DOI:
10.1039/C6RA16335A
(Paper)
RSC Adv., 2016,
6, 86904-86908
Composites of graphene oxide and epoxy resin assuming a uniform 3D graphene oxide network structure
Received
24th June 2016
, Accepted 4th September 2016
First published on 7th September 2016
Abstract
Graphene based composites usually show unique interior structures, extraordinary properties and diverse applications. In this work, with waterborne epoxy resin and an aqueous suspension of graphene oxide (GO) as raw materials, composites of graphene oxide and epoxy resin (GO/ER) with different GO to ER ratios were prepared. The scanning electron microscopy (SEM) and transmission electron microscopy (TEM) images show that the as-obtained composites have unique structures in which the GO sheets assemble into three-dimensional (3D) networks. It was also illustrated that the mechanical properties, the glass transition temperatures (Tg) and thermal conductivities could be tuned by varying the content of GO in the composites. For instance, the mechanical strength and elastic modulus of the GO/ER composites containing 2% (in weight) GO were increased by 50% and 19.6%; the Tg was increased by 15.59 °C; the thermal conductivity was improved by 1.4 fold in comparison to the bare epoxy resin.
1. Introduction
Epoxy resins have been widely used as matrices for polymer based composites due to their unique stiffness, dimensional stability, chemical resistance and strong adhesion to certain embedded reinforcements.1–11 Up to now, a variety of epoxy-based composites with different fillers, such as silver,1 clay,2,3 alumina,4 silica,5,6 α-zirconiumphosphate,7 titanate,8 carbon fiber9 and carbon nanotubes,10,11 have been successfully prepared and their properties have been explored well. More recently, graphene and graphene oxide (GO) sheets have also been used as the fillers for the epoxy-based composites. For example, Yu et al.12 prepared graphene/epoxy composites containing 25% graphene (in volume) and found that the thermal conductivity of the composite can reach 6.44 W mK−1, which is much higher than that of the bare epoxy resin. However, the higher graphene content added unacceptably to the cost of the as-prepared composites and that may limit their practical applications.
Alternatively, as a derivative of graphene, GO does not only preserve the single atomic layered motif of graphene, but also has enriched periphery functional groups. And thus it shows unique hydrophilic property, which make GO sheets be proper fillers for composites.13,14 For instance, Wang et al. prepared GO/epoxy composites and found that GO can reduce the thermal expansion and increase the thermal conductivity of epoxy.15 Similarly, Liu et al. also prepared GO reinforced epoxy resin and found that the toughness of the epoxy resins could be improved by increasing the GO content.16 Additionally, chemically functionalized GO sheets including mainly polyetheramine and silane functionalized GO sheets have also been used as fillers in polymeric composites.17,18 However, the poor solubility of GO sheets in most organic solvents limited the preparation of the polymeric composites with relatively high GO contents and uniform distribution, which is a dominating factor for practical performance of the composites.19
With development of emulsification technique of epoxy, the waterborne epoxy can be produced routinely in industry.20–22 Considering the excellent water solubility of GO sheets, in this work, we intend to prepare the composites of GO and epoxy resin (GO/ER) using the waterborne epoxy particles and the aqueous GO suspension. The composition, structure, morphology, mechanical and thermomechanical performances, as well as thermal conductivity, are studied. As will be shown, the GO sheets can assemble into a uniform 3D network structure within the epoxy matrix. The properties such as mechanical properties, the glass transition temperatures and thermal conductivities of the composites could be improved largely. The formation mechanism of 3D network of GO in the composites is proposed and the relationship between the unique structural morphology and the properties is also discussed.
2. Experimental
2.1 Preparation of GO/ER composites
GO was prepared by a modified Hummers method that we described previously.23,24 The waterborne epoxy (MU-618, the 50% aqueous emulsion) and the waterborne hardener (KH-700) were purchased from Shanghai Forlon Chemical Development Company. To prepare GO/ER composites, the GO was dispersed in the water with a concentration of 4.0 mg mL−1. The waterborne epoxy was mixed with the hardener with a ratio of 5
:
1 in weight and then different amount of the GO suspension was added and stirred for 2 h. The mixed suspension was firstly concentrated through rotary evaporation. Then they were poured onto a Teflon plate and spread out by a tape casting method. Finally, the composite films were cured in an oven at 60 °C for 5 h and followed at 150 °C for 2 h. The contents of GO in the composites were kept at 0% (bare epoxy), 0.5%, 1%, 1.5%, and 2% in weight.
2.2 Characterization
The morphologies and structures of as-prepared composites were characterized with transmission electron microscope (TEM) (JEOL, JEM-2001F, Japan) and field emission scanning electronic microscopy (FESEM) (Zeiss, Ultra55, Germany). The specimens for TEM were prepared by dropping the aqueous suspension of composite on a copper grid, which was then drained with a piece of filter paper. The SEM images were acquired directly on a GO/ER composite film.
The functional groups in GO and its interaction with epoxy matrix were recorded using a FT-IR spectrometer (Bruker, Germany) with a resolution of 4 cm−1. The specimens for FT-IR measurement were prepared by grinding the dried powders of the composites with KBr together and then compressed into thin pellets.
Strips with dimension of 20 × 4 × 0.25 mm were cut from GO/ER films and were used for tensile test. The tensile properties were measured on a hydraulic universal material testing machine (Instron4465, Instron Corporation, USA) with a cross-head speed of 4 mm min−1. Dynamic mechanical properties of the composites were tested using a dynamic mechanical analyser (DMA, Netzsch, Germany). The specimens were subjected to a sinusoidal displacement of 60 μm at a frequency of 1 Hz and a static loading force of 0 N. The scanning temperature range was set to 23–250 °C and scanning rate was 4 °C min−1.
Thermal conductivities (λ(T)) of bare epoxy resin and GO/ER composites were calculated using thermal diffusivity (a(T)) and specific heat (cp(T)) measured on a LFA 467 Nanoflash (Netzsch, Germany). The dimensions of the samples used for thermal conductivity measurements were 10 × 10 × 0.3 mm.
3. Results and discussion
3.1 Microstructure of GO/ER composites
Fig. 1 shows the SEM and TEM images of bare waterborne epoxy particles and GO/waterborne epoxy mixtures before curing. As illustrated in Fig. 1(a), the sizes of as-purchased spherical waterborne epoxy particles range in 100 nm to 5 μm in diameter. Once the aqueous GO solutions and epoxy particles were mixed under a vigorous stirring, the GO sheets would coated onto the surface of epoxy particles (Fig. 1(c) and (d)). By comparing with Fig. 1(b) and (d), it is found that the thickness of GO coating layer is about 0.5 nm, revealing the single atomic layer motif of the GO. The reason might be that the GO sheets have abundant oxygen containing groups, such as carboxylic, hydroxyl and epoxide groups, which can interact with the enriched epoxide groups of epoxy particles either forming chemical bonds or weaker van der Waals bonds.
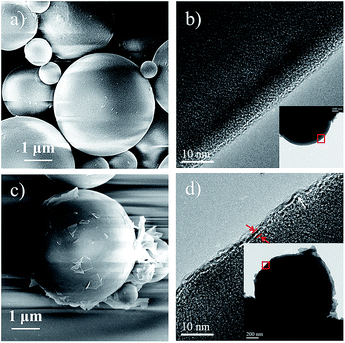 |
| Fig. 1 FESEM images of (a) bare waterborne epoxy particles and (c) the mixture of GO/waterborne epoxy particles before curing. TEM images of (b) bare waterborne epoxy particles and (d) the mixture of GO/waterborne epoxy particles before curing. | |
Fig. 2 gives the optical microscopic images of bare epoxy resin and GO/ER composite. As can be seen, different from bare epoxy resin, the black color of the GO/ER composite provides a hint of embedding of the GO within epoxy resin. To get insight into their structural morphology, the topography images of the bare epoxy and the GO/ER composite were observed using FESEM. As depicted in Fig. 3(a), the surface of bare epoxy resin is smooth and featureless. However, for the GO/ER, lots of ripple-like features (Fig. 3(b)) were observed which might be originated from GO sheets. To illustrate the assumption, both bare epoxy and the GO/ER composite were etched in concentrated sulfuric acid. It was found that the bare epoxy resin were dissolved equally in the concentrated sulfuric acid. In contrast, as presented in Fig. 4, although the epoxy in the GO/ER composite was etched by sulfuric acid, GO sheets were preserved well. Significantly, the high magnification FESEM images (Fig. 4(c) and (d)) demonstrated that GO sheets interconnected each other and form a uniform 3D network.
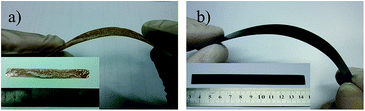 |
| Fig. 2 Opical images of (a) bare epoxy resin and (b) the GO/ER composite. | |
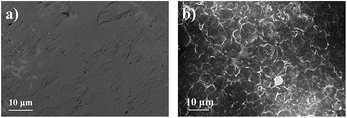 |
| Fig. 3 FESEM images of the surfaces of (a) bare epoxy resin and (b) the GO/ER composite containing 2% GO (in weight). | |
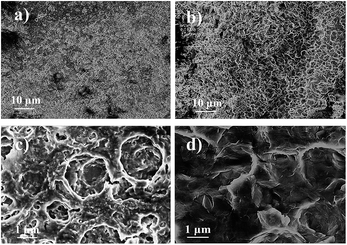 |
| Fig. 4 FESEM images of GO/ER composite thin film were etched in concentrated sulfuric acid for 5 min (a and c) and 1 h (b and d). | |
3.2 FT-IR and curing reaction analysis of GO/ER composites
In order to explore the curing reaction and formation mechanism of 3D GO network, FT-IR analysis were conducted. As can be seen from Fig. 5, the peak appears at 1536 cm−1 is attributed to the vibration of H–N–C
O groups. It is obvious that the intensity of this peak becomes stronger when involving GO into epoxy resin, which indicating that the reaction between carboxyl of GO and amidogen of curing agent has took place.25 The peak appears at 1250 cm−1 is ascribed to the vibration of N–C groups, which is the product of the reaction between epoxy groups and the amidogen of curing agent.26 Since both GO and waterborne epoxy emulsion have epoxy groups, the intensity of this peak is dependent on the amount of curing agent. As shown in Fig. 5, when 1% GO was added, the intensity of this peak is almost unchanged. However, it becomes weaker as the GO content increases to 2%, which indicating that the reactions between waterborne epoxy emulsion and curing agent was reduced when more GO was involved. From Fig. 1(c) and (d), it is obvious that partial surface of waterborne epoxy was covered by GO sheets and thus the reactions between waterborne epoxy emulsion and curing agent was partially blocked.
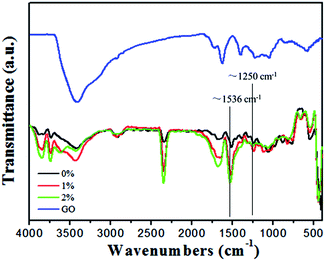 |
| Fig. 5 FT-IR spectra of GO, bare epoxy and GO/ER composites. | |
3.3 Formation mechanism of 3D GO network
Based on the discussion above, the formation mechanism of 3D GO network is proposed as shown in Fig. 6. When the aqueous GO solution, waterborne epoxy emulsion and curing agent were mixed together, the GO sheets and curing agent were around the waterborne epoxy particles. As the water was evaporated gradually, the waterborne epoxy particles, GO sheets and curing agent were in touch with each other. And thus, the curing reactions took place. Specifically, the epoxy groups of waterborne epoxy and GO reacted with the amidogen of curing agent and as a result, the waterborne epoxy particles connected with each other or GO sheets by N–C bonding. Meanwhile, the carboxyl of GO either reacted with amidogen of curing agent which made GO sheets combine together by H–N–C
O bonding and therefore 3D GO network were formed around the waterborne epoxy particles.
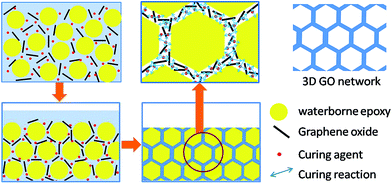 |
| Fig. 6 Schematical presentation of the curing reactions and formation mechanisms of 3D GO network. | |
3.4 Properties of GO/ER composites
The tensile strength and elastic modulus of GO/ER composite is shown in Fig. 7. It can be seen that both of them are increased as the increasing of GO content. Typically, when the GO content is 2%, the tensile strength can reach to 49.7 MPa, improved about 50% in comparison with that of the bare epoxy resin. The elastic modulus is about 753.4 MPa, increased by 20%. The improvement of mechanical properties is attributed to the curing reactions and uniform 3D GO network. On one hand, since both GO sheets and waterborne epoxy particles can react with curing agent, the bonding strength between waterborne epoxy particles can be enhanced in the presence of GO sheets. On the other hand, the crack propagation during fracture can be retarded due to the 3D GO network.
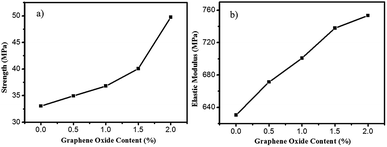 |
| Fig. 7 Strength (a) and elastic modulus (b) of GO/ER composites with different GO contents. | |
Fig. 8(a) presents the temperature effect on the loss factor (tan
δ = E′′/E′) for the GO/ER composites with different GO contents. The tan
δ represents the ratio of the dissipation energy (E′′, in heat) to the stored energy (E′). It can be seen that the value of tan
δ increases first and then decreases as the temperature increasing for all the GO/ER composites. Furthermore, the maximum value of tan
δ decreases as the GO content increasing. However, the temperature related to the maximum value of tan
δ is increased. It is known that this temperature represents the glass transition temperature (Tg). It means the Tg of the composites tend to increase with the increasing of the GO content, as shown in Fig. 8(b). It is generally accepted that the rise of Tg for polymeric materials is associated with the restriction of the molecule motion, the reduction of free volume, and/or the increasing of the cross-linking degree of the molecular chains.27 As indicated in Section 3.2 and 3.3, the involving of GO into the epoxy could intensify the bonding strength of epoxy particles and form a relative rigid 3D GO network. As a result, the molecule motion was restricted and the free volume during phase boundary was reduced. Therefore, it can be concluded that both thermo-mechanical property and thermal reliability are improved in the GO/ER composite.
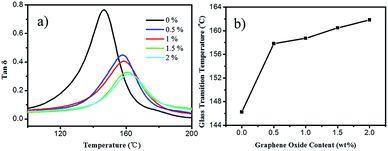 |
| Fig. 8 Loss factor (tan δ) curves (a) and the Tg values (b) of bare epoxy and GO/ER composites containing 0.5, 1, 1.5 and 2% GO. | |
For the sake of the potential application in thermal management, the thermal conductivity of GO/ER composite was examined. As depicted in Fig. 9, the thermal conductivity of GO/ER is increased compared with bare epoxy resin. The reason on one hand is ascribed to the formation of 3D GO network, in which the heat can be conducted easily since GO has relative high thermal conductivity. On the other hand, according to the principle of solid physics, the motion of phonons play a great role on the thermal conductivity for a solid. Specifically, when the GO is added into epoxy, the bond strength between epoxy particles is enhanced and as a result, the velocity of phonons in GO/ER composite is increased, which is beneficial to the increasing of thermal conductivity. It should be pointed out that the thermal conductivity of GO/ER composite with 2% GO is less than that of 1% GO content. The reason might be attributed to the presence of more interface between GO and epoxy as more GO was added into epoxy since the phonon could be scattered when it meets the interface. Therefore, in order to increase the thermal conductivity of GO/ER composite, the content of GO should be kept at a proper level and the future research should be concentrated on the selection and optimization of the curing agents.
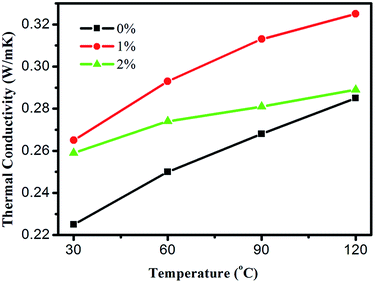 |
| Fig. 9 Thermal conductivities of bare epoxy resin and the GO/ER composites with different GO content measured in a temperature range 30 to 120 °C. | |
4. Conclusion
In summary, the composites of GO and epoxy with varied GO contents have been successfully prepared using waterborne epoxy and aqueous GO suspension as raw materials. It has been demonstrated that the GO sheets are well dispersed within the epoxy matrix and form a uniform 3D network structure. In comparison with bare epoxy resin, the strength, elastic modulus, glass transition temperature, thermal stability and thermal conductivity of GO/ER composites can be improved by tuning properly the contents of the GO in the composites. Based on the results, we envisage that introducing aqueous GO sheets into the waterborne epoxy should be a green and promising way to prepare the epoxy-based composites with advanced performances.
Acknowledgements
This work was funded by 973 Special Preliminary Study Plan (Grant no. 2014CB260411), National Natural Science Foundation of China (Grant no. 51602184), Specialized Research Fund for the Doctoral Program of Higher Education (Grant no. 20136125120003), Natural Science Foundation of Shaanxi Province (Grant no. 2015JM5151), Scientific Research Fund of San-qin Scholar (Grant no. BJ11-26) and Scientific Research Fund of Shaanxi University of Science & Technology (Grant no. BJ12-10).
Notes and references
- L. Qi, B. I. Lee, S. H. Chen, W. D. Samuels and G. J. Exarhos, Adv. Mater., 2005, 17, 1777–1781 CrossRef CAS.
- K. Wang, L. Chen, J. S. Wu, M. L. Toh, C. B. He and A. F. Yee, Macromolecules, 2005, 38, 788–800 CrossRef CAS.
- O. Becker, R. J. Varley and G. P. Simon, Eur. Polym. J., 2004, 40, 187–195 CrossRef CAS.
- B. Wetzel, F. Haupert and M. Q. Zhang, Compos. Sci. Technol., 2003, 63, 2055–2067 CrossRef CAS.
- S. Kang, S. I. Hong, C. R. Choe, M. Park, S. Rim and J. Kim, Polymer, 2001, 42, 879–887 CrossRef CAS.
- C. Chen, R. S. Justice, D. W. Schaefer and J. W. Baur, Polymer, 2008, 49, 3805–3815 CrossRef CAS.
- H. J. Sue, K. T. Gam, N. Bestaoui, N. Spurr and A. Clearfield, Chem. Mater., 2004, 16, 242–249 CrossRef CAS.
- Y. Rao, S. Ogitani, P. Kohl and C. P. Wong, J. Appl. Polym. Sci., 2002, 83, 1084–1090 CrossRef CAS.
- Y. K. Choi, K. Sugimoto, S. M. Song, Y. Gotoh, Y. Ohkoshi and M. Endo, Carbon, 2005, 43, 2199–2208 CrossRef CAS.
- A. Allaoui, S. Bai, H. M. Cheng and J. B. Bai, Compos. Sci. Technol., 2002, 62, 1993–1998 CrossRef CAS.
- Q. Wang, J. Dai, W. Li, Z. Wei and J. Jiang, Compos. Sci. Technol., 2008, 68, 1644–1648 CrossRef CAS.
- A. P. Yu, P. Ramesh, M. E. Itkis, E. Bekyarova and R. C. Haddon, J. Phys. Chem. C, 2007, 111, 7565–7569 CAS.
- A. K. Geim, Science, 2009, 324, 1530–1534 CrossRef CAS PubMed.
- S. Stankovich, D. A. Dikin, G. H. B. Dommett, K. M. Kohlhaas, E. J. Zimney, E. A. Stach, R. D. Piner, S. T. Nguyen and R. S. Ruoff, Nature, 2006, 442, 282–286 CrossRef CAS PubMed.
- S. R. Wang, M. Tambraparni, J. J. Qiu, J. Tipton and D. Dean, Macromolecules, 2009, 42, 5251–5255 CrossRef CAS.
- Q. H. Liu, X. F. Zhou, X. Y. Fan, C. Y. Zhu, X. Y. Yao and Z. P. Liu, Polym.-Plast. Technol. Eng., 2012, 51, 251–256 CrossRef CAS.
- L. Z. Guan, Y. J. Wan, L. X. Gong, D. Yan, L. C. Tang, L. B. Wu, J. X. Jiang and G. Q. Lai, J. Mater. Chem. A, 2014, 2, 15058–15069 CAS.
- Y. J. Wan, L. X. Gong, L. C. Tang, L. B. Wu and J. X. Jiang, Composites, Part A, 2014, 64, 79–89 CrossRef CAS.
- J. I. Paredes, S. Villar-Rodil, A. Martinez-Alonso and J. M. D. Tascon, Langmuir, 2008, 24, 10560–10564 CrossRef CAS PubMed.
- A. Wegmann, Prog. Org. Coat., 1997, 32, 231–239 CrossRef CAS.
- X. Shanhong, N. Girouard, G. Schueneman, M. L. Shofner and J. C. Meredith, Polymer, 2013, 54, 6589–6598 CrossRef.
- J. D. Elmore, D. S. Kincaid, P. C. Komar and J. E. Nielsen, J. Coat. Technol., 2002, 74, 63–72 CrossRef CAS.
- J. Zhang, H. Yang, G. Shen, P. Cheng, J. Zhang and S. Guo, Chem. Commun., 2010, 46, 1112–1114 RSC.
- G. I. Titelman, V. Gelman, S. Bron, R. L. Khalfin, Y. Cohen and H. Bianco-Peled, Carbon, 2005, 43, 641–649 CrossRef CAS.
- N. Yousefi, M. M. Gudarzi, Q. B. Zheng, X. Y. Lin, X. Shen, J. J. Jia, F. Sharif and J. K. Kim, Composites, Part A, 2013, 49, 42–50 CrossRef CAS.
- X. Y. Li, H. Deng, Z. Li, H. Xiu, X. D. Qi, Q. Zhang, K. Wang, F. Chen and Q. Fu, Composites, Part A, 2015, 68, 264–275 CrossRef CAS.
- K. Liu, S. Lu, S. Li, B. Huang and C. Wei, Iran. Polym. J., 2012, 21, 497–503 CrossRef CAS.
|
This journal is © The Royal Society of Chemistry 2016 |
Click here to see how this site uses Cookies. View our privacy policy here.