DOI:
10.1039/C6RA16640G
(Paper)
RSC Adv., 2016,
6, 69421-69430
Inhibition of chikungunya virus replication by hesperetin and naringenin
Received
28th June 2016
, Accepted 8th July 2016
First published on 11th July 2016
Abstract
Chikungunya virus (CHIKV) is an emerging arbovirus, which has recently become globally important. In recent years, it has posed a progressive threat to humankind, causing the development of possibly life-threatening and incapacitating arthritis. Currently, there is no available effective antiviral drug for chikungunya infection. Thus, finding and developing lead compounds with anti-CHIKV activity that could be further developed into a practical treatment is urgently required. Several studies have reported the wide-ranging antiviral activities of flavanones; however, an inhibitory effect of selected compounds has yet to be shown against CHIKV. In this study, we investigated the antiviral properties of two types of flavanones, namely naringenin and hesperetin, against CHIKV in vitro replication. Our data have shown dose dependent inhibitory effects for naringenin and hesperetin against CHIKV intracellular replication using different assays, including the CHIKV replicon cell line, time-of-addition and virus yield assays. The antiviral activity of the compounds was further investigated by the evaluation of CHIKV protein expression using a quantitative immunofluorescence assay and western blotting. In brief, these compounds presented significant antiviral activity against CHIKV, reducing both the CHIKV replication efficiency and down-regulating the production of viral proteins involved in replication. Naringenin with IC50 = 6.818 μM (SI = 80.27) and hesperetin with IC50 = 8.500 μM (SI = 23.34) inhibited the post entry stages of CHIKV replication activity. In conclusion, the obtained data from the current study suggest that naringenin and hesperetin could be potential candidates to be developed further as anti-CHIKV therapeutic agents.
Introduction
Chikungunya virus (CHIKV) is an arthropod-borne virus that has re-emerged as a prominent public health threat in many parts of the world in recent years. CHIKV was first recognized in a febrile individual during an outbreak in Tanzania (East Africa) in the 1950s.1,2 In 2005–2006, CHIKV came into focus due to large outbreaks, with nearly a million suspected cases reported in the Indian Ocean island of La Reunion.3,4 Since then, further recurrent outbreaks have been observed periodically in Africa, the Indian Ocean islands and many parts of South-East Asia.5,6 In 2013 and 2014, huge outbreaks of over of 1.2 million cases were reported in the Caribbean island of Saint Martin and America.7,8 Worldwide, millions of cases of CHIKV have been reported in over 50 countries including India, Thailand, Indonesia, Malaysia, Singapore, the United States and some European countries like Italy and France.9,10
Serologically, CHIKV belongs to the Semliki Forest Virus (SFV) complex of the genus Alphavirus, within the family Togaviridae.11 CHIKV is an enveloped virus with icosahedral symmetry, possessing a genome of approximately 11.8 kb in size. It consists of single-stranded, positive-sense RNA with two open reading frames (ORFs).12 The 5′ ORF is translated from genomic RNA and encodes four non-structural proteins (nsP1, 2, 3 and 4), which together form the virus replicase,13 whereas the 3′ ORF is translated from a subgenomic RNA and encodes the capsid protein (C), the two surface envelope glycoproteins (E1 and E2) and two small peptides named (E3 and K6).14–16 Similar to other alphaviruses, CHIKV enters the host cells by receptor-mediated endocytosis in a clatherin coated vesicle, via a pH-dependent mechanism, which induces an irreversible conformational change in the glycoproteins and the release of a nucleocapsid into the cytoplasm.17 Afterwards, nonstructural protein precursors are translated from viral mRNA to initiate the replication. PE2 and E1 are translated in the endoplasmic reticulum and processed in the Golgi apparatus, and then removed to the plasma membrane, where pE2 is cleaved into E2 and E3 in the host cell by furin-like protease activity.18 The virion assembly arises in the cytoplasm and buds at the cell membrane as a spherical particle of 65 to 70 nm in diameter, which comprises genomic RNA molecules and the capsid protein along with the lipid bilayer envelope derived from the host cell membrane.15,19
Sequence analysis of genomes isolated from different geographical areas has revealed that CHIKV has three genotype variants, which are so-called West African, East, Central and South African (ECSA) and Asian.20 CHIKV is primarily transmitted to humans by several species of mosquitoes of the genus Aedes including Aedes Aegypti and Aedes albopictus, found both in rural and urban areas.21,22 It is the common cause of chikungunya fever (CHIKF) in humans, a viral disease marked by excruciating and persistent myalgia, sudden-onset fever, nausea, headache, fatigue, lymphadenitis and maculopapular rashes. In addition, many patients suffer from severe joint pain, which may persist in 10–30% of patients and may last for months or even years beyond the acute stage. The name itself reveals the contorted posture of infected patients: “Chikungunya” in Swahili or Mankonde language means “to walk bent over”.23,24 CHIKV attacks osteoblast cells,25 endothelial cells, epithelial fibroblast cells,26 muscle satellite cells,27 monocytes28 and macrophages.29 Since currently no licensed vaccine or antiviral drugs have been developed against CHIKV infection, most of the treatment regimens are symptomatic with administration of non-steroidal anti-inflammatory drugs or corticosteroids for the symptomatic relief of arthralgia and myalgia.30
Due to the huge risk of mosquito vectors spreading the CHIKV infection in many parts of the world, there is an urgent demand to prevent the spread of CHIKV and develop safe and effective antivirals to control the symptoms and reduce the occurrence of future incidences. Numerous studies have been performed toward the development of CHIKV antiviral therapy in recent years. Chloroquine phosphate, an antimalarial drug, was reported to be effective in the treatment of chronic CHIKV arthritis symptoms.31 However, there is still no endorsement for the effectiveness of chloroquine to treat the chikungunya infection, owing to unsuccessful clinical trial results.32 It was also shown that the viral replication and pathogenesis of the disease increases due to the effects of chloroquine in a mouse model trial33. The antiviral drug arbidol (ARB), licensed for influenza A and B, and acute respiratory infections, was found to be a potent inhibitor of an in vitro CHIKV infection34,35. Ribavirin, a licensed antiviral agent for the treatment of the respiratory syncytial virus, displayed good inhibitory effects on an in vitro CHIKV infection and was found to be beneficial in relieving CHIKV induced joint pain36. Ribavirin37 in combination with interferons38 and mercaptopurine39 were also shown to have antiviral activity against CHIKV. Currently, none of these antiviral agents have been permitted for treating CHIKV infection and the status of drug discovery is still in the premature stages.
In recent years, extensive studies have been conducted on a wide variety of active phytochemicals to develop new antiviral drugs, as they can be less toxic (or non-toxic), cost effective and have minor adverse effects40. Flavonoids (polyphenolic plant secondary metabolites) are naturally found in various plants and are responsible for flower and fruit pigmentation41. According to their molecular structure, flavonoids can be divided into a variety of classes such as flavones, flavonols, flavanones and others.42 Flavanones are highly and almost exclusively found in citrus fruits and in some aromatic herbs including mint, parsley, hot peppers and celery, which are widely and frequently consumed in the world. Citrus flavanones mostly occur in the glycoside or agylcone form. Naringenin and hesperetin are the most essential flavanones in the agylcone form (Fig. 1), and possess promising anti-oxidant, anti-inflammatory, anti-carcinogenic, antimicrobial and immune-stimulatory properties both in vitro and in vivo.43 Up to now, many studies have evidenced that naringenin and hesperetin exhibit effective antiviral activity against many different viruses. Hesperetin was shown to inhibit the replication of poliovirus type 1, herpes simplex virus type 1, parainfluenza virus type 3, influenza, respiratory syncytial virus and sindbis virus.44–47 Naringenin was also shown to have a great inhibition on hepatitis C virus (HCV) and the CHIKV replicon cell system, along with a slight inhibition of HIV-1 virus.48–50 More recently, it has also been reported that hesperetin and naringenin also inhibit the replication of yellow fever virus.51
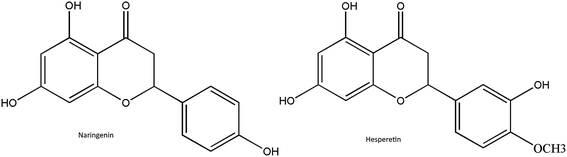 |
| Fig. 1 The chemical structure of naringenin and hesperetin. | |
Therefore, in this study we evaluate the in vitro antiviral activity of naringenin and hesperetin against a clinical isolate of CHIKV in a cell culture system. Our results suggest that the anti-viral effects of these compounds may support the development of effective therapeutics to deal with CHIKV infection.
Material and methods
Cell lines, virus and virus titration
Baby hamster kidney cells (BHK-21) and African green monkey kidney cells (Vero) from American Type Culture Collection (ATCC) were grown in Eagle's Minimum Essential Medium (EMEM, Gibco, NY, USA) supplemented with 10% inactivated fetal bovine serum (FBS) and penicillin–streptomycin. BHK–CHIKV replicon cells50 were grown in Dulbecco's Minimum Essential Medium (DMEM, Gibco, NY, USA) supplemented with 8% fetal bovine serum (FBS), 2% tryptose-broth phosphate and penicillin–streptomycin. Both cell lines were cultured at 37 °C under 5% CO2. The CHIKV (ECSA) genotype E1-226V, isolated from an outbreak in Johor in 2008 (coded as MY/065/08/FN295485),52,53 was used in this study. CHIKV was further propagated in the BHK-21 cell line, titrated using the tissue culture infectious dose 50 (TCID50) method54 and stored at −80 °C in a freezer. During the time of virus propagation and the antiviral assay, the FBS concentration of the cell culture medium was reduced to 2%.
Chemicals
Hesperetin and naringenin were purchased from Sigma-Aldrich Corporation (Sigma-Aldrich, St. Louis, MO, USA) and dissolved to completion in 0.1% dimethyl sulfoxide (DMSO) (Sigma-Aldrich, St. Louis, MO, USA), to prepare stock solutions at 66.16 and 183.65 μM, respectively. The stock solutions were then stored at −20 °C until use. Ribavirin (Sigma-Aldrich, St. Louis, MO, USA) was proposed as a control compound with well-known anti-CHIKV activity.36,37
Cell viability assay
The in vitro cytotoxicity of the compounds was studied against Vero and BHK-21 cells using MTS (3-(4,5-dimethylthiazol-2-yl)-5-(3-carboxymethoxyphenyl)-2-(4-sulfophenyl)-2H-tetrazolium) assay kit (Promega, WI, USA), and following the manufacturer's instruction. Confluent Vero cells in a 96-well cell culture microplate were exposed to different concentrations of each compound in triplicate wells for 2 days at 37 °C under 5% CO2. At the end of the incubation period, MTS solution was added to each well and the cells were maintained for 4 hours at 37 °C under 5% CO2. The absorbance of each well was measured at a wavelength of 570 nm using an Infinite 200 Pro multiplate reader (Tecan, Männedorf, Switzerland). Graph Pad Prism 5 (Graph Pad Software Inc., San Diego, CA, USA, 2005) was used to calculate the half maximal cytotoxic concentration (CC50).
In vitro antiviral assays
Primary screening – CHIKV replicon cell system. BHK–CHIKV replicon cells were seeded onto an opaque-white 96-well plate with a clear bottom at a confluency of approximately 80–90% (Corning Inc., NY, USA). The cells were exposed to different concentrations of each compound for 48 hours at 37 °C under 5% CO2. After the incubation period, the expression of Renilla luciferase (Rluc) activity of the CHIKV replicon was detected using a Renilla luciferase assay kit (Promega, WI, USA), following the manufacturer's protocol. The resulting luminescence signals were measured using a GloMAX 20/20 Luminometer (Promega, WI, USA), and plotted against the log transformation of the concentrations of each compound. Moreover, a sigmoidal curve fit with variable slope was created to calculate the half maximal inhibitory concentration (IC50) value for each compound using Graph Pad Prism 5 (Graph Pad Software Inc., San Diego, CA, USA, 2005).
Time-of-addition. Vero cells were grown in a 96-well microplate. Then, the confluent cell monolayers were treated with the highest concentration of naringenin, hesperetin and ribavirin at different time intervals before and after virus infection. The plate was then incubated at 37 °C under 5% CO2 for 48 hours, which was followed by conducting a virus yield assay on the collected supernatant from each well.
Entry inhibition assay
Confluent Vero cells in a 24-well plate were exposed to CHIKV (MOI = 1) for 1 h at 4 °C to allow for viral attachment. The infected cells were then washed twice with PBS to remove the unabsorbed viruses and treated with the compounds at various concentrations for 2 h at 37 °C. The cells were then washed with PBS and treated with citrate buffer (pH = 3) to inactivate the non-internalized viral particles. Finally, the cells were overlaid with EMEM containing 2% FBS and incubated for 48 h at 37 °C under 5% CO2.
Post-entry assay
Vero cells were grown in a 24-well microplate. Confluent cells were infected with CHIKV (MOI = 1) for 2 h. After incubation, the cells were washed twice with PBS, treated with the compounds and incubated for 48 h at 37 °C under 5% CO2.
Anti-adsorption assay
Confluent Vero cells in a 24-well microplate were infected with CHIKV (MOI = 1), followed by the addition of the compounds. Cells were then incubated for 1 h at 4 °C. The treated Vero cells were then rinsed with PBS three times to remove the free viral particles, supplemented with EMEM containing 2% FBS and incubated for 2 days at 37 °C under 5% CO2.
Virus inactivation assay
A suspension of CHIKV (MOI = 10) with different concentrations of hesperetin and naringenin were mixed and incubated for 2 h at 37 °C. In order to reduce the potential effect of the compounds on the virus adsorption, the Vero cells were infected and incubated with a 1000 fold diluted virus suspension for 1 h at 37 °C. Afterwards, the cells were washed twice with PBS, supplemented with EMEM containing 2% FBS and incubated for 2 days at 37 °C under 5% CO2.
Quantitative real time RT-PCR
For each antiviral analysis, a qRT-PCR was performed to measure the virus yield. For this, the extracellular CHIKV RNA was harvested from the supernatant of the infected Vero cells and the 136 base region of the nsP3 encoding sequences underwent amplification as described by Chiam and colleagues.55 The actual primers were nsP3-F (5′-GCGCGTAAGTCCAAGGGAAT-3′) and nsP3-R (5′-AGCATCCAGGTCTGACGGG-3′). The cDNA was consequently produced from the earlier extracted RNA (QIAGEN, Germany) by utilizing the nsP3-R primer and Superscript III Reverse Transcriptase (Life Technologies, USA) according to the manufacturer's protocol. The unincorporated primers were then digested with 20 U of Exonuclease I (New England Biolabs, USA). The qRT-PCR assay was performed with a Step-OnePlus Real-Time PCR System (Life Technologies, USA) with 2× Power SYBR Green PCR Master Mix (Life Technologies, USA), following the manufacturer's protocol. Cycling parameters were 95 °C for 10 min, followed by 40 cycles of 95 °C for 15 s and 60 °C for 1 min. Melting curve analysis was performed to validate the amplified RNA. The viral RNA in each sample was measured with a standard curve that was designed from CHIKV inoculums of known titer. The standard curve contained 12 points of concentration ranging from 1012 to 102 FFU ml−1 and each concentration was assayed in triplicate.
Immunofluorescence assay (IFA)
CHIKV infected and treated Vero cells in a 96-well plate were fixed using 4% paraformaldehyde for 30 min and were then air-dried for 5 min. The cells were then washed three times with PBS and subsequently stained with monoclonal rabbit anti-CHIKV E2 antibody (1
:
300) and incubated for 1 h at 37 °C. Following washing with PBS, the cells were stained with secondary antibody (1
:
1000 anti-rabbit IgG Fab2 conjugated with Alexa Fluor (RT) 488 – Cell Signaling Technology, MA, USA), and were incubated for 1 h at 37 °C. The cells were then covered with DAPI (Thermo Scientific, MA, USA) for 15 min at room temperature before being washed 3 times with PBS. Images were captured using a high content screening system (Operetta, PerkinElmer, Hamburg, Germany) and the immunofluorescence signals were measured and analyzed using Harmony Software Version 3.5.1 (PerkinElmer, Hamburg, Germany).
Western blot analysis
Vero cells were added to 75 cm2 tissue culture flasks and infected with CHIKV (MOI = 1). Then they were treated with different concentrations of selected compounds and incubated at 37 °C. After 48 h of incubation, the treated cells were scraped, washed with PBS and lysed using 1% Triton X100 (Sigma-Aldrich, St. Louis, MO, USA) containing a protease inhibitor cocktail (Sigma-Aldrich, St. Louis, MO, USA) at 4 °C for 45 min. Cellular debris was removed by centrifugation at 16
000 × g for 5 min. Protein concentrations of each sample were quantified using a Micro BCA™ Protein Assay Kit (Thermo Scientific, Rockford, IL). Total proteins were then prepared in 0.5 ml of 10× Laemmli sample buffer (100 mM Tris–HCl, pH 6.8, 40% glycerol, 8% SDS, 40 mM DTT, 0.04 mg ml−1 bromophenol blue) and separated onto 10% SDS-PAGE. The gels were equilibrated in Towbin buffer (0.025 M Tris, 0.192 M glycine 20% methanol) for 10 min and the proteins were transferred to a PVDF membrane using the Bio-Rad wet transfer system (Bio Rad, San Francisco, CA). The membranes were blocked with PBS 1% Casein Blocker (Bio Rad, San Francisco, CA) for 1 h at room temperature. The membranes were then rinsed three times with PBS Tween 20 before being incubated with primary anti-CHIKV nsP1 and anti-CHIKV nsP3 rabbit polyclonal antibodies in 1% casein solution. After washing the membranes three times with PBS containing Tween 20 for 15 min each time, they were then incubated with the secondary goat anti-rabbit IgG (Abcam, Cambridge, UK) antibodies conjugated with horseradish peroxidase (HRP) for 1 h at room temperature. The membranes were then washed three times with PBS containing Tween 20 for 15 min each time. Primary anti-β-actin mouse monoclonal antibody conjugated with HRP (Cell Signaling Technology, MA, USA) dissolved in 1% casein was used for the detection of loading control. The membranes were then washed again three times with PBS containing Tween 20 for 15 min each time and developed by a colorimetric method using appropriate substrates (Thermo Scientific, Rockford, IL).
Results
Determination of potential cytotoxic activity of compounds
The cytotoxic effects of the compounds on the Vero cells and/or BHK-21 cells were first evaluated using an MTS assay and microscopic observations. Table 1 shows the 50% cytotoxic concentration values (CC50) of each compound against Vero and BHK-21. 0.1% DMSO, as a vehicle control, did not show any cytotoxicity against the cells.
Table 1 The CC50 values of the tested flavanones and nucleoside analogue on Vero cells and BHK-21 cells. MTS assay was used to evaluate the cytotoxicity of the compounds. Different concentrations of the compounds of up to 500 μM were used to treat the Vero cells and BHK-21 cells for 2 days. All experiments were conducted in triplicate
Compounds |
Vero cells |
BHK-21 cells |
CC50 (μM) |
CC50 (μM) |
NA = not applicable. |
Naringenin |
547.3 ± 6.60 |
831.3 ± 4.085 |
Hesperetin |
198.4 ± 4.40 |
>125 |
Ribavirin |
>500 |
NAa |
Primary screening assays. The CHIKV replicon cell line was used to study the antiviral activity of the compounds. The replicon was constructed from a non-infectious replicon of CHIKV as well as the virus replicase proteins together with puromycin acetyltransferase, EGFP and Renilla luciferase marker genes.50 The replicon was then transfected into BHK cells to yield a stable cell line. The Rluc activity in the CHIKV replicon is proportional to the viral replicon RNA replication. In this experiment, it was found that hesperetin and naringenin inhibited the Rluc activity with IC50 = 85.0 ± 5.104 and 93.27 ± 11.150 μM, respectively (Fig. 2). Hesperetin (P = 0.0027) and naringenin (P = 0.0014) showed a moderate inhibitory effect against the CHIKV replicon, which validates that both compounds affect the post-entry steps of CHIKV infection. These data also indicate that they can interfere with CHIKV RNA replication by affecting the viral replicase system. Since these compounds showed inhibitory activity against CHIKV in vitro replication, it is worthwhile to implement further evaluation on different antiviral assays.
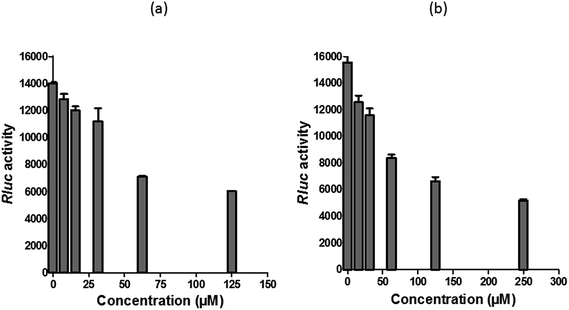 |
| Fig. 2 Evaluation of anti-CHIKV activity of selected flavanones using CHIKV replicon cell line. Both flavanones, hesperetin (a) and naringenin (b), are able to reduce the percentage of Rluc activity produced by the CHIKV replicon in a dose-dependent manner. The Rluc activity was measured at 48 h post treatment. Vehicle-treated (0.1% DMSO) cells were used as control (“0” concentration). Data from triplicate assays were plotted and analyzed using one-way ANOVA (Kruskal–Wallis test), Graph Pad Prism (Version 5, Graph Pad Software Inc., San Diego, CA), P < 0.05. Error bars represent standard errors of triplicate means. | |
Time-of-addition studies. A time-of-addition assay was performed to determine the effects of varying the time of the compound addition on CHIKV replication. Hesperetin, naringenin and ribavirin (as a control) were added at different time points before and after infection of Vero cells with CHIKV. Significant inhibition of virus replication was observed when hesperetin and naringenin were added 2 h before the viral infection. Fig. 3 shows that hesperetin exerts its antiviral activity when added prior to the virus infection up until 4 h post infection, while naringenin exhibits antiviral activity up until 12 h post infection. Ribavirin also shows antiviral activity at 2 h prior to infection until 2 h post infection. The antiviral activity of ribavirin at these hours was confirmed beforehand since it acts as a RNA replication inhibitor. The results indicate that hesperetin and naringenin may inhibit the early to middle stages of CHIKV. However, naringenin displayed potent antiviral effects compared to hesperetin. These two compounds were then selected for further analysis of their anti-CHIKV activity, due to their significant inhibition of CHIKV infection and minimal cytotoxicity, as well as their novel status as antiviral agents.
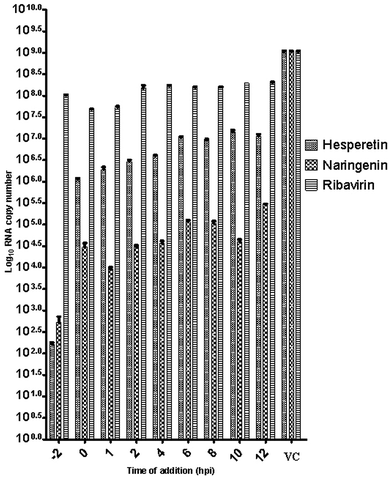 |
| Fig. 3 Effect of time-of-addition of selected flavanones on CHIKV RNA copy number. CHIKV viral load was reduced significantly at the early hour (−2 hpi) of hesperetin, naringenin and ribavirin treatment in the time-of-addition assay. “VC” refers to the non-treated CHIKV-infected controls. Statistical significance was analyzed from a one-way ANOVA (Kruskal–Wallis test) and Dunn's multiple comparisons post-test. | |
Hesperetin and naringenin markedly inhibit post entry stages of CHIKV replication. Given that hesperetin and naringenin were most likely affecting the CHIKV intracellular replication of CHIKV, their activities on the post entry stages of CHIKV infection were investigated. Fig. 4 shows that hesperetin (P = 0.0090) with IC50 = 8.500 ± 0.270 μM, and naringenin (P = 0.0053) with IC50 = 6.818 ± 0.404 μM show potent intracellular anti-CHIKV activity. However, compared to ribavirin (P = 0.0061) with IC50 = 17.68 ± 0.503 μM as a positive control with defined anti-CHIKV activity, it could be concluded that the tested compounds exert stronger intracellular anti-CHIKV activity than ribavirin.
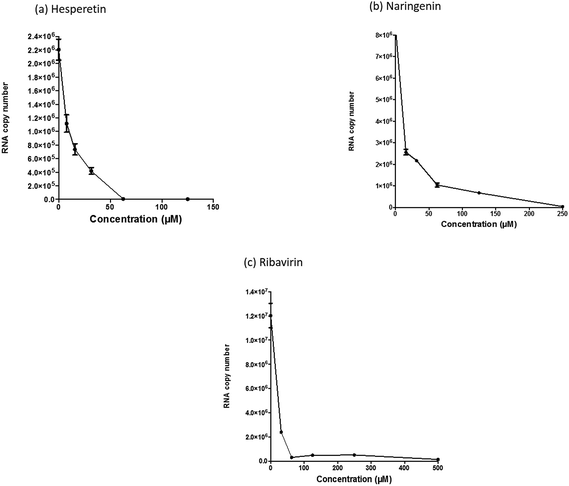 |
| Fig. 4 Evaluation of selected flavanones and ribavirin against the post-entry stages of CHIKV infection. Hesperetin, naringenin and ribavirin displayed potent antiviral activity at the post-entry stage of CHIKV infection. Statistical analysis was performed by using one-way ANOVA (Kruskal–Wallis test) where P < 0.05 is significant (“0” on the X-axis refers to the non-treated CHIKV-infected controls). Error bars represent standard errors of triplicate means. | |
Hesperetin and naringenin do not exhibit antiviral activity against early stages of CHIKV in vitro replication. Anti-entry, anti-adsorption and direct virucidal assays were also performed. As Fig. 5 shows, there was no antiviral activity of hesperetin and naringenin against these stages of the CHIKV replicative cycle.
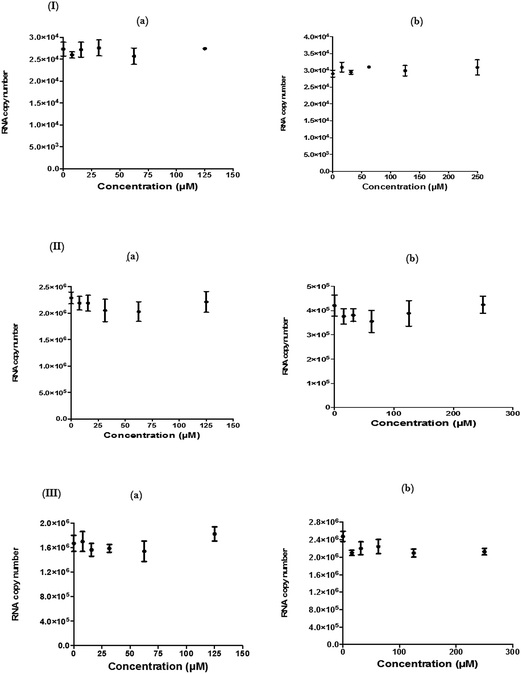 |
| Fig. 5 Effect of hesperetin and naringenin against early stages of CHIKV replication. There were no antiviral activities of hesperetin (a) and naringenin (b) against (I) anti-entry, (II) anti-adsorption and (III) direct virucidal stages of the CHIKV replication cycle. Statistical analysis was performed by using one-way ANOVA (Kruskal–Wallis test) where P < 0.05 is significant (“0” on the X-axis refers to the non-treated CHIKV-infected controls). Error bars represent standard errors of triplicate means. | |
Development of immunofluorescence assay. An immunofluorescence assay was conducted to distinguish the CHIKV antigen presentation as an indicator for the successful replication and infection of CHIKV, as well as to discover the degree of inhibition exerted by the tested compounds. Fig. 6 shows that all tested compounds display a dose-dependent inhibition of CHIKV infection compared to the vehicle control. According to an automated calculation by a HTS machine, it was discovered that hesperetin and naringenin at a concentration of 125 and 250 μM, respectively, inhibited ≥90% of the CHIKV antigen presentation. Therefore, this screening platform was found to be beneficial and consistent for testing the compounds effectively and revealing their potential as CHIKV inhibitors.
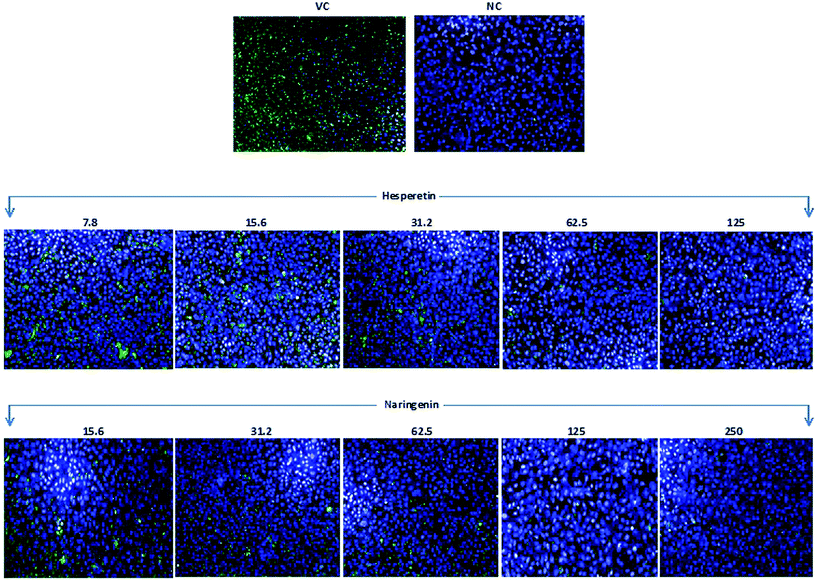 |
| Fig. 6 Evaluation of flavanones on CHIKV activity using an immunofluorescence assay. Dose-dependent inhibition of hesperetin and naringenin on the CHIKV infectivity is shown. Detection of the CHIKV E2 protein is used as the indication of the successful CHIKV infection. Cell nuclei are stained with DAPI (blue) and CHIKV infection is indicated by Alexa Fluor 488 (green) staining. | |
In order to determine the effect of hesperetin and naringenin on CHIKV protein synthesis, western blot analyses on nsP1 and nsP3 proteins were performed. Fig. 7 shows a dose-dependent reduction of CHIKV, nsP1 and nsP3 following hesperetin and naringenin treatment. Using hesperetin at concentrations of 125 and 62.5 μM and naringenin at 250 and 125 μM gave rise to minimal amounts of nsP3 and nsP1 proteins. This suggested that hesperetin and naringenin might inhibit CHIKV protein production, leading to a decrease in infectious virus titers as seen above. Nevertheless, there was a general trend of dose-dependent inhibition of CHIKV infection by hesperetin and naringenin, supporting the results obtained with the different experimental approaches reported above. β-Actin was used as a loading control in the experiment, as well as to ensure that the concentration of both compounds used in this study did not affect the synthesis and expression of the host cellular proteins.
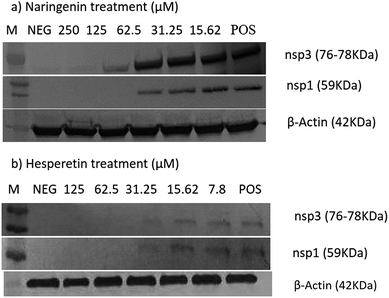 |
| Fig. 7 Hesperetin and naringenin inhibited the accumulation of CHIKV-encoded proteins. Western blot analyses were performed to determine the effect of both compounds on the production of nsP1 and nsP3 proteins. Reduction of CHIKV nsP1 and nsP3 proteins was observed upon naringenin and hesperetin treatment. β-Actin was used as a loading control for each compound. | |
Discussion
Since several compounds with anti-CHIKV drugs such as chloroquine, arbidol and ribavirin have not exhibited any beneficial effects in clinical cases so far, there is an urgent demand to search for antiviral compounds with high viral inhibitory activities, low toxicity and high efficiency. To that end, the current study was designed to evaluate the anti-CHIKV activity of hesperetin and naringenin, which are well known to exhibit various medicinal properties including antiviral activity. Several studies have reported that both flavanones were able to block the replication of a variety of other viruses.44,45,47,51 It has also been reported that both flavanones are able to reduce the activity and the expression of the microsomal triglyceride transfer protein and acyl-coenzyme A cholesterol acyltransferase 2, which modulate apolipoprotein B (apoB) secretion that is vital for HCV budding from the human hepatoma cell line.56,57 Naringenin has been discovered to inhibit virion assembly, and, more recently, docking studies of HCV nonstructural protein (NS2) protease as targets have indicated that naringenin is a successful inhibitor of the virus replications.58,59
In our study, the cytotoxicity assay showed that hesperetin and naringenin at concentrations of up to 125 and 250 μM can be used to exert antiviral activity without affecting the cell viability. It was shown that hesperetin with IC50 = 8.500 ± 0.270 μM and naringenin with IC50 = 6.818 ± 0.404 μM possess significant antiviral effects that act by inhibiting viral replication in a post-entry assay. Interestingly, this result is consistent with our primary data from the time-of-addition study, where the viral yield was significantly reduced in CHIKV-infected cells upon treatment with hesperetin and naringenin shortly before and a few hours post infection (−2.0 and 2 hpi). In addition, ribavirin exhibited the most potent antiviral activity for up to 1 h post infection. However, like the other tested compounds, it showed anti-CHIKV activity for up to 12 h post infection, which is consistent with previous findings.60 These findings are also consistent with the CHIKV replicon cell system, where no virus entry or exit takes place. In this regard, it was found that both compounds were capable of eliciting an inhibitory effect against the activity of the Rluc marker expressed by CHIKV replicon, which confirms that flavanones suppress CHIKV RNA replication in a dose dependent manner. While The Rluc is fused to the nsP3 protein of the virus, a reduction of Rluc activity causes the reduction of the nsP3–Rluc protein in the CHIKV replicon cell line. Recently, it has been reported that naringenin considerably reduces the expression of viral RNA levels by utilizing a CHIKV replicon system. In the same study, the authors examined different compounds using a different replicon system, and showed that naringenin was also capable of inhibiting the viral entry of Semliki Forest virus.50 Taken together, the obtained results were extensively explained by the antiviral assay, where there is no inhibition in anti-entry, anti-adsorption, or direct virucidal assays correspondingly.
To further confirm the potent anti-CHIKV activity of flavanones, we examined the effect of the tested compounds on viral antigen synthesis in CHIKV infected cells through a quantitative immunofluorescence assay using a monoclonal antibody against an envelope protein of CHIKV, together with western blotting which showed a significant reduction in structural and non-structural protein synthesis. This observation could be due to the inhibition of CHIKV RNA replication and/or transcription, or even the inhibition of viral protein synthesis and processing. It is most likely that some of the non-structural proteins of CHIKV become possible targets for hesperetin and naringenin. These possible targets may include the nsP1 protein, which participates in the synthesis of the negative strand of viral RNA and RNA capping, as well as the nsP3 protein, which is another key component of the viral life cycle which includes viral RNA synthesis, virulence and protein–protein interactions. Since it has been reported that the nsP3 protein is the only non-structural protein that contains a large number of serine and threonine residues in order to be phosphorylated by activated host kinases,13 both flavanones may target the phosphorylation status of nsP3 which leads to the restriction of viral replication and increases host survival. Reduction of E2 protein expression is possibly due to the suppression of replication, either directly, or via the inhibition of ns-protein(s). This viral protein reduction is applicable from the point of view of the development of effective antivirals, as the E2 protein plays an important role in viral glycoproteins and is essential for receptor binding. Nevertheless, to confirm the direct effect of the compounds against protein synthesis and processing, further experiments are needed, which could be considered in future studies. Nevertheless, regarding the molecular size and the solubility of hesperetin and naringenin, this might lead to significant penetration of these molecules through the biological membrane, causing them to enter the cytoplasm or other subcellular compartments. They therefore may be noble candidates for further investigation towards antiviral drug development.51
Conclusion
In summary, we have convincingly shown that hesperetin and naringenin exhibit significant anti-CHIKV activity by interfering with virus intracellular replication. These compounds suppress post-entry stages of viral replication in a dose dependent manner. In agreement with this, the expression of proteins, needed for RNA replication, and also the expression of viral structural E2 protein, were down regulated. These findings warrant future mechanistic, in vivo anti-viral, toxicity and pharmacokinetic studies as part of the process to evaluate hesperetin and naringenin as potential therapeutic candidates against CHIKV. Another approach for inhibiting CHIKV infection is by using targeting cellular factors such as protein kinases and other cellular factors, which are involved in CHIKV replication, and/or by the induction of immune-based cellular enzymes that possess antiviral activity.
Author contributions statement
KZ and SAB conceived and designed the experiments. AH, PH, AJ, PY and RL performed the experiments and collected the data. AH analyzed the data and wrote the paper.
Competing interests
The authors declare that they have no competing interests.
Acknowledgements
The authors would like to thank the Ministry of Higher Education, Malaysia, for the High Impact Research (HIR) MOHE Grant (H20001-E000087) and the Fundamental Research Grant Scheme (FP054-2014B). We also would like to thank University of Malaya for the Postgraduate Research Fund (PG120-2015A). We also would like to acknowledge the team at the Tropical Infectious Disease Research Centre, Department of Medical Microbiology, Faculty of Medicine, University of Malaya for providing help and support throughout the study.
References
- E. G. Strauss and J. H. Strauss, in The Togaviridae and Flaviviridae, Springer, 1986, pp. 35–90 Search PubMed.
- M. C. Robinson, Trans. R. Soc. Trop. Med. Hyg., 1955, 49, 28–32 CrossRef CAS PubMed.
- R. N. Charrel, X. de Lamballerie and D. Raoult, N. Engl. J. Med., 2007, 356, 769 CrossRef CAS PubMed.
- M. Enserink, Science, 2006, 311, 1085 CrossRef CAS PubMed.
- E. D'Ortenzio, M. Grandadam, E. Balleydier, J. S. Dehecq, M. C. Jaffar-Bandjee, A. Michault, S. F. Andriamandimby, J. M. Reynes and L. Filleul, Eurosurveillance: bulletin europeen sur les maladies transmissibles = European communicable disease bulletin, 2009, p. 14 Search PubMed.
- M. Grandadam, V. Caro, S. Plumet, J.-M. Thiberge, Y. Souarès, A.-B. Failloux, H. J. Tolou, M. Budelot, D. Cosserat and I. Leparc-Goffart, Emerging Infect. Dis., 2011, 17, 910 CrossRef PubMed.
- E. W. Campion, S. C. Weaver and M. Lecuit, N. Engl. J. Med., 2015, 372, 1231–1239 CrossRef PubMed.
- S. C. Weaver, Arrival of chikungunya virus in the new world: prospects for spread and impact on public health, 2014, vol. 8, ch. 6, p. e2921 Search PubMed.
- S. S. Cherian, A. M. Walimbe, S. M. Jadhav, S. S. Gandhe, S. L. Hundekar, A. C. Mishra and V. A. Arankalle, Infect., Genet. Evol., 2009, 9, 16–23 CrossRef CAS PubMed.
- H. C. Hapuarachchi, K. B. A. T. Bandara, S. D. M. Sumanadasa, M. D. Hapugoda, Y.-L. Lai, K.-S. Lee, L.-K. Tan, R. T. P. Lin, L. F. P. Ng and G. Bucht, J. Gen. Virol., 2010, 91, 1067–1076 CrossRef CAS PubMed.
- D. E. Griffin, Fields Virology, Lippincott/The Williams & Wilkins Co, Philadelphia, PA, 5th ed, 2007 Search PubMed.
- J. H. Strauss and E. G. Strauss, Microbiol. Rev., 1994, 58, 491 CAS.
- J. Jose, J. E. Snyder and R. J. Kuhn, Future Microbiol., 2009, 4, 837–856 CrossRef CAS PubMed.
- A. M. Q. King, M. J. Adams and E. J. Lefkowitz, Virus Taxonomy: Classification and Nomenclature of Viruses: Ninth Report of the International Committee on Taxonomy of Viruses, Elsevier, 2011 Search PubMed.
- B. Simizu, K. Yamamoto, K. Hashimoto and T. Ogata, J. Virol., 1984, 51, 254–258 CAS.
- M. Solignat, B. Gay, S. Higgs, L. Briant and C. Devaux, Virology, 2009, 393, 183–197 CrossRef CAS PubMed.
- J. E. Voss, M.-C. Vaney, S. Duquerroy, C. Vonrhein, C. Girard-Blanc, E. Crublet, A. Thompson, G. Bricogne and F. A. Rey, Nature, 2010, 468, 709–712 CrossRef CAS PubMed.
- B. L. Tang, Cell. Microbiol., 2012, 14, 1354–1363 CrossRef CAS PubMed.
- N. Higashi, A. Matsumoto, K. Tabata and Y. Nagatomo, Virology, 1967, 33, 55–69 CrossRef CAS PubMed.
- S. AbuBakar, I. C. Sam, P.-F. Wong, N. MatRahim, P.-S. Hooi and N. Roslan, Emerging Infect. Dis., 2007, 13, 147 CrossRef PubMed.
- M. Diallo, J. Thonnon, M. Traore-Lamizana and D. Fontenille, Am. J. Trop. Med. Hyg., 1999, 60, 281–286 CAS.
- K. A. Tsetsarkin, D. L. Vanlandingham, C. E. McGee and S. Higgs, PLoS Pathog., 2007, 3, e201 Search PubMed.
- W. Maek-a-nantawat and U. Silachamroon, Presence of autoimmune antibody in chikungunya infection, Case reports in medicine, 2009, 2, 840183 Search PubMed.
- M. Sourisseau, C. Schilte, N. Casartelli, C. Trouillet, F. Guivel-Benhassine, D. Rudnicka, N. Sol-Foulon, K. Le Roux, M.-C. Prevost and H. Fsihi, PLoS Pathog., 2007, 3, e89 CrossRef PubMed.
- M. Noret, L. Herrero, N. Rulli, M. Rolph, P. N. Smith, R. W. Li, P. Roques, G. Gras and S. Mahalingam, J. Infect. Dis., 2012, 206, 455–457 CrossRef CAS PubMed.
- O. Schwartz and M. L. Albert, Nat. Rev. Microbiol., 2010, 8, 491–500 CrossRef CAS PubMed.
- S. Ozden, M. Huerre, J.-P. Riviere, L. L. Coffey, P. V. Afonso, V. Mouly, J. De Monredon, J.-C. Roger, M. El Amrani and J.-L. Yvin, PLoS One, 2007, 2, e527 Search PubMed.
- Z. Her, B. Malleret, M. Chan, E. K. S. Ong, S.-C. Wong, D. J. C. Kwek, H. Tolou, R. T. P. Lin, P. A. Tambyah and L. Rénia, J. Immunol., 2010, 184, 5903–5913 CrossRef CAS PubMed.
- K. Labadie, T. Larcher, C. Joubert, A. Mannioui, B. Delache, P. Brochard, L. Guigand, L. Dubreil, P. Lebon and B. Verrier, J. Clin. Invest., 2010, 120, 894 CAS.
- B. Queyriaux, F. Simon, M. Grandadam, R. Michel, H. Tolou and J.-P. Boutin, Lancet Infect. Dis., 2008, 8, 2–3 CrossRef PubMed.
- S. W. Brighton, S. Afr. Med. J., 1984, 66, 217–218 CAS.
- M. Khan, S. R. Santhosh, M. Tiwari, P. V. Lakshmana Rao and M. Parida, J. Med. Virol., 2010, 82, 817–824 CrossRef CAS PubMed.
- R. K. Maheshwari, V. Srikantan and D. Bhartiya, J. Virol., 1991, 65, 992–995 CAS.
- I. Delogu, B. Pastorino, C. Baronti, A. Nougairède, E. Bonnet and X. de Lamballerie, Antiviral Res., 2011, 90, 99–107 CrossRef CAS PubMed.
- A. Di Mola, A. Peduto, A. La Gatta, L. Delang, B. Pastorino, J. Neyts, P. Leyssen, M. de Rosa and R. Filosa, Bioorg. Med. Chem., 2014, 22, 6014–6025 CrossRef CAS PubMed.
- R. Ravichandran and M. Manian, J. Infect. Dev. Countries, 2008, 2, 140–142 CrossRef CAS.
- S. Briolant, D. Garin, N. Scaramozzino, A. Jouan and J. M. Crance, Antiviral Res., 2004, 61, 111–117 CrossRef CAS PubMed.
- M. Takehara, Kobe J. Med. Sci., 1975, 21, 23 CAS.
- J. W. S. Geme, D. S. Horrigan and P. S. Toyama, Exp. Biol. Med., 1969, 130, 852–855 CrossRef.
- P. Kaur and J. J. H. Chu, Drug Discovery Today, 2013, 18, 969–983 CrossRef CAS PubMed.
- B. Havsteen, Biochem. Pharmacol., 1983, 32, 1141–1148 CrossRef CAS PubMed.
- C. A. Rice-Evans, N. J. Miller and G. Paganga, Free Radicals Biol. Med., 1996, 20, 933–956 CrossRef CAS PubMed.
- D. Pathak, K. Pathak and A. K. Singla, Fitoterapia, 1991, 62, 371–389 CAS.
- H. K. Kim, W. K. Jeon and B. S. Ko, Planta Med., 2001, 67, 548–549 CrossRef CAS PubMed.
- T. N. Kaul, E. Middleton and P. L. Ogra, J. Med. Virol., 1985, 15, 71–79 CrossRef CAS PubMed.
- E.-A. Bae, M. J. Han, M. Lee and D.-H. Kim, Biol. Pharm. Bull., 2000, 23, 1122–1124 CAS.
- A. Paredes, M. Alzuru, J. Mendez and M. Rodríguez-Ortega, Biol. Pharm. Bull., 2003, 26, 108–109 CAS.
- Y.-M. Lin, H. Anderson, M. T. Flavin, Y.-H. S. Pai, E. Mata-Greenwood, T. Pengsuparp, J. M. Pezzuto, R. F. Schinazi, S. H. Hughes and F.-C. Chen, J. Nat. Prod., 1997, 60, 884–888 CrossRef CAS PubMed.
- Y. Nahmias, J. Goldwasser, M. Casali, D. van Poll, T. Wakita, R. T. Chung and M. L. Yarmush, Hepatology, 2008, 47, 1437–1445 CrossRef CAS PubMed.
- L. Pohjala, A. Utt, M. Varjak, A. Lulla, A. Merits, T. Ahola and P. Tammela, PLoS One, 2011, 6, e28923 CAS.
- M. Castrillo, T. Córdova, G. Cabrera and M. Rodríguez-Ortega, Avances en Biomedicina, 2015, 4, 69–78 Search PubMed.
- I. C. Sam, Y. F. Chan, S. Y. Chan, S. K. Loong, H. K. Chin, P. S. Hooi, R. Ganeswrie and S. AbuBakar, J. Clin. Virol., 2009, 46, 180–183 CrossRef CAS PubMed.
- L. P. Chew and H. H. Chua, Med. J. Malays., 2009, 64 Search PubMed.
- L. J. Reed and H. Muench, Am. J. Epidemiol., 1938, 27, 493–497 Search PubMed.
- C. W. Chiam, Y. F. Chan, S. K. Loong, S. S. J. Yong, P. S. Hooi and I. C. Sam, Diagn. Microbiol. Infect. Dis., 2013, 77, 133–137 CrossRef CAS PubMed.
- L. J. Wilcox, N. M. Borradaile, L. E. de Dreu and M. W. Huff, J. Lipid Res., 2001, 42, 725–734 CAS.
- M. Castrillo, T. Córdova, G. Cabrera and M. R. Ortega, Avances en Biomedicina, 2015, 4, 69–78 Search PubMed.
- R. Khachatoorian, V. Arumugaswami, S. Raychaudhuri, G. K. Yeh, E. M. Maloney, J. Wang, A. Dasgupta and S. W. French, Virology, 2012, 433, 346–355 CrossRef CAS PubMed.
- S. S. Lulu, A. Thabitha, S. Vino, A. M. Priya and M. Rout, Nat. Prod. Res., 2015, 1–5 Search PubMed.
- H. A. Rothan, H. Bahrani, Z. Mohamed, T. C. Teoh, E. M. Shankar, N. A. Rahman and R. Yusof, PloS one, 2015, 10, e0126360 Search PubMed.
|
This journal is © The Royal Society of Chemistry 2016 |