DOI:
10.1039/C6RA15266J
(Paper)
RSC Adv., 2016,
6, 64315-64322
A highly selective ratiometric fluorescent probe for the sensitive detection of hypochlorous acid and its bioimaging applications
Received
12th June 2016
, Accepted 28th June 2016
First published on 30th June 2016
Abstract
The development of simple techniques for monitoring hypochlorous acid (HOCl) in living cells is urgently needed to disclose the important roles of HOCl in biological systems. Although a number of fluorescent probes have been exploited to detect HOCl, most of them are easily influenced by other competing reactive oxygen species (ROS) and reactive nitrogen species (RNS), and disturbed by many factors including variabilities in excitation, probe distribution and environmental conditions due to the recognition of HOCl based on the fluorescence changes in single wavelength. Herein, in this work, we present a simple fluorescent probe AETU-HOCl containing a new recognition group (2-amino-ethyl)-thiourea (AETU) for the ratiometric detection of HOCl in living cells. Probe AETU-HOCl exhibits outstanding selectivity for HOCl over other ROS/RNS at higher concentrations (0.5 mM, 100 equiv.). More importantly, the rapid response (1 min) and high sensitivity (a detection limit of 3.9 nM) allow it to successfully visualize the intracellular HOCl levels by the ratiometric imaging. The present study would offer a simple, ratiometric, rapid, and ultrasensitive assay for the accurate determination of HOCl in the biological systems.
1. Introduction
Hypochlorous acid (HOCl) is well-known because of its unique antibacterial properties in the immune system.1 Endogenous HOCl is mainly generated from hydrogen peroxide and chloride ions with the help of myeloperoxidase (MPO) in immunocytes.2 However, more and more established evidences show that excessive production of HOCl is implicated in many diseases including atherosclerosis,3 lung injury,4 hepatic ischemia–reperfusion injury5 and neuron degeneration.6 Therefore, the development of simple techniques for monitoring HOCl in living cells is urgently needed to disclose the HOCl important roles in the biological systems.
Fluorescent probes that can respond directly to the change in concentration of HOCl would be well used to study the distribution of HOCl in living cells.7 Although various small-molecule probes for HOCl/OCl− have been reported for applications in vitro and in vivo,8 there are still some limitations. For example, most of the available fluorescent probes detect HOCl/OCl− only by the fluorescence changes in single wavelength, which may be disturbed by many factors during the quantitative detection, such as variabilities in excitation, probe distribution and environmental conditions.9 By contrast, ratiometric fluorescent probes can overcome the limitation via self-calibration of two emission bands. Almost all of them were restricted by acid–base conditions,7b,9,10 only a few of them can respond to HOCl across a wide range of pH.11 What's more, it is especially notable that most of them are easily influenced by other competing reactive oxygen species (ROS) and reactive nitrogen species (RNS).12 Thus, it is still a great challenge to exploit highly selective ratiometric fluorescent probes for HOCl.
Extensive research reveals that sulfur atom with strong electron-donating ability exists special reaction with HOCl.7c,10a,11a So we expected that the (2-amino-ethyl)-thiourea group may serve as a specific recognition group for HOCl. Recently, we developed a series of 4-aminonaphthalimide ratiometric fluorescent probes because of its outstanding internal charge transfer (ICT) structure and desirable photophysical properties.13 Herein, in this work, we synthesized an ICT-based ratiometric fluorescent probe AETU-HOCl with a receptor of (2-amino-ethyl)-thiourea group and 4-aminonaphthalimide fluorophore (Scheme 1). Our design strategy is that HOCl-triggered intramolecular cyclization of thiourea results in the change of emission wavelengths. Experimental results demonstrated that probe AETU-HOCl has the significant advantages of (1) ratio-imaging, (2) respond to HOCl at a wide range of pH, (3) highly selectivity over other ROS/RNS at higher concentrations (0.5 mM, 100 equiv.), (4) the lower detection limit (3.9 nM, based on 3σ/slope), (5) rapid response (about 1 min). Altogether, this study offers a specific fluorescent probe for the ratiometric detection of HOCl at nanomole level and a simple, rapid, accurate, ultrasensitive assay that would be widely applied in the various HOCl-related studies.
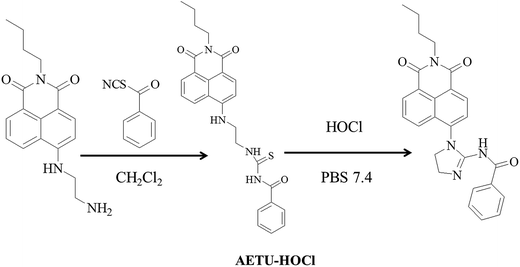 |
| Scheme 1 The synthesis and recognition reaction of probe AETU-HOCl for HOCl. | |
2. Experimental
2.1. Materials and measurements
Unless otherwise stated all chemical materials used in this study were gained from commercial products and used without further purification. Ultrapure water was obtained from Sartorius Arium 611DI system and used throughout the experiment. HOCl was standardized at pH 12 (ε292 nm = 350 M−1 cm−1).14 1H NMR and 13C NMR were measured on a Bruker AV-400 spectrometer with chemical shifts reported as ppm (in DMSO-d6, TMS as internal standard). Fluorescence emission spectra were carried out on a Horiba FluoroMax-4 spectrophotometer with excitation wavelength of 380 nm. All the fluorescence spectra were not revised. Absorption spectra were recorded on a UV-3101PC spectrophotometer. All pH were measured with a Sartorius basic pH-meter PB-10.
2.2. Synthesis of probe AETU-HOCl
As shown in Scheme 1, N-butyl-4-(2-aminoethylamino)-1,8-naphthalimide was readily synthesized by the reaction between N-butyl-4-bromo-1,8-naphthalimide and ethylenediamine.15
Benzoylisothiocyanate (163 mg, 1 mmol) was added dropwise to a solution of N-butyl-4-(2-aminoethylamino)-1,8-naphthalimide (311 mg, 1 mmol) in 20 mL dichloromethane. The reaction mixture was then stirred at room temperature for 4 h. The product was purified by chromatography using CH2Cl2 to yield probe AETU-HOCl (393 mg, 83%).
Melting point: 214–218 °C. FT-IR (KBr) ν: 3423.95, 3354.96, 2955.71, 2864.47, 1687.74, 1649.37, 1587.95, 1542.91, 1433.32, 1395.17, 1364.44, 1299.38, 1241.57, 772.47, 755.17, 707.70, 686.76, 663.80 cm−1. 1H NMR (400 MHz, DMSO-d6) δ (×10−6): 0.92 (t, J = 8.0 Hz, 3H), 1.29–1.38 (m, 2H), 1.55–1.62 (m, 2H), 3.69–3.73 (m, 2H), 3.99–4.06 (m, 4H), 7.01 (d, J = 8.0 Hz, 1H), 7.51 (t, J = 8.0 Hz, 2H), 7.64 (t, J = 8.0 Hz, 1H), 7.70 (t, J = 8.0 Hz, 1H), 7.87–7.94 (m, 3H), 8.25 (d, J = 8.0 Hz, 1H), 8.43 (d, J = 8.0 Hz, 1H), 8.69 (d, J = 8.0 Hz, 1H), 11.10 (t, J = 8.0 Hz, 1H), 11.42 (s, 1H). 13C NMR (100 MHz, DMSO-d6) δ (×10−6): 14.22, 20.31, 30.30, 41.62, 43.62, 104.63, 108.64, 120.68, 122.38, 124.85, 128.85, 128.97, 129.97, 131.11, 132.70, 133.40, 134.50, 151.04, 163.36, 164.18, 168.26, 181.24. ESI-MS calcd for C26H27N4O3S [M + H]+ 475.1804, found 475.1805.
2.3. Synthesis of N-[(N-butyl-1,8-naphthalimide)-4-yl-2,3-dihydro-1H-imidazol-2-yl]benzamide
Probe AETU-HOCl (200 mg, 0.42 mmol) was dissolved in 15 mL ethanol. Then NaOCl (2 mL) was added and stirred at room temperature for 10 min. The pure product was obtained by filtering reaction mixture (175 mg, 95%). The structure was confirmed to be N-[(N-butyl-1,8-naphthalimide)-4-yl-2,3-dihydro-1H-imidazol-2-yl]benzamide.
Melting point: >280 °C. FT-IR (KBr) ν: 3465.14, 2961.64, 1698.08, 1656.00, 1594.86, 1567.91, 1445.79, 1356.94, 1303.49, 1140.65, 1062.41, 995.06, 878.48, 848.96, 781.29, 759.50, 644.97, 619.46 cm−1. 1H NMR (400 MHz, DMSO-d6) δ (×10−6): 0.85 (t, J = 8.0 Hz, 3H), 1.23–1.29 (m, 2H), 1.44–1.49 (m, 2H), 3.93 (t, J = 8.0 Hz, 2H), 4.06–4.15 (m, 4H), 7.19 (t, J = 8.0 Hz, 2H), 7.34 (t, J = 8.0 Hz, 1H), 7.61 (d, J = 8.0 Hz, 2H), 7.87 (t, J = 8.0 Hz, 1H), 7.96 (d, J = 8.0 Hz, 1H), 8.51–8.59 (m, 3H), 9.44 (s, 1H). ESI-MS calcd for C26H25N4O3 [M + H]+ 441.1927, found 441.1942.
2.4. General procedure for analysis
The parent stock solutions of probe AETU-HOCl (1 mM) were dissolved in the ethanol. Parent stock solutions (1 mM) of HOCl and other bioanalytes were prepared in ultrapure water. Test solutions were prepared by placing 50 μL of the probe stock solution into a test tube, adding an appropriate aliquot of each stock solution, and then diluting the solution to 10 mL with the mixture of ethanol and ultrapure water (5
:
5, v/v) containing PBS (5 mM, pH 7.4). All spectra were obtained in a quartz cuvette (path length = 1 cm).
2.5. Cell culture
RAW 264.7 cells were cultured in DMEM containing 10% fetal bovine serum, 1% penicillin, and 1% streptomycin at 37 °C (w/v) in a 5% CO2 and 95% air incubator MCO-15AC (Sanyo, Tokyo, Japan). The concentrations of counted cells were adjusted to 1 × 106 cells per mL for confocal imaging in high-glucose DMEM (4.5 g of glucose per L) supplemented with 10% fetal bovine serum (FBS), NaHCO3 (2.0 ng L−1), and 1% antibiotics (penicillin/streptomycin, 100.0 U mL−1). Cultures were maintained at 37 °C under a humidified atmosphere containing 5% CO2.
After 24 h, the control cells were incubated with 10 μM probe AETU-HOCl in culture media for 30 min at 37 °C, and washed with PBS three times. Then the fluorescence imaging of cells was carried out. On the other hand, cells pretreated with PMA (2.0 μg mL−1) for 1 h were incubated with 10 μM probe AETU-HOCl in culture media for another 30 min at 37 °C, and washed with PBS three times. Then the fluorescence imaging of cells was carried out. The fluorescence imaging of RAW 264.7 macrophage cells was observed under an Olympus FV1000 confocal fluorescence microscope.
2.6. Cytotoxicity assays
RAW 264.7 macrophage cells were cultured in culture media (DMEM) in an atmosphere of 5% CO2 and 95% air at 37 °C. The cells were seeded into 96-well plates at a density of 3 × 103 cells per well in culture media, then 0, 5, 10, 20 and 30 μM (final concentration) probe AETU-HOCl were added, respectively. Next, the cells were incubated at 37 °C in an atmosphere of 5% CO2 and 95% air for 24 h. Finally, 20 μL 3-(4,5-dimethylthiazol-2-yl)-2,5-diphenyltetrazolium bromide (MTT, 5 mg mL−1) was added and cultured for another 4 h, respectively.
3. Results and discussion
3.1. Absorption spectra properties
The absorption spectrum of free probe AETU-HOCl (5 μM) was measured in the mixture of ethanol and ultrapure water (1
:
1, v/v) containing (5 mM PBS, pH 7.4). As shown in Fig. 1, probe AETU-HOCl displayed a major absorption band at 440 nm. Then, addition of HOCl (5 μM) to the solution of probe AETU-HOCl resulted in a remarkable decrease of the absorbance at 440 nm, and appearance of a new absorption band at 376 nm. The blue-shifted absorption spectrum induced by HOCl might be attributed to the formation of an imidazoline moiety with a much less electron-donating group (Scheme 1).15
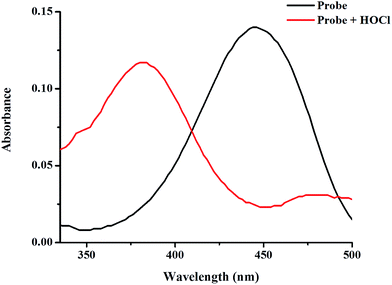 |
| Fig. 1 Absorption spectra of probe AETU-HOCl (5 μM) in the absence and presence of HOCl (5 μM) in the mixture of ethanol and ultrapure water (5 : 5, v/v) containing (5 mM PBS, pH 7.4). | |
3.2. Fluorescent response of probe AETU-HOCl to HOCl
In the fluorescent spectra, as expected, probe AETU-HOCl exhibited ratiometric changes upon the addition of increasing concentrations of HOCl under the above-mentioned analytical conditions. As shown in Fig. 2, the fluorescent intensity of the emission band centered at 533 nm was gradually decreased with the concurrent appearance of a new blue-shifted emission maximum at 473 nm. Notably, there was a good linearity between the ratio of emission intensities (I473/I533) and concentrations of HOCl in the range of 0–800 nM (Fig. 3). The limit of detection (LOD) of HOCl was calculated to be 3.9 nM (3σ/slope). The result makes it sufficiently sensitive for application in living systems.
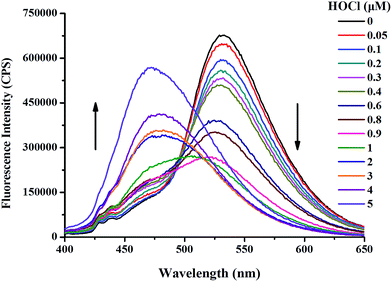 |
| Fig. 2 Fluorescence spectra changes of probe AETU-HOCl (5 μM) in the presence of increasing concentrations of HOCl (0–5 μM) in the mixture of ethanol and ultrapure water (5 : 5, v/v) containing (5 mM PBS, pH 7.4). The excitation wavelength was 380 nm. Excitation and emission slit widths were 3 nm. | |
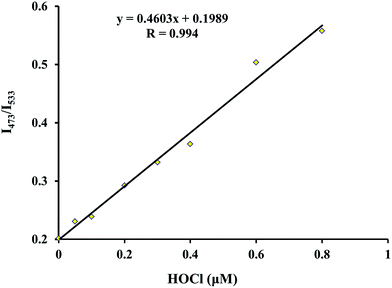 |
| Fig. 3 The linearity between the ratio of fluorescence intensities (I473/I533) and increasing concentrations of HOCl. | |
Reaction time is a significant factor for reaction-based probes, so the reaction time of probe AETU-HOCl with HOCl was investigated. As shown in Fig. 4, the fluorescence intensity increased with reaction time and then almost levels off at reaction time greater than about 1 min. The result exhibited that probe AETU-HOCl could provide a fast assay of HOCl.
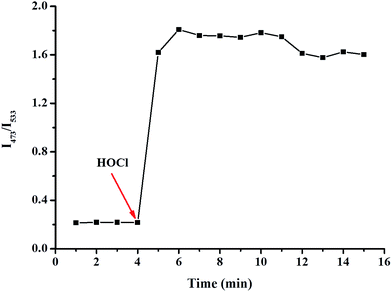 |
| Fig. 4 Time-course for probe AETU-HOCl (5 μM) in the presence of HOCl (5 μM). | |
Consequently, probe AETU-HOCl can be successfully constructed as a ratiometric fluorescent probe for HOCl, which allows for fast and ultrasensitive measurements (Table 1).
Table 1 Comparison of fluorescent probes for HOCl
Probe |
Ratiometric detection |
Quantitative range (μM) |
LOD (nM) |
Selectivity |
References |
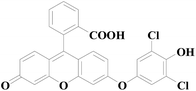 |
No |
0–10 |
0.33 |
10 equiv. |
8a |
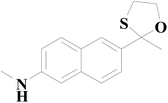 |
No |
0–100 |
16.6 |
5 equiv. |
11a |
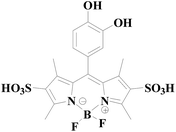 |
No |
2–20 |
300 |
2.5 equiv. |
12e |
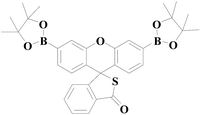 |
No |
0.2–20 |
— |
5 equiv. |
13a |
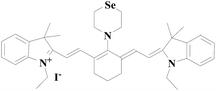 |
No |
0–60 |
310 |
10 equiv. |
7h |
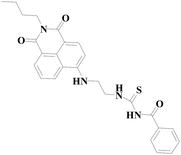 |
Yes |
0–0.8 |
3.9 |
100 equiv. |
This work |
3.3. Selectivity studies
Then we evaluated the selectivity of probe AETU-HOCl towards HOCl. Firstly, probe AETU-HOCl was treated with other potentially competing ROS/RNS to examine the selectivity. As shown in Fig. 5, nearly no fluorescence intensity changes were observed in the presence of H2O2, tert-butylhydroperoxide (TBHP), tert-butoxy radical (˙OtBu), hydroxyl radical (˙OH), peroxynitrite (ONOO−), O2−, 1O2, NO, NO2−, NO3−. In addition, the fluorescence responses of probe AETU-HOCl to a variety of metal ions and bio-reductants were performed. The results showed that K+, Ca2+, Na+, Mg2+, Al3+, Zn2+, glutathione (GSH), cysteine (Cys) and ascorbic acid exert no obvious spectral changes (Fig. 5). It is necessary to point out that 1 equiv. of HOCl resulted in a considerable fluorescence enhancement, while 100 equiv. (500 μM) of other bioanalytes only triggered a negligible increase. On the other hand, even though (2-amino-ethyl)-thiourea group as the receptor of Hg2+ was investigated in the previous paper,15 the concentration of Hg2+ in biosystems is negligible.7c Therefore, the above-mentioned results clearly demonstrated that probe AETU-HOCl possesses the excellent selectivity for HOCl in living systems (Table 1).
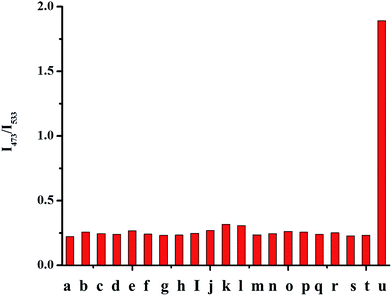 |
| Fig. 5 The fluorescence responses of probe AETU-HOCl (5 μM) in the presence of different analytes (0.5 mM except for specific label) in the mixture of ethanol and ultrapure water (5 : 5, v/v) containing (5 mM PBS, pH 7.4). (a) Only probe, (b) K+, (c) Ca2+, (d) Na+, (e) Mg2+, (f) Al3+, (g) Zn2+, (h) H2O2, (i) O2−, (j) TBHP, (k) ˙OH, (l) ˙OtBu, (m) NO, (n) 1O2, (o): ascorbic acid, (p) NO3−, (q) NO2−, (r) Cys (3 mM), (s) GSH (3 mM), (t) ONOO−, (u) HOCl (5 μM). Bars represent the ratio of fluorescence intensities (I473/I533). | |
3.4. The effect of pH
Probe AETU-HOCl in different pH values ranging from 3 to 8.5 exhibits almost no distinct variations, suggesting that probe AETU-HOCl is stable under the measurement conditions. The HOCl-sensing ability of probe AETU-HOCl under the different pH values was also evaluated (Fig. 6). The experimental results exhibited that probe AETU-HOCl could detect HOCl in a wide range of pH value, and importantly, it responded for HOCl rather than OCl− because hypochloric species almost existed in the only one formation of HOCl under the strong acidic conditions (pKa of 7.6 for HOCl).16
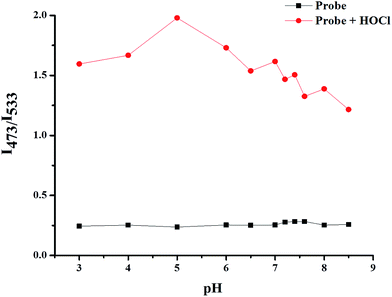 |
| Fig. 6 The ratio of fluorescence intensities (I473/I533) of probe AETU-HOCl (5 μM) in the absence and presence HOCl (5 μM) in different pH values. | |
3.5. Proposed mechanism for the reaction of probe AETU-HOCl with HOCl
To fully explore the sensing mechanism of probe AETU-HOCl for HOCl, we carried out the experiment on the reaction of AETU-HOCl and HOCl in ethanol. The blue fluorescence product was purified and characterized to be N-[(N-butyl-1,8-naphthalimide)-4-yl-2,3-dihydro-1H-imidazol-2-yl]benzamide by FT-IR, 1H-NMR and HRMS. Additionally, the mixture of probe AETU-HOCl and HOCl under the above-mentioned analytical conditions were subjected to electrospray ionization mass spectral analysis. The mass spectral peak at m/z 441.1933 [M + H]+ corresponding to imidazole product N-[(N-butyl-1,8-naphthalimide)-4-yl-2,3-dihydro-1H-imidazol-2-yl]benzamide was obtained. The fluorescence spectra of AETU-HOCl induced by HOCl also agreed with the fluorescence spectrum of N-[(N-butyl-1,8-naphthalimide)-4-yl-2,3-dihydro-1H-imidazol-2-yl]benzamide (Fig. 7). Finally, the titration experiment of AETU-HOCl with HOCl by 1H-NMR was further performed (Fig. 8). Therefore, consulted with the previous report,15 probe AETU-HOCl most likely undergoes the proposed mechanism as shown in Scheme 1.
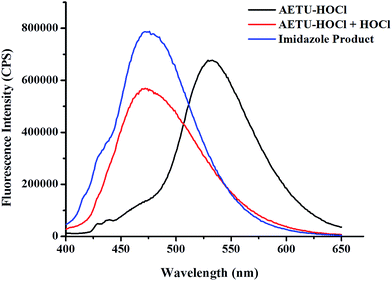 |
| Fig. 7 The fluorescence spectra of probe AETU-HOCl, AETU-HOCl with HOCl, and imidazole product N-[(N-butyl-1,8-naphthalimide)-4-yl-2,3-dihydro-1H-imidazol-2-yl]benzamide in the mixture of ethanol and ultrapure water (5 : 5, v/v) containing (5 mM PBS, pH 7.4). | |
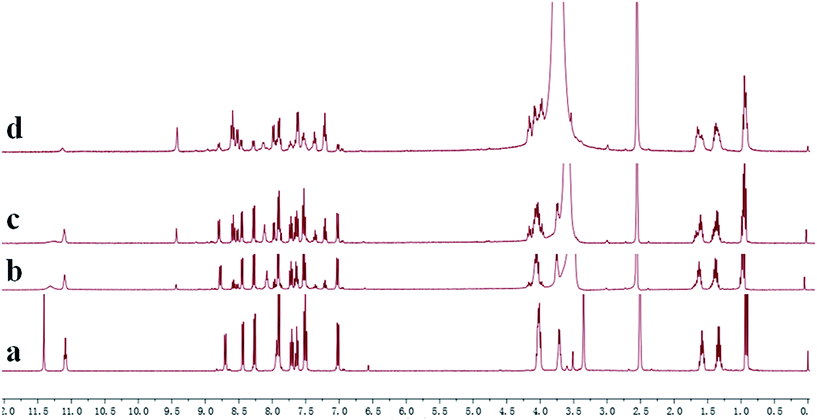 |
| Fig. 8 The 1H NMR spectra of probe AETU-HOCl in DMSO-d6 under the absence and presence of HOCl. (a) Free probe AETU-HOCl, (b) probe AETU-HOCl with 0.5 equiv. HOCl, (c) probe AETU-HOCl with 1.0 equiv. HOCl, (d) probe AETU-HOCl with 2.0 equiv. HOCl. | |
3.6. Biological applications
In consideration of excellent performances of probe AETU-HOCl in vitro, we then applied it to RAW 264.7 macrophage cells for examining its capability to image HOCl levels in living cells. As shown in Fig. 9, an intense intracellular fluorescence of the control cells incubated with probe AETU-HOCl (10 μM) for 30 min demonstrated that probe AETU-HOCl is cell-permeable (Fig. 9b and c). As expected, the bigger ratiometric measurement generated from blue channel and green channel in living cells pretreated with PMA (2.0 μg mL−1) for 1 h was observed. These results demonstrated that probe AETU-HOCl could be used for the ratiometric fluorescence imaging of endogenous HOCl in living systems.
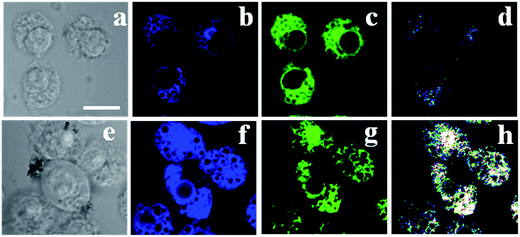 |
| Fig. 9 Confocal fluorescence images of living RAW 264.7 macrophage cells: the control cells were incubated with probe AETU-HOCl (10 μM) for 30 min; (a) bright-field transmission image, (b) blue channel, (c) green channel, and (d) ratio image generated from (b) and (c). The cells pretreated with PMA (2.0 μg mL−1) for 1 h were incubated with probe AETU-HOCl (10 μM) for another 30 min; (e) bright-field transmission image, (f) blue channel, (g) orange channel, and (h) ratio image generated from (f) and (g). Incubation was performed at 37 °C under a humidified atmosphere containing 5% CO2. Scale bar = 10 μm. | |
Furthermore, to evaluate cytotoxicity of probe AETU-HOCl, we performed 3-(4,5-dimethylthiazol-2-yl)-2,5-diphenyltetrazolium bromide (MTT) assays in RAW 264.7 macrophage cells with 5, 10, 20, and 30 μM probe AETU-HOCl for 24 h. As can be seen from Fig. 10, cell viabilities were over 90%, implying that probe AETU-HOCl was of low toxicity to cultured cells under the experimental conditions.
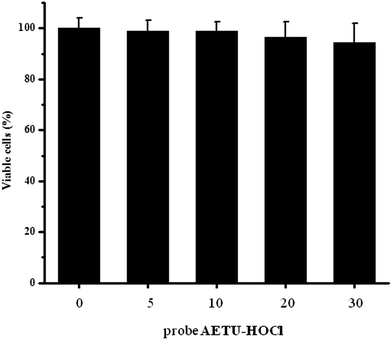 |
| Fig. 10 Cytotoxicity assays of probe AETU-HOCl at different concentrations for RAW 264.7 cells. | |
4. Conclusions
In summary, a new ratiometric fluorescent probe AETU-HOCl for HOCl has been designed and synthesized. The experimental results demonstrated that probe AETU-HOCl possesses outstanding selectivity toward HOCl over other ROS, RNS, bio-reductants and metal ions at higher concentrations (0.5 mM). Probe AETU-HOCl could detect HOCl in the range of 0 to 800 nM with the detection limit of 3.9 nM by the ratiometric fluorescence spectra. Additionally, probe AETU-HOCl showed a rapid response (about 1 min) to HOCl at a wide range of pH. Importantly, probe AETU-HOCl could be successfully used for the ratiometric fluorescence imaging of endogenous HOCl in living cells. The excellent sensing features of probe AETU-HOCl make it a promising tool for detecting HOCl in living cells. Our proposed recognition mechanism would open up new exciting opportunities for designing highly selective and ultrasensitive fluorescent probes for the ratiometric determination of HOCl in living systems.
Acknowledgements
We gratefully acknowledge financial support from the National Natural Science Foundation of China (No. 21107029), Outstanding Young Scientists Award Fund of Shandong Province (BS2013HZ007), Postdoctoral Science Foundation of China (2013M541953, 2016M592127), Graduate Innovation Foundation of University of Jinan (YCXS15016) for financial support.
References
-
(a) S. L. Hazen, F. F. Hsu, K. Duffin and J. W. Heinecke, J. Biol. Chem., 1996, 271, 23080 CrossRef CAS PubMed;
(b) Z. M. Prokopowicz, F. Arce, R. Biedroń, C. L. Chiang, M. Ciszek, D. R. Katz, M. Nowakowska, S. Zapotoczny, J. Marcinkiewicz and B. M. Chain, J. Immunol., 2010, 184, 824 CrossRef CAS PubMed.
-
(a) J. E. Harrison and J. Schultz, J. Biol. Chem., 1976, 251, 1371 CAS;
(b) T. J. Fiedler, C. A. Davey and R. E. Fenna, J. Biol. Chem., 2000, 275, 11964 CrossRef CAS PubMed.
- A. Daugherty, J. L. Dunn, D. L. Rateri and J. W. Heinecke, J. Clin. Invest., 1994, 94, 437 CrossRef CAS PubMed.
- S. Hammerschmidt, N. Büchler and H. Wahn, Chest, 2002, 121, 573 CrossRef CAS PubMed.
- T. Hasegawa, E. Malle, A. Farhood and H. Jaeschke, Am. J. Physiol.: Gastrointest. Liver Physiol., 2005, 289, 760 Search PubMed.
- S. M. Wu and S. V. Pizzo, Arch. Biochem. Biophys., 2001, 391, 119 CrossRef CAS PubMed.
-
(a) M. T. Sun, H. Yu, H. J. Zhu, F. Ma, S. Zhang, D. J. Huang and S. H. Wang, Anal. Chem., 2014, 86, 671 CrossRef CAS PubMed;
(b) H. Zhu, J. L. Fan, J. Y. Wang, H. Y. Mu and X. J. Peng, J. Am. Chem. Soc., 2014, 136, 12820 CrossRef CAS PubMed;
(c) J. Zhou, L. H. Li, W. Shi, X. H. Gao, X. H. Li and H. M. Ma, Chem. Sci., 2015, 6, 4884 RSC;
(d) G. H. Cheng, J. L. Fan, W. Sun, K. Sui, X. Jin, J. Y. Wang and X. J. Peng, Analyst, 2013, 138, 2986 Search PubMed;
(e) Y. R. Zhang, X. P. Chen, J. Shao, J. Y. Zhang, Q. Yuan, J. Y. Miao and B. X. Zhao, Chem. Commun., 2014, 50, 14241 CAS;
(f) Y. K. Yue, F. J. Huo, C. X. Yin, J. O. Escobedoc and R. M. Strongin, Analyst, 2016, 141, 1859 RSC;
(g) Q. L. Xu, C. H. Heo, G. Kim, H. W. Lee, H. M. Kim and J. Yoon, Angew. Chem., Int. Ed., 2015, 54, 4890 CrossRef CAS PubMed;
(h) G. H. Cheng, J. L. Fan, W. Sun, J. F. Cao, C. Hu and X. J. Peng, Chem. Commun., 2014, 50, 1018 RSC.
-
(a) J. J. Hu, N. K. Wong, M. Y. Lu, X. m. Chen, S. Ye, A. Q. Zhao, P. Gao, R. Y. Kao, J. G. Shen and D. Yang, Chem. Sci., 2016, 7, 2094 RSC;
(b) Z. R. Lou, P. Li, P. Song and K. L. Han, Analyst, 2013, 138, 6291 RSC;
(c) W. Shu, L. G. Yan, Z. K. Wang, J. Liu, S. Zhang, C. Y. Liu and B. C. Zhu, Sens. Actuators, B, 2015, 221, 1130 CrossRef CAS;
(d) W. Zhang, W. Liu, P. Li, J. Q. Kang, J. Y. Wang, H. Wang and B. Tang, Chem. Commun., 2015, 51, 10150 RSC;
(e) W. Z. Yin, H. J. Zhu and R. Y. Wang, Dyes Pigm., 2014, 107, 127 CrossRef CAS.
-
(a) S. R. Liu and S. P. Wu, Org. Lett., 2013, 15, 878 CrossRef CAS PubMed;
(b) X. Q. Zhan, J. H. Yana, J. H. Sua, Y. C. Wang, J. He, S. Y. Wang, H. Zheng and J. G. Xu, Sens. Actuators, B, 2010, 150, 774 CrossRef CAS;
(c) S. Y. Yu, C. Y. Hsu, W. C. Chen, L. F. Wei and S. P. Wu, Sens. Actuators, B, 2014, 196, 203 CrossRef CAS;
(d) Q. Q. Wang, L. Y. Zhou, L. P. Qiu, D. Q. Lu, Y. X. Wu and X. B. Zhang, Analyst, 2015, 140, 5563 RSC;
(e) X. Y. Zhu, M. Y. Xiong, H. W. Liu, G. J. Mao, L. Y. Zhou, J. Zhang, X. X. Hu, X. B. Zhang and W. H. Tan, Chem. Commun., 2016, 52, 733 RSC;
(f) X. Feng, T. Zhang, J. T. Liu, J. Y. Miao and B. X. Zhao, Chem. Commun., 2016, 52, 3131 RSC;
(g) X. Y. Han, F. B. Yu, X. Y. Song and L. X. Chen, Chem. Sci., 2016, 52 10.1039/C6SC00838K.
-
(a) L. Yuan, W. Lin, Y. Xie, B. Chen and J. Song, Chem.–Eur. J., 2012, 18, 2700 CrossRef CAS PubMed;
(b) Q. A. Best, N. Sattenapally, D. J. Dyer, C. N. Scott and M. E. McCarroll, J. Am. Chem. Soc., 2013, 135, 13365 CrossRef CAS PubMed.
-
(a) L. Yuan, L. Wang, B. K. Agrawalla, S. J. Park, H. Zhu, B. Sivaraman, J. J. Peng, Q. H. Xu and Y. T. Chang, J. Am. Chem. Soc., 2015, 137, 5930 CrossRef CAS PubMed;
(b) J. Hwang, M. G. Choi, J. Bae and S. K. Chang, Org. Biomol. Chem., 2011, 9, 7011 RSC;
(c) X. J. Wu, Z. Li, L. Yang, J. H. Han and S. F. Han, Chem. Sci., 2013, 4, 460 RSC.
-
(a) S. R. Liu, M. Vedamalai and S. P. Wu, Anal. Chim. Acta, 2013, 800, 71 CrossRef CAS PubMed;
(b) Y. X. Liao, M. D. Wang, K. Li, Z. X. Yang, J. T. Hou, M. Y. Wu, Y. H. Liu and X. Q. Yu, RSC Adv., 2015, 5, 18275 RSC;
(c) F. N. Lu and T. Nabeshima, Dalton Trans., 2014, 43, 9529 RSC;
(d) Y. Zhou, J. Y. Li, K. H. Chu, K. Liu, C. Yao and J. Y. Li, Chem. Commun., 2012, 48, 4677 RSC;
(e) J. Y. Kim and Y. M. Kim, Analyst, 2014, 139, 2986 RSC.
-
(a) B. C. Zhu, X. L. Zhang, H. Y. Jia, Y. M. Li, H. P. Liu and W. H. Tan, Org. Biomol. Chem., 2010, 8, 1650 RSC;
(b) B. C. Zhu, X. L. Zhang, Y. M. Li, P. F. Wang, H. Y. Zhang and X. Q. Zhuang, Chem. Commun., 2010, 46, 5710 RSC;
(c) X. D. Zeng, X. L. Zhang, B. C. Zhu, H. Y. Jia, Y. M. Li and J. Xue, Analyst, 2011, 136, 4008 RSC.
- J. C. Morris, J. Phys. Chem., 1966, 70, 3798 CrossRef CAS.
- B. Liu and H. Tian, Chem. Commun., 2005, 3156 RSC.
- S. Kenmoku, Y. Urano, H. Kojima and T. Nagano, J. Am. Chem. Soc., 2007, 129, 7313 CrossRef CAS PubMed.
|
This journal is © The Royal Society of Chemistry 2016 |
Click here to see how this site uses Cookies. View our privacy policy here.