1/O/W2 emulsions to alter the osmotic balance, NaCl led to a significant reduction in the strength of the interfacial film probably due to unfavourable interactions between NaCl and Tween80 and/or salting out.50
In addition to oil globule bursting other mechanisms may have resulted in the release of E. coli-GFP. We have recently shown that coalescence between oil globules does not result in release of E. coli-GFP.16 The coalescence of W1 droplets with the O/W2 interface may result in release of E. coli-GFP but this mechanism was shown to occur more frequently with increasing concentrations of Tween80 (ref. 21) which if was occurring in our study would have resulted in increased release of E. coli-GFP cells at high concentration of Tween80 but that would be in contrast to what we observed in this study. Further work is needed to find out if such mechanisms were responsible in the release of E. coli-GFP from the oil globules in hyper-osmotic solution.
We found that the release of E. coli-GFP significantly (P < 0.05) increased at high amount of W1. The release of solutes from W1/O/W2 emulsion globules in hypo-osmotic solution increased with higher W1 fraction.15 When the W1 volume is higher we found a significant increase in D(4, 3) and creaming thickness. Therefore, we believe that the interfacial film will be expanding at a faster rate when the W1 concentration is high compared to when it is low and this will lead to more gaps to form at the O/W2 interface which increase the chances of hole formation and propagation resulting in film rupture. We also found that the viscosity of the W1/O emulsion increases with higher amounts of W1 (see ESI Results S3 and Table S5†). According to the Mooney equation53,54 the increase in viscosity of W1/O/W2 emulsion globules at higher amounts of W1 has a destabilising effect on the oil globules making them more susceptible to rupture.49 However, the increase in W1/O/W2 emulsion globule viscosity due to higher amount of W1 phase has an opposite effect on emulsion stability than does the increase in the globule's viscosity due to higher amounts of lipophilic surfactant present in the oil phase.49
When water migrates from W2 to W1 the volume of the continuous phase is reduced which can be observed as an increase in cream layer height. The stability of the cream layer was not affected by the presence of E. coli-GFP within the W1/O/W2 emulsion globules. Also the presence of E. coli-GFP within the W1/O/W2 emulsion globules had no effect on the transport of water and/or the bursting of the oil globules. Moreover, the release mechanism had no effect on the viability of E. coli cells and there were no DiBAC4 (3) stained E. coli cells. Since DiBAC4 (3) is a membrane potential dye and will stain E. coli cells with a depolarised membrane55 our results indicate that no injury was caused to the E. coli cells after encapsulation and release from W1/O/W2 globules. The encapsulation efficiency of W1/O/W2 emulsions was significantly (P < 0.05) lower after 3 and 7 days of storage, however they still achieved high encapsulation efficiency (>90%) (see ESI Results S4 and Fig. S10†) and their viability was not affected (see ESI Results S5 and Fig. S11†) proving their suitability for industrial applications.
5. Conclusion
In this study the release of E. coli cells from W1/O/W2 emulsion globules under hypo-osmotic pressure was investigated. The release of bacteria from the oil globules of W1/O/W2 emulsion was due to the bursting of the oil globules and this release can be modulated by changing the structure of the W1/O/W2 emulsion. Globule bursting under hypo-osmotic pressure allows controlling the release of bacteria as well as hydrophilic compounds from the inner W1 phase. More investigation is required to understand the release mechanism such as monitoring interfacial film strength during osmotic balance alteration. Moreover, bacterial viability was not affected by the structure of the W1/O/W2 emulsion. We demonstrate the potential use of W1/O/W2 emulsion in microbiological applications as they show high stability and encapsulation efficiency for up to 7 days of storage. The possibility of modulating bacterial release from W1/O/W2 emulsion globules by changing its structure could be useful for fermentation industry to control and/or inhibit bacterial release. For example, a formulation can be intelligently designed containing oil globules encapsulating various different bacterial species and/or growth factors that can be sequentially released during a fermentation process.
Acknowledgements
Financial support from the School of Chemical Engineering, University of Birmingham is acknowledged. The authors would like to thank Mr Mohd Iqram Fauzi and Dr Gurpreet Birdi for technical assistance. PGPR was kindly provided by Danisco (Denmark). The authors are grateful to Prof Ian Norton and the Microstructure Engineering Research Group for sharing invaluable expertise and resources.
Notes and references
- N. Garti, Progress in stabilization and transport phenomena of double emulsions in food applications, LWT–Food Sci. Technol., 1997, 30, 222–235 CrossRef CAS.
- F. Jiménez-Colmenero, Potential applications of multiple emulsions in the development of healthy and functional foods, Food Res. Int., 2013, 52(1), 64–74 CrossRef.
- M. Shima, Y. Morita, M. Yamashit and S. Adachi, Protection of Lactobacillus acidophilus from the low pH of a model gastric juice by incorporation in a W/O/W emulsion, Food Hydrocolloids, 2006, 20, 1164–1169 CrossRef CAS.
- D. J. Pimentel-González, R. G. Campos-Montiel, C. Lobato-Calleros, R. Pedroza-Islas and E. J. Vernon-Carter, Encapsulation of Lactobacillus rhamnosus in double emulsions formulated with sweet whey as emulsifier and survival in simulated gastrointestinal conditions, Food Res. Int., 2009, 42, 292–297 CrossRef.
- M. Shima, T. Matsuo, M. Yamashita and S. Adachi, Protection of Lactobacillus acidophilus from bile salts in a model intestinal juice by incorporation into the inner-water phase of a W/O/W emulsion, Food Hydrocolloids, 2009, 23, 281–285 CrossRef CAS.
- X. Xin, C. Xiguang, L. Chengsheng, C. Dongsu and P. Hogni, Influence of Lactobacillus E1 on the storage stability in emulsion immobilization, J. Wuhan Univ. Technol., Mater. Sci. Ed., 2009, 24(1), 75–80 CrossRef.
- M. E. Rodríguez-Huezo, A. G. Estrada-Fernandez, B. E. García-Almendarez, F. Ludena-Urquizo, R. G. Campos-Montiel and D. J. Pimentel-Gonzalez, Viability of Lactobacillus plantarum entrapped in double emulsion during Oaxaca cheese manufacture, melting and simulated intestinal conditions, LWT–Food Sci. Technol., 2014, 59, 768–773 CrossRef.
- C. B. Chang, J. N. Wilking, S.-H. Kim, H. C. Shum and D. A. Weitz, Monodisperse emulsion drop microenvironments for bacterial biofilm growth, Small, 2015, 11, 3954–3961 CrossRef CAS PubMed.
- Y. Zhang, Y.-P. Ho, Y.-L. Chiu, H. F. Chan, B. Chlebina, T. Schuhmann, L. You and K. W. Leong, A programmable microenvironment for cellular studies via microfluidics-generated double emulsions, Biomaterials, 2013, 34, 4564–4572 CrossRef CAS PubMed.
- D. J. McClements, E. A. Decker, Y. Park and J. Weiss, Structural design principles for delivery of bioactive components in nutraceutical and functional foods, Crit. Rev. Food Sci. Nutr., 2009, 49, 577–606 CrossRef CAS PubMed.
- M. Kanouni, H. L. Rosano and N. Naouli, Preparation of a stable double emulsion (W1/O/W2): role of the interfacial films on the stability of the system, Adv. Colloid Interface Sci., 2002, 99, 229–254 CrossRef CAS PubMed.
- R. Mezzenga, B. M. Folmer and E. Hughes, Design of Double Emulsions by Osmotic Pressure Tailoring, Langmuir, 2004, 20, 3574–3582 CrossRef CAS PubMed.
- E. C. Rojas and K. D. Papadopoulos, Induction of instability in water-in-oil-in-water double emulsions by freeze-thaw cycling, Langmuir, 2008, 23, 6911–6917 CrossRef PubMed.
- E. C. Rojas, J. A. Staton, V. T. John and K. D. Papadopoulos, Temperature-induced protein release from water-in-oil-in-water double emulsions, Langmuir, 2008, 24, 7154–7160 CrossRef CAS PubMed.
- A. Cárdenas and E. Castro, Breaking of multiple emulsions under osmotic pressure and the effect of W1/O relation, Interciencia, 2003, 28(9), 534–538 Search PubMed.
- H. El Kadri, T. Overton, S. Bakalis and K. Gkatzionis, Understanding and controlling the release mechanism of Escherichia coli in double W1/O/W2 emulsion globules in the presence of NaCl in the W2 phase, RSC Adv., 2015, 5, 105098–105110 RSC.
- G. M. Tedajo, S. Bouttier, J. Fourniat, J. L. Grossiord, J. P. Marty and M. Seiller, Release of antiseptics from the aqueous compartments of a w/o/w multiple emulsion, Int. J. Pharm., 2005, 288, 63–72 CrossRef CAS PubMed.
- S. Geiger, S. Togoz, A. Fructus, N. Jager-Lezer, M. Seiller, C. Lacombe and J.-L. Grossiord, Kinetics of swelling-breakdown of a W/O/W multiple emulsion: possible mechanism for the lipophilic surfactant effect, J. Controlled Release, 1998, 52, 99–107 CrossRef CAS PubMed.
- S. Geiger, N. Jager-Lezer, S. Togoz, M. Seiller and J.-L. Grossiord, Characterization of the mechanical properties of water/oil/water multiple emulsion oily membrane by a micropipette aspiration technique, Colloids Surf., A, 1999, 157, 325–332 CrossRef CAS.
- R. Lutz, A. Aserin, L. Wicker and N. Garti, Release of electrolytes from W/O/W double emulsions stabilized by a soluble complex of modified pectin and whey protein isolate, Colloids Surf., B, 2009, 74, 178–185 CrossRef CAS PubMed.
- M. F. Ficheux, L. Bonakdar, F. Leal-Calderon and J. Bibette, Some stability criteria for double emulsions, Langmuir, 1998, 14, 2702–2706 CrossRef CAS.
- J. A. Omotosho, T. L. Whateley, T. K. Law and A. T. Florence, Nature of the oil phase and the release of solutes from multiple (W/O/W) emulsion, J. Pharm. Pharmacol., 1986, 38, 865–870 CrossRef CAS PubMed.
- P. Colinart, S. Delepine, G. Trouve and H. Renon, Water transfer in emulsified liquid membrane processes, J. Membr. Sci., 1984, 20(2), 167–187 CrossRef CAS.
- M. H. Ly, M. Naïtali-Bouchez, T. Meylheuc, M.-N. Bellon-Fontaine, T. M. Le, J.-M. Belin and Y. Waché, Importance of bacterial surface properties to control the stability of emulsions, Int. J. Food Microbiol., 2006, 112, 26–34 CrossRef CAS PubMed.
- M. H. Ly, M. Aquedoc, S. Goudota, M. L. Lea, P. Cayotd, T. M. Teixeirac, J.-M. Leb, J. –M. Belina and Y. Waché, Interactions between bacterial surfaces and milk proteins, impact on food emulsions stability, Food Hydrocolloids, 2008, 22, 742–751 CrossRef CAS.
- L. S. Dorobantu, A. K. C. Yeung, M. J. Foght and M. R. Gray, Hydrophobic bacteria stabilization of oil-water emulsions by hydrophobic bacteria, Appl. Environ. Microbiol., 2004, 70(10), 6333 CrossRef CAS PubMed.
- H. Firoozmand and D. Rousseau, Tailoring the morphology and rheology of phase-separated biopolymer gels using microbial cells as structure modifiers, Food Hydrocolloids, 2014, 42, 204–214 CrossRef CAS.
- P. Prachaiyo and L. A. McLandsborough, Oil-in-water emulsion as a model system to study the growth of Escherichia coli O157:H7 in a heterogeneous food system, J. Food Sci., 2003, 63(3), 1018–1024 CrossRef.
- M. L. Parker, T. F. Brocklehurst, P. A. Gunning, H. P. Coleman and M. M. Robins, Growth of Food-borne pathogenic bacteria in oil-in-water emulsion: I-methods for investigating the form of growth, J. Appl. Bacteriol., 1995, 78, 601–608 CrossRef CAS PubMed.
- T. F. Brocklehurst, M. J. Parker, P. A. Gunning, H. P. Coleman and M. M. Robins, Growth of Food-borne pathogenic bacteria in oil-in-water emulsion: I-Effects of emulsion structure on growth parameters and form of growth, J. Appl. Bacteriol., 1995, 78, 609–615 CrossRef CAS PubMed.
- L. Boitard, D. Cottineta, C. Kleinschmitta, N. Bremonda, J. Baudrya, G. Yvertb and J. Bibettea, Monitoring single-cell bioenergetics via the coarsening of emulsion droplets, Proc. Natl. Acad. Sci. U. S. A., 2012, 109(19), 7181–7186 CrossRef CAS PubMed.
- H. Miao, S. Ratnasingam, C. S. Pu, M. M. Desai and C. C. Sze, Dual fluorescence system for flow cytometric analysis of Escherichia coli transcriptional response in multi-species context, J. Microbiol. Methods, 2009, 76, 109–119 CrossRef CAS PubMed.
- A. Miles, S. Misra and O. Irwin, The estimation of the bactericidal power of the blood, J. Hyg., 1938, 38, 732 CrossRef CAS PubMed.
- J. Weiss and G. Muschiolik, Factors Affecting the Droplet Size of Water-in-Oil Emulsions (W/O) and the Oil Globule Size in Water-in-Oil-in-Water Emulsions (W/O/W), J. Dispersion Sci. Technol., 2007, 28, 703–716 CrossRef CAS.
- J. Galindo-Alvarez, V. Sadtler, L. Choplin and J.-L. Salager, Viscous oil emulsification by catastrophic phase inversion: Influence of oil viscosity and process conditions, Ind. Eng. Chem. Res., 2011, 50, 5575–5583 CrossRef CAS.
- L. Sapei, M. A. Naqvi and D. Rousseau, Stability and release properties of double emulsions for food applications, Food Hydrocolloids, 2012, 27, 316–323 CrossRef CAS.
- L. Wen and K. D. Papadopoulos, Effects of Osmotic Pressure on Water Transport in W1/O/W2 Emulsions, J. Colloid Interface Sci., 2001, 235, 398–404 CrossRef CAS PubMed.
- N. Jager-Lezer, I. Terrisse, F. Bruneau, S. Tokgoz, L. Ferreira, D. Clausse, M. Seiller and J.-L. Grossiord, Influence of lipophilic surfactant on the release kinetics of water-soluble molecules entrapped in a W/O/W multiple emulsion, J. Controlled Release, 1997, 45, 1–13 CrossRef CAS.
- K. Chong-Kook, K. Seong-Cheol, S. Hee-Jong, M. K. Kyoung, O. Kyoung-Hee, L. Yong-Bok and O. In-Joon, Preparation and characterization of cytarabine-loaded w/o/w multiple emulsions, Int. J. Pharm., 2005, 124, 61–67 Search PubMed.
- L. Wen and K. D. Papadopoulos, Visualization of water transport in W1/O/W2 emulsions, Colloids Surf., A, 2000, 174, 159–167 CrossRef CAS.
- D. Shuster, Encyclopedia of Emulsion Technology: Application, CRC Press, NY, USA, 1985, vol. 4, pp. 13–17 Search PubMed.
- T. Frising, C. Noïk and C. Dalmazzone, The liquid/liquid sedimentation process: From droplet coalescence to technologically enhanced water/oil emulsion gravity separators: A review, J. Dispersion Sci. Technol., 2006, 27, 1035–1057 CrossRef CAS.
- T. Krebs, C. P. G. H. Schroen and R. M. Boom, A microfluidic study of oil-water separation kinetics, Advances in Fluid Mechanics IX, 2012, vol. 74, pp. 427–438 Search PubMed.
- Emulsion formation and stability, ed. T. F. Tadros, Wiley-VCH Publishers, Weinheim, 2013, pp. 173–174 Search PubMed.
- C. V. Nikiforidis and V. Kiosseoglou, Competitive displacement of oil body surface proteins by Tween80-Effect on physical stability, Food Hydrocolloids, 2011, 25, 1063–1068 CrossRef CAS.
- D. J. McClements, Food Emulsions: Principles, and Techniques, CRC Press, Boca Raton, FL, USA, 3rd edn, 2015, pp. 336–337 Search PubMed.
- F. Leal-Calderon, S. Homer, A. Goh and L. Lundin, W/O/W emulsions with high internal droplet volume fraction, Food Hydrocolloids, 2012, 27, 30–41 CrossRef CAS.
- T. Schmidts, D. Dobler, C. Nissing and F. Runkel, Influence of hydrophilic surfactants on the properties of multiple W/O/W emulsions, J. Colloid Interface Sci., 2009, 338, 184–192 CrossRef CAS PubMed.
- J. Jiao and D. J. Burgess, Rheology and Stability of Water-in-Oil-in-Water Multiple Emulsions Containing Span 83 and Tween 80, AAPS PharmSci, 2003, 5(1), 12 CrossRef PubMed.
- J. Jiao, D. G. Rhodes and D. J. Burgess, Multiple emulsion stability: Pressure balance and interfacial film strength, J. Colloid Interface Sci., 2002, 250, 444–450 CrossRef CAS PubMed.
- D. Vasiljevic, J. Parojcic, M. Primorac and G. Vuleta, An investigation into the characteristics and drug release properties of multiple W/O/W emulsion systems containing low concentration of lipophilic polymeric emulsifier, Int. J. Pharm., 2006, 309, 171–177 CrossRef CAS PubMed.
- F. Opawale and D. J. Burgess, Influence of interfacial rheological properties of mixed emulsifier films on the stability of water-in-oil-in-water emulsions, J. Pharm. Pharmacol., 1998, 50, 965–973 CrossRef CAS PubMed.
- M. Mooney, The viscosity of a concentrated suspension of spherical particles, J. Colloid Sci., 1951, 6, 162–170 CrossRef CAS.
- Y. Kita, S. Matsumoto and D. Yonezawa, Viscometric method for estimating the stability of W/O/W type multiple-phase emulsions, J. Colloid Interface Sci., 1977, 62, 87–94 CrossRef CAS.
- G. Lewis, I. W. Taylor, A. W. Nienow and C. J. Hewitt, The application of multi-parameter flow cytrometry to the study of recombinant Escherichia coli batch fermentation processes, J. Ind. Microbiol. Biotechnol., 2004, 31, 311–322 CrossRef CAS PubMed.
Footnote |
† Electronic supplementary information (ESI) available. See DOI: 10.1039/c6ra17091a |
|
This journal is © The Royal Society of Chemistry 2016 |