DOI:
10.1039/C6RA13985J
(Paper)
RSC Adv., 2016,
6, 61680-61685
Synthesis of functionalized tetrahydropyridazines via catalyst-free self [4 + 2] cycloaddition of in situ generated 1,2-diaza-1,3-dienes†
Received
30th May 2016
, Accepted 18th June 2016
First published on 21st June 2016
Abstract
An efficient method for the synthesis of structurally diverse functionalized tetrahydropyridazines via self [4 + 2] cycloaddition of in situ generated 1,2-diaza-1,3-dienes was developed, which may play an important role in drug discovery.
Introduction
The synthesis of diverse nitrogen-containing heterocyclic compounds has received wide interest due to their important applications and special activities in medicinal chemistry and related sciences.1 Among them, tetrahydropyridazines represent one of the important building blocks found in a variety of bioactive and natural compounds (Fig. 1).2 Antrimycin, which was isolated from a soil sample, has been shown to have antibacterial activity against Mycobacterium smegmatis ATCC 607.3 Quinoxapeptin A and B isolated from a nocardioform actinomycete with indeterminate morphology are potent inhibitors of HIV-1 and HIV-2 reverse transcriptase.4 Tetrahydropyridazines can also be found in lots of synthetic pharmaceutical compounds, for instance 3-aryl-1-benzoxyl-1,4,5,6-tetrahydropyridazines have been identified as nonsteroidal progesterone receptor ligands (e.g. I),5 3-aryl-1-(arylsulfonyl)-1,4,5,6-tetrahydropyridazines are a class of nonsteroidal allosteric modulators at the GABAA receptor (e.g. II).6 As a result, the distinctive structures and medicinal potential of molecules containing tetrahydropyridazines have become popular targets in target-oriented synthesis.7,8 Among these well known methods, the hetero Diels–Alder reaction of 1,2-diaza-1,3-dienes generated in situ from hydrazones with alkenes has proved to be an attractive strategy for the construction of structurally diverse tetrahydropyridazines.7 However, such reactions are limited to electron-rich or electron-neutral alkenes and electron-deficient or in situ generated alkenes remain challenging substrates.7n So we want to explore these substrates considering the importance of structurally diverse functionalized tetrahydropyridazines. To our surprise, the 6-diazenyl substituted tetrahydropyridazine formed via self [4 + 2] cycloaddition of in situ generated 1,2-diaza-1,3-dienes is the only product even in the presence of methyl acrylate or methyl buta-2,3-dienoate under various conditions (Scheme 1). It should be noted that the substituted tetrahydropyridazine derivative with a diazenyl substituent is not easily accessed by known methods, and could be potentially be useful in medicinal science. Herein, we report our systematic study on the self [4 + 2] cycloaddition of in situ generated 1,2-diaza-1,3-dienes under catalyst free conditions to construct diverse 6-diazenyl substituted tetrahydropyridazine derivatives.9
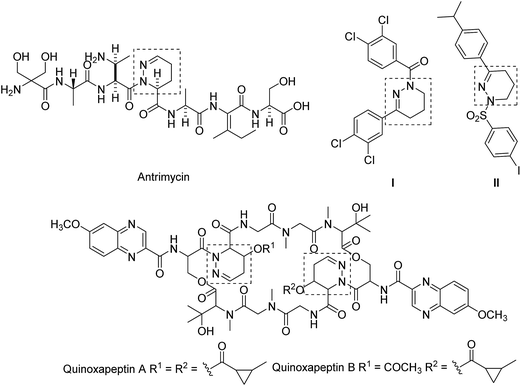 |
| Fig. 1 Examples of tetrahydropyridazine derivatives in natural products and bioactive compounds. | |
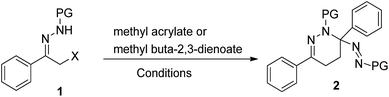 |
| Scheme 1 Synthesis of tetrahydropyridazines. | |
Results and discussion
Our study commenced with the self [4 + 2] cycloaddition reaction of α-chloro-N-acylhydrazones (1) in the presence of different bases, solvents and reaction time to optimize the reaction conditions. To our delight, the reaction proceeded successfully via dimerization of the in situ generated 1,2-diaza-1,3-butadiene to provide product 2a in 18% yield when it was conducted in CH2Cl2 with DMAP (1.0 equiv.) at room temperature without any catalyst (Table 1, entry 1). A series of bases were then screened. Organic bases, such as DIPEA and Et3N, were ineffective (Table 1, entry 2–4). While the use of inorganic bases resulted in an improvement of the yield (Table 1, entries 5–11) and K2CO3 was shown to be the most effective in this reaction. Based on this encouraging result, we evaluated several other solvents, but none of these performed any better than CH2Cl2 (Table 1, entries 12–19). Next, we examined the influence of the reaction time on the model reaction and we found that 1.0 h was still the most suitable time for the reaction (Table 1, entries 20–22). However, no desired products were obtained when the benzoyl group of 1 was replaced by phenyl or benzenesulfonyl group (Table 1, entries 23 and 24). Further investigation toward the influence of leaving group revealed that bromine as the leaving group gave the best yield, whereas tosyl was ineffective (Table 1, entries 25 and 27).
Table 1 Optimization of the reaction conditionsa
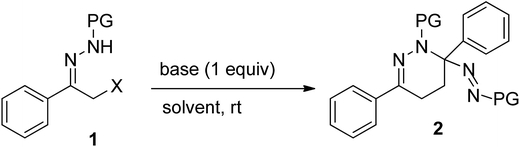
|
Entry |
PG |
X |
Base |
Solvent |
Product |
Yieldb |
The reaction was carried out with 1 (0.5 mmol) and base (1.0 equiv.) at room temperature under an atmosphere of air for 1.0 h. Isolated yields. 15 min. 30 min. 1.5 h. |
1 |
PhCO |
Cl |
DMAP |
CH2Cl2 |
2a |
18 |
2 |
PhCO |
Cl |
Pyridine |
CH2Cl2 |
2a |
12 |
3 |
PhCO |
Cl |
Et3N |
CH2Cl2 |
2a |
Trace |
4 |
PhCO |
Cl |
DIPEA |
CH2Cl2 |
2a |
Trace |
5 |
PhCO |
Cl |
Na2CO3 |
CH2Cl2 |
2a |
57 |
6 |
PhCO |
Cl |
NaHCO3 |
CH2Cl2 |
2a |
44 |
7 |
PhCO |
Cl |
NaOH |
CH2Cl2 |
2a |
11 |
8 |
PhCO |
Cl |
K2CO3 |
CH2Cl2 |
2a |
89 |
9 |
PhCO |
Cl |
Cs2CO3 |
CH2Cl2 |
2a |
50 |
10 |
PhCO |
Cl |
AcOK |
CH2Cl2 |
2a |
32 |
11 |
PhCO |
Cl |
K3PO4 |
CH2Cl2 |
2a |
81 |
12 |
PhCO |
Cl |
K2CO3 |
AcOEt |
2a |
38 |
13 |
PhCO |
Cl |
K2CO3 |
CH3OH |
2a |
Trace |
14 |
PhCO |
Cl |
K2CO3 |
THF |
2a |
19 |
15 |
PhCO |
Cl |
K2CO3 |
DMF |
2a |
Trace |
16 |
PhCO |
Cl |
K2CO3 |
CH3CN |
2a |
21 |
17 |
PhCO |
Cl |
K2CO3 |
(CH2)2Cl2 |
2a |
45 |
18 |
PhCO |
Cl |
K2CO3 |
Toluene |
2a |
62 |
19 |
PhCO |
Cl |
K2CO3 |
1,4-Dioxane |
2a |
Trace |
20c |
PhCO |
Cl |
K2CO3 |
CH2Cl2 |
2a |
33 |
21d |
PhCO |
Cl |
K2CO3 |
CH2Cl2 |
2a |
57 |
22e |
PhCO |
Cl |
K2CO3 |
CH2Cl2 |
2a |
85 |
23 |
Ph |
Cl |
K2CO3 |
CH2Cl2 |
— |
— |
24 |
PhSO2 |
Cl |
K2CO3 |
CH2Cl2 |
— |
— |
25 |
PhCO |
Br |
K2CO3 |
CH2Cl2 |
2a |
85 |
26 |
PhCO |
I |
K2CO3 |
CH2Cl2 |
2a |
63 |
27 |
PhCO |
Tosyl |
K2CO3 |
CH2Cl2 |
— |
— |
With the optimized conditions in hand, the generality and scope of the catalyst-free self [4 + 2] cycloaddition reaction were explored. A variety of α-chloro-N-acylhydrazones bearing electron-donating or -withdrawing groups were subjected to the standard conditions (Table 2, entries 1–16), most of them resulting in the expected functionalized tetrahydropyridazines in good to excellent yields. It is noteworthy that the reaction demonstrated wide tolerance for diverse substituents. Benzoyl hydrazones bearing electron-neutral (4-H), electron-rich (e.g. 4-Me, 4-OMe), and electron-deficient (e.g. 4-Cl) phenyl rings were successfully converted to the corresponding products 2a–d in good yields (83–92%; Table 2, entries 1–4). Furthermore, the hydrazone substrate with a thiophene skeleton afforded the target heterocycles in 95% yield. Gratifyingly, the naphthalene attached tetrahydropyridazines could be obtained in 98% yield. Regrettably, the reaction of nonaromatic acylhydrazones under standard conditions led to complicated mixtures, and a trace amount of the products were obtained (Table 2, entries 7–9). The compatibility and generality of the present method were further explored by variations of the R1 group. Halogen substituents on the R1 benzene group were tested. Thus, 4-chlorobenzene and 4-fluorobenzene reacted and gave products 2j–n in 89–92% yields. In addition, styrene or alkyl substituted hydrazones did not work well, leading to no desired product (Table 2, entry 15 and 16).
Table 2 Synthesis of structurally diverse functionalized tetrahydropyridazinesa
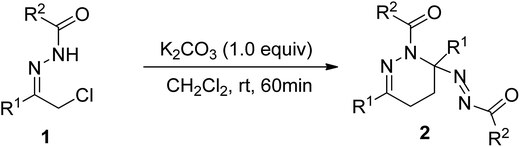
|
Entry |
R1 |
R2 |
2 |
Yieldb [%] |
The reaction was carried out with 1 (0.5 mmol) and K2CO3 (1.0 equiv.) at room temperature under an atmosphere of air for 1.0 h. Isolated yields. |
1 |
Ph |
Ph |
2a |
89 |
2 |
Ph |
4-MeOPh |
2b |
92 |
3 |
Ph |
4-MePh |
2c |
90 |
4 |
Ph |
4-ClPh |
2d |
83 |
5 |
Ph |
2-Thienyl |
2e |
95 |
6 |
Ph |
2-Naphthyl |
2f |
98 |
7 |
Ph |
Me |
2g |
Trace |
8 |
Ph |
OEt |
2h |
Trace |
9 |
Ph |
OtBu |
2i |
Trace |
10 |
4-ClPh |
4-MeOPh |
2j |
90 |
11 |
4-ClPh |
4-MePh |
2k |
90 |
12 |
4-FPh |
Ph |
2l |
89 |
13 |
4-FPh |
4-MeOPh |
2m |
91 |
14 |
4-FPh |
2-Thienyl |
2n |
92 |
15 |
 |
Ph |
— |
NR |
16 |
tBu |
Ph |
— |
NR |
To further expand the substrate scope, we next tested other α-chloro-N-acylhydrazones. As shown in Scheme 2, when 3 was treated under standard conditions, instead of the desired self [4 + 2] cycloaddition, an eliminated product 4 was obtained in 92% yield. Notably, a novel diazenyl substituted tetrahydropyridazine derivative 6 was obtained in 87% yield when α-chloro-N-acylhydrazone 5 derived from 2-chloro-3,4-dihydronaphthalen-1(2H)-one was used under standard conditions even though a longer reaction time was needed to completely consume 5.
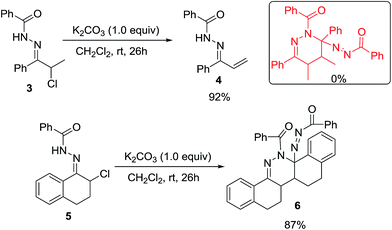 |
| Scheme 2 Substrate scope for hydrazones. | |
Next, the functionalized tetrahydropyridazine derivative 2a could also be successfully converted to a pharmaceutically important tetrahydropyridazine compound 7 in 86% yield. Treatment of 2a with Pd/C under H2 atmosphere in methanol led to the reduced and ring-opened product N′-(1,4-diphenylbutyl)benzohydrazide in 82% yield (Scheme 3). The developed cycloaddition could be performed on a gram scale. The reaction of α-chloro-N-benzoyl hydrazone 1 afforded 2a (1.03 g) in 87% yield (Scheme 4).
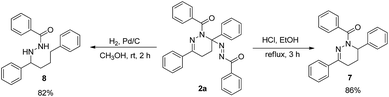 |
| Scheme 3 Transformation of 2a. | |
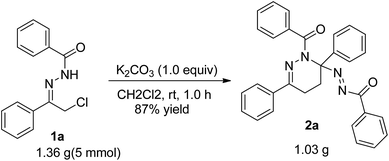 |
| Scheme 4 Synthesis of 2a on a gram scale. | |
Experimental
Unless otherwise noted, materials were purchased from commercial suppliers and used without further purification. All reactions were performed in anhydrous solvents. Reactions were monitored by thin layer chromatography (TLC), and column chromatography purifications were performed using 200–300 mesh silica gel. Melting points were obtained on a melting point apparatus and are uncorrected. 1H and 13C NMR spectra were recorded in DMSO-d6 or CDCl3 using a 300 MHz spectrometer. Chemical shifts are reported in delta (δ) units in parts per million (ppm) relative to the singlet (0 ppm) for tetramethylsilane (TMS). High-resolution mass spectra were recorded in ESI mode on a QTOF MS spectrometer. The α-chloro-N-acylhydrazones were prepared according to literature procedures.10
General procedure for the synthesis of structurally diverse functionalized tetrahydropyridazines
To the solution of α-chloro-N-acylhydrazones (0.5 mmol) in dry CH2Cl2 (5 mL) was added K2CO3 (69.1 mg, 0.5 mmol). The resulting mixture was stirred at room temperature for the required period of time. After completion of the reaction as monitored by TLC, the reaction mixture was diluted with 15 mL CH2Cl2, which was washed with water and brine successively, dried over MgSO4, filtered, and concentrated in vacuo. Purification by flash chromatography (ethyl acetate/PE, 1
:
20–1
:
5) yielded the desired products.
((2-Benzoyl-3,6-diphenyl-2,3,4,5-tetrahydropyridazin-3-yl)diazenyl)(phenyl)methanone (2a). Yellow solid (105.2 mg, 89% yield); mp = 122–124 °C; 1H NMR (300 MHz, CDCl3) δ 8.01 (d, J = 7.3 Hz, 2H), 7.89–7.81 (m, 2H), 7.76 (d, J = 7.4 Hz, 2H), 7.69–7.53 (m, 2H), 7.45–7.41 (m, J = 14.6, 13.1, 7.7 Hz, 8H), 7.36–7.27 (m, 4H), 2.92–2.70 (m, 2H), 2.37 (m, 2H), ppm; 13C NMR (75 MHz, CDCl3) δ 182.66, 169.48, 145.24, 141.96, 136.01, 134.79, 134.31, 130.41, 130.23, 129.59, 128.87, 128.60, 128.49, 128.43, 127.97, 127.16, 126.95, 125.03, 124.25, 86.14, 31.72, 17.68, ppm; HRMS (ESI) calcd for C30H24O2N4Na+ (M + Na)+ 495.6174, found 495.6177.
((2-(4-Methoxybenzoyl)-3,6-diphenyl-2,3,4,5-tetrahydropyridazin-3-yl)diazenyl)(4-methoxyphenyl)methanone (2b). Yellow solid (122.5 mg, 92% yield); mp = 135–137 °C; 1H NMR (300 MHz, CDCl3) δ 7.97 (t, J = 5.8 Hz, 2H), 7.90 (d, J = 8.9 Hz, 2H), 7.78–7.70 (m, 2H), 7.67–7.57 (m, 2H), 7.43–7.27 (m, 6H), 6.95 (m, 4H), 3.87 (t, J = 5.7 Hz, 6H), 2.93–2.65 (m, 2H), 2.50–2.21 (m, 2H), ppm; 13C NMR (75 MHz, CDCl3) δ 182.38, 169.18, 164.84, 161.78, 145.28, 142.78, 136.72, 133.34, 132.59, 132.26, 129.22, 128.83, 128.45, 127.50, 125.53, 124.77, 121.74, 114.33, 112.75, 86.42, 55.53, 55.38, 32.19, 18.26, ppm; HRMS (ESI) calcd for C32H28O4N4Na+ (M + Na)+ 555.6257, found 555.6251.
((2-(4-Methylbenzoyl)-3,6-diphenyl-2,3,4,5-tetrahydropyridazin-3-yl)diazenyl)(p-tolyl)methanone (2c). Yellow solid (112.6 mg, 90% yield); mp = 116–118 °C; 1H NMR (300 MHz, DMSO) δ 7.90–7.80 (m, 4H), 7.72 (d, J = 7.7 Hz, 4H), 7.59 (d, J = 6.3 Hz, 4H), 7.36 (s, 5H), 7.29 (s, 1H), 2.73 (dd, J = 48.2, 28.5 Hz, 2H), 2.40 (s, 3H), 2.37 (s, 3H), 2.25–1.89 (m, 2H), ppm; 13C NMR (75 MHz, CDCl3) δ 183.00, 169.69, 145.86, 145.38, 142.65, 141.04, 135.68, 132.27, 130.97, 130.37, 130.20, 129.65, 129.19, 128.80, 128.40, 128.07, 127.48, 125.52, 124.73, 86.48, 32.20, 21.89, 21.55, 18.21, ppm; HRMS (ESI) calcd for C32H28O2N4Na+ (M + Na)+ 0.523.4922, found 523.4919.
((2-(4-Chlorobenzoyl)-3,6-diphenyl-2,3,4,5-tetrahydropyridazin-3-yl)diazenyl)(4-chlorophenyl)methanone (2d). Yellow solid (112.1 mg, 83% yield); mp = 130–132 °C; 1H NMR (300 MHz, CDCl3) δ 7.97–7.91 (m, 2H), 7.81–7.75 (m, 2H), 7.74–7.68 (m, 2H), 7.57 (dd, J = 6.7, 3.0 Hz, 2H), 7.47–7.33 (m, 10H), 2.80 (m, 2H), 2.37 (m, 2H), ppm; 13C NMR (75 MHz, CDCl3) δ 182.00, 168.89, 146.44, 142.03, 141.61, 136.93, 136.23, 133.53, 132.16, 131.56, 129.58, 129.41, 128.96, 128.54, 127.80, 127.75, 127.43, 125.49, 124.65, 86.82, 32.19, 18.23, ppm; HRMS (ESI) calcd for C30H22Cl2N4O2Na+ (M + Na)+ 563.0999, found 563.0992.
((3,6-Diphenyl-2-(thiophene-2-carbonyl)-2,3,4,5-tetrahydropyridazin-3-yl)diazenyl)(thiophen-2-yl)methanone (2e). Yellow solid (115.0 mg, 95% yield); mp = 160–162 °C; 1H NMR (300 MHz, CDCl3) δ 8.35–8.33 (m, 1H), 7.94–7.95 (m, 4H), 7.61 (m, 1H), 7.53–7.51 (m, 4H), 7.44–7.35 (m, 5H), 6.89 (m, 1H), 2.86–2.60 (m, 2H), 2.56–2.26 (m, 2H), ppm; 13C NMR (75 MHz, DMSO) δ 176.04, 160.30, 149.65, 142.27, 139.53, 137.94, 136.22, 136.07, 135.94, 132.88, 132.75, 129.75, 129.62, 128.75, 128.59, 127.32, 126.65, 126.61, 124.60, 86.64, 32.08, 18.79, ppm; HRMS (ESI) calcd for C26H20N4O2S2Na+ (M + Na)+ 507.0898, found 507.0908.
((2-(2-Naphthoyl)-3,6-diphenyl-2,3,4,5-tetrahydropyridazin-3-yl)diazenyl)(naphthalen-2-yl)methanone (2f). Yellow solid (140.4 mg, 98% yield); mp = 198–200 °C; 1H NMR (300 MHz, CDCl3) δ 8.58 (s, 1H), 8.40 (s, 1H), 8.10 (dd, J = 8.6, 1.6 Hz, 1H), 8.00 (dd, J = 8.6, 1.5 Hz, 1H), 7.95 (s, 1H), 7.93–7.82 (m, 6H), 7.54–7.51 (m, 8H), 7.38–7.22 (m, 5H), 2.99–2.74 (m, 2H), 2.63–2.31 (m, 2H), ppm; 13C NMR (75 MHz, CDCl3) δ 182.99, 170.98, 145.76, 142.49, 136.46, 134.54, 132.03, 130.82, 130.26, 129.49, 129.36, 129.31, 128.90, 128.83, 128.56, 128.44, 128.26, 128.11, 127.78, 127.73, 127.65, 127.36, 126.96, 126.85, 126.72, 126.65, 126.24, 125.50, 125.40, 124.83, 124.23, 86.70, 29.67, 18.26, ppm; HRMS (ESI) calcd for C38H29O2N4+ (M + H)+ 573.1468, found 573.1456.
((3,6-Bis(4-chlorophenyl)-2-(4-methoxybenzoyl)-2,3,4,5-tetrahydropyridazin-3-yl)diazenyl)(4-methoxyphenyl)methanone (2j). Yellow solid (135.0 mg, 90% yield); mp = 107–109 °C; 1H NMR (300 MHz, CDCl3) δ 7.94 (d, J = 8.9 Hz, 2H), 7.86 (d, J = 8.8 Hz, 2H), 7.67 (d, J = 8.7 Hz, 2H), 7.53 (d, J = 8.7 Hz, 2H), 7.36 (d, J = 8.7 Hz, 2H), 7.30 (d, J = 8.7 Hz, 2H), 6.94 (dd, J = 8.8, 5.9 Hz, 4H), 3.87 (d, J = 3.0 Hz, 6H), 2.88–2.65 (m, 2H), 2.41–2.22 (m, 2H), ppm; 13C NMR (75 MHz, CDCl3) δ 182.09, 169.18, 164.93, 161.96, 144.27, 141.23, 135.28, 135.0, 133.33, 13.27, 132.49, 129.06, 128.65, 126.77, 126.14, 121.50, 114.36, 113.72, 112.82, 85.81, 55.54, 55.38, 31.84, 18.03, ppm; HRMS (ESI) calcd for C32H26Cl2N4O4Na+ (M + Na)+ 623.1213, found 623.1214.
((3,6-Bis(4-chlorophenyl)-2-(4-methylbenzoyl)-2,3,4,5-tetrahydropyridazin-3-yl)diazenyl)(p-tolyl)methanone (2k). Yellow solid (127.8 mg, 90% yield); mp = 97–99 °C; 1H NMR (300 MHz, CDCl3) δ 7.78 (d, J = 8.2 Hz, 2H), 7.66 (d, J = 8.1 Hz, 2H), 7.62–7.56 (m, 2H), 7.45–7.38 (m, 2H), 7.27 (d, J = 8.7 Hz, 2H), 7.21–7.13 (m, 6H), 2.81–2.51 (m, 2H), 2.33 (d, J = 2.1 Hz, 6H), 2.27–2.15 (m, 2H), ppm; 13C NMR (75 MHz, CDCl3) δ 182.72, 169.72, 146.08, 144.39, 141.41, 141.12, 135.30, 134.96, 133.36, 131.85, 130.90, 130.26, 129.97, 129.71, 129.24, 128.63, 128.16, 126.78, 126.12, 85.88, 31.87, 21.90, 21.55, 17.99, ppm; HRMS (ESI) calcd for C32H26Cl2N4O2Na+ (M + Na)+ 591.1312, found 593.1310.
((2-Benzoyl-3,6-bis(4-fluorophenyl)-2,3,4,5-tetrahydropyridazin-3-yl)diazenyl)(phenyl)methanone (2l). Yellow solid (113.1 mg, 89% yield); mp = 84–86 °C; 1H NMR (300 MHz, CDCl3) δ 8.02–7.95 (m, 2H), 7.84–7.77 (m, 2H), 7.73 (dd, J = 8.9, 5.2 Hz, 2H), 7.57–7.40 (m, 8H), 7.10 (t, J = 8.7 Hz, 2H), 7.00 (t, J = 8.7 Hz, 2H), 2.78 (m, 2H), 2.43–2.25 (m, 2H), ppm; 13C NMR (75 MHz, CDCl3) δ 182.88, 169.99, 165.13, 163.65, 160.38, 144.80, 138.10, 135.12, 134.83, 132.55, 131.26, 130.80, 129.88, 129.57, 128.97, 127.97, 127.66, 127.46, 127.44, 127.33, 126.54, 126.43, 126.27, 116.25, 115.96, 115.67, 115.56, 115.27, 86.05, 32.14, 18.15, ppm; HRMS (ESI) calcd for C30H22F2N4O2Na+ (M + Na)+ 531.1585, found 531.1590.
((3,6-Bis(4-fluorophenyl)-2-(4-methoxybenzoyl)-2,3,4,5-tetrahydropyridazin-3-yl)diazenyl)(4-methoxyphenyl)methanone (2m). Yellow solid (129.3 mg, 91% yield); mp = 135–137 °C; 1H NMR (300 MHz, CDCl3) δ 7.94 (d, J = 8.9 Hz, 2H), 7.86 (d, J = 8.8 Hz, 2H), 7.67 (d, J = 8.7 Hz, 2H), 7.53 (d, J = 8.7 Hz, 2H), 7.36 (d, J = 8.7 Hz, 2H), 7.30 (d, J = 8.7 Hz, 2H), 6.94 (dd, J = 8.8, 5.9 Hz, 4H), 3.87 (d, J = 3.0 Hz, 6H), 2.88–2.65 (m, 2H), 2.41–2.22 (m, 2H), ppm; 13C NMR (75 MHz, CDCl3) δ 182.18, 169.20, 165.06, 164.90, 163.57, 161.87, 161.75, 160.31, 144.34, 138.43, 133.27, 132.76, 132.45, 127.43, 127.32, 127.03, 126.53, 126.42, 121.57, 115.87, 115.59, 115.55, 115.26, 114.33, 112.78, 85.82, 55.52, 55.36, 32.08, 18.23, ppm; HRMS (ESI) calcd for C32H26F2O4N4Na+ (M + Na)+ 591.1904, found 591.1805.
((3,6-Bis(4-fluorophenyl)-2-(thiophene-2-carbonyl)-2,3,4,5-tetrahydropyridazin-3-yl)diazenyl)(thiophen-2-yl)methanone (2n). Yellow solid (119.6 mg, 92% yield); mp = 170–172 °C; 1H NMR (300 MHz, CDCl3) δ 8.03 (dd, J = 3.9, 1.3 Hz, 1H), 7.93–7.85 (m, 2H), 7.82 (dd, J = 4.9, 1.0 Hz, 1H), 7.78 (dd, J = 3.8, 1.0 Hz, 1H), 7.74–7.63 (m, 3H), 7.21–7.05 (m, 6H), 2.79–2.68 (m, 2H), 2.43–2.40 (m, 2H), ppm; 13C NMR (75 MHz, CDCl3) δ 176.76, 167.21, 165.34, 163.60, 148.33, 138.29, 137.10, 136.52, 134.88, 133.72, 133.14, 132.57, 130.79, 130.67, 129.14, 128.79, 128.68, 127.76, 126.46, 126.34, 115.99, 115.83, 115.72, 115.70, 115.54, 115.45, 86.31, 32.51, 19.14, ppm; HRMS (ESI) calcd for C26H18F2N4O2S2Na+ (M + Na)+ 543.0705, found 543.0715.
N′-(1-Phenylallylidene)benzohydrazide (4). White solid (115.1 mg, 92% yield); mp = 125–127 °C; 1H NMR (300 MHz, DMSO) δ 11.12 (s, 1H), 7.88 (d, J = 6.1 Hz, 2H), 7.52 (dd, J = 18.7, 11.4 Hz, 8H), 7.15 (dd, J = 17.5, 11.3 Hz, 1H), 5.87 (d, J = 12.2 Hz, 1H), 5.53 (d, J = 17.5 Hz, 1H), ppm; 13C NMR (75 MHz, CDCl3) δ 163.18, 156.33, 136.99, 131.95, 130.03, 129.76, 128.56, 128.43, 128.33, 128.11, 126.99, 123.79, ppm; HRMS (ESI) calcd for C16H15ON2+ (M + H)+ 251.1179, found 251.1157.
((14-Benzoyl-5,6b,7,8,14,14a-hexahydrobenzo[h]naphtha[1,2-c]cinnolin-6a(6H)-yl)diazenyl)(phenyl)methanone (6). Yellow solid (114.0 mg, 87% yield); mp = 198–200 °C; 1H NMR (300 MHz, DMSO) δ 7.70 (t, J = 7.5 Hz, 1H), 7.63 (d, J = 7.5 Hz, 2H), 7.54 (t, J = 7.7 Hz, 4H), 7.50–7.42 (m, 2H), 7.35 (dd, J = 14.7, 8.1 Hz, 4H), 7.27 (d, J = 8.5 Hz, 1H), 7.22 (d, J = 6.9 Hz, 1H), 7.17 (d, J = 7.3 Hz, 2H), 7.11 (t, J = 7.5 Hz, 1H), 5.35 (dd, J = 11.4, 4.7 Hz, 1H), 3.53 (dd, J = 11.2, 5.7 Hz, 1H), 3.14 (t, J = 16.2 Hz, 2H), 2.88 (s, 2H), 2.38 (s, 2H), 2.24 (s, 2H), ppm; 13C NMR (75 MHz, DMSO) δ 182.09, 169.60, 144.63, 139.83, 136.20, 135.88, 135.58, 135.04, 131.79, 130.70, 130.30, 130.18, 129.79, 129.70, 129.60, 129.29, 128.85, 128.55, 127.81, 126.73, 125.70, 124.15, 72.99, 52.19, 28.92, 27.51, 25.00, 22.57, ppm; HRMS (ESI) calcd for C34H29O2N4+ (M + H)+ 525.2272, found 525.2272.
(3,6-Diphenyl-5,6-dihydropyridazin-1(4H)-yl)(phenyl)methanone (7). Yellow solid (146.3 mg, 86% yield); mp = 147–149 °C; 1H NMR (300 MHz, CDCl3) δ 7.90–7.77 (m, 2H), 7.59 (dd, J = 6.7, 2.9 Hz, 2H), 7.47–7.45 (m, 3H), 7.35–7.24 (m, 6H), 7.18 (d, J = 7.1 Hz, 2H), 6.09 (s, 1H), 2.69 (dd, J = 16.9, 2.4 Hz, 1H), 2.45–2.14 (m, 3H), ppm; 13C NMR (75 MHz, CDCl3) δ 170.21, 146.89, 139.93, 137.17, 135.34, 130.20, 130.01, 129.17, 128.80, 128.40, 127.38, 127.32, 125.46, 125.38, 51.69, 23.98, 18.72, ppm; HRMS (ESI) calcd for C23H21ON2+ (M + H)+ 341.1648, found 341.1623.
N′-(1,4-Diphenylbutyl)benzohydrazide (8). White solid (140.3 mg, 82% yield); mp = 99–101 °C; 1H NMR (300 MHz, DMSO) δ 9.88 (d, J = 4.7 Hz, 1H), 7.73 (d, J = 7.8 Hz, 2H), 7.54–7.46 (m, 1H), 7.41 (t, J = 7.6 Hz, 2H), 7.31–7.34 (m, 4H), 7.28–7.18 (m, 3H), 7.14 (t, J = 7.2 Hz, 3H), 5.27 (s, 1H), 4.06 (s, 1H), 1.86 (dd, J = 13.3, 8.9 Hz, 1H), 1.67–1.38 (m, 3H), ppm; 13C NMR (75 MHz, DMSO) δ 166.72, 143.35, 142.90, 134.11, 132.05, 129.10, 129.07, 129.05, 128.96, 128.49, 127.99, 127.92, 126.47, 64.67, 35.90, 35.32, 28.42, ppm; HRMS (ESI) calcd for C23H25ON2+ (M + H)+ 345.1961, found 345.1964.
Conclusions
In summary, we have developed an efficient protocol for the preparation of various functionalized tetrahydropyridazines via catalyst-free self [4 + 2] cycloaddition of in situ generated 1,2-diaza-1,3-dienes. The methodology features accessible starting reagents, convenient operating conditions, a broad substrate scope, and high efficiency. Further studies to explore the application of 1,2-diaza-1,3-dienes to other reaction systems and the evaluation of biological activity of the products are ongoing in our laboratory.
Acknowledgements
This work was financially supported by the National Natural Science Foundation of China (Grant No. 21102179, 21572271 and 81473078), Qing Lan Project, the project-sponsored by SRF for ROCS, SEM and National Found for Fostering Talents of Basic Science (Grant No. J1030830).
Notes and references
-
(a) S. H. Kim and R. D. Rieke, Molecules, 2010, 15, 8006 CrossRef CAS PubMed;
(b) K. Salat, A. Moniczewski and T. Librowski, Mini-Rev. Med. Chem., 2013, 13, 335 CAS;
(c) M. S. Sham, S. Nidhi, J. Monika, B. S. N. Reddy and J. W. Lown, Curr. Med. Chem., 2002, 9, 1045 CrossRef;
(d) M. Yan and S. Ma, ChemMedChem, 2012, 7, 2063 CrossRef CAS PubMed;
(e) R. F. George, M. A. Fouad and I. E. O. Gomaa, Eur. J. Med. Chem., 2016, 112, 48 CrossRef CAS PubMed;
(f) R. F. George and D. O. Saleh, Eur. J. Med. Chem., 2016, 108, 663 CrossRef CAS PubMed;
(g) K. Kusakabe, N. Ide, Y. Daigo, T. Itoh, T. Yamamoto, H. Hashizume, K. Nozu, H. Yoshida, G. Tadano, S. Tagashira, K. Higashino, Y. Okano, Y. Sato, M. Inoue, M. Iguchi, T. Kanazawa, Y. Ishioka, K. Dohi, Y. Kido, S. Sakamoto, S. Ando, M. Maeda, M. Higaki, Y. Baba and Y. Nakamura, J. Med. Chem., 2015, 58, 1760 CrossRef CAS PubMed;
(h) G. H. Laszlo, T. Julia, S. Geza, U. Szabolcs, M. N. Katalin, M. Bernadett, Z. Gabriella, C. Andrea, T. Pal, K. Akos and M. Peter, Curr. Pharm. Des., 2015, 21, 2291 CrossRef.
-
(a) M. A. Ciufolini and N. Xi, Chem. Soc. Rev., 1998, 27, 437 RSC;
(b) A. J. Oelke, D. J. France, T. Hofmann, G. Wuitschik and S. V. Ley, Nat. Prod. Rep., 2011, 28, 1445 RSC.
- N. Shimada, K. Morimoto, H. Naganawa, T. Takita, M. Hamada, K. Maeda, T. Takeuchi and H. Umezawa, J. Antibiot., 1981, 34, 1613 CrossRef CAS PubMed.
- R. B. Lingham, J. A. Hsu, A. F. Brien, J. M. Sigmund, M. M. Gagliardi, B. K. Heimbuch, O. Genilloud, I. Martin, M. T. Martin, C. F. Hirsch, D. L. Zink, J. M. Liesch, G. E. Koch, S. E. Gartner, G. M. Garrity, N. N. Tsou, G. M. Salituro and G. M. Salituro, J. Antibiot., 1996, 49, 253 CrossRef CAS PubMed.
-
(a) D. W. Combs, K. Reese, L. A. M. Cornelius, J. W. Gunnet, E. V. Cryan, K. S. Granger, J. J. Jordan and K. T. Demarest, J. Med. Chem., 1995, 38, 4880 CrossRef CAS PubMed;
(b) D. W. Combs, K. Reese and A. Phillips, J. Med. Chem., 1995, 38, 4878 CrossRef CAS PubMed.
- P. J. Rybczynski, D. W. Combs, K. Jacobs, R. P. Shank and B. Dubinsky, J. Med. Chem., 1999, 42, 2403 CrossRef CAS PubMed.
-
(a) O. A. Attanasi, L. D. Crescentini, G. Favi, P. Filippone, F. Mantellini, F. R. Perrulli and S. Santeusanio, Eur. J. Org. Chem., 2009, 3109 CrossRef CAS;
(b) J.-R. Chen, W.-R. Dong, M. Candy, F.-F. Pan, M. Jörres and C. Bolm, J. Am. Chem. Soc., 2012, 134, 6924 CrossRef CAS PubMed;
(c) S. Gao, J.-R. Chen, X.-Q. Hu, H.-G. Cheng, L.-Q. Lu and W.-J. Xiao, Adv. Synth. Catal., 2013, 355, 3539 CrossRef CAS;
(d) M.-C. Tong, X. Chen, J. Li, R. Huang, H. Tao and C.-J. Wang, Angew. Chem., Int. Ed., 2014, 53, 4680 CrossRef CAS PubMed;
(e) O. A. Attanasi, G. Favi, P. Filippone, F. R. Perrulli and S. Santeusanio, Org. Lett., 2009, 11, 309 CrossRef CAS PubMed;
(f) O. A. Attanasi, G. Favi, P. Filippone, F. Mantellini, G. Moscatelli and F. R. Perrulli, Org. Lett., 2010, 12, 468 CrossRef CAS PubMed;
(g) O. A. Attanasi, G. Favi, F. Mantellini, G. Moscatelli and S. Santeusanio, Adv. Synth. Catal., 2011, 353, 1519 CrossRef CAS;
(h) S. M. M. Lopes, A. F. Brigas, F. Palacios, A. Lemos and P. Melo, Eur. J. Org. Chem., 2012, 2152 CrossRef CAS;
(i) X.-Q. Hu, J.-R. Chen, S. Gao, B. Feng, L.-Q. Lu and W.-J. Xiao, Chem. Commun., 2013, 49, 7905 RSC;
(j) X.-Q. Hu, J.-R. Chen, Q. Wei, F.-L. Liu, Q.-H. Deng, A. M. Beauchemin and W.-J. Xiao, Angew. Chem., Int. Ed., 2014, 53, 12163 CrossRef CAS;
(k) O. A. Attanasi, L. D. Crescentinia, P. Filipponea, F. Fringuelli, F. Mantellinia, M. Matteuccib, O. Piermattib and F. Pizzo, Helv. Chim. Acta, 2001, 84, 513 CrossRef;
(l) E. Rossi, G. Abbiati, O. A. Attanasi, S. Rizzato and S. Santeusanio, Tetrahedron, 2007, 63, 11055 CrossRef CAS;
(m) O. A. Attanasi, G. Favi, F. Mantellini, S. Mantenuto, G. Moscatelli and S. Nicolini, Synlett, 2015, 26, 193 CAS;
(n) O. A. Attanasi, L. Bianchi, M. D. Auria, F. Mantellini and R. Racioppi, Curr. Org. Synth., 2013, 10, 631 CrossRef CAS;
(o) M. D. Auria, R. Racioppi, O. A. Attanasi and F. Mantellini, Synlett, 2010, 1363 Search PubMed.
- C. Guo, B. Sahoo, C. G. Daniliuc and F. Glorius, J. Am. Chem. Soc., 2014, 136, 17402 CrossRef CAS PubMed.
- Some sporadical reports on dimerization of the 1,2-diaza-1,3-butadiene, please see:
(a) S. M. Lopes, M. S. Henriques, J. A. Paixao and T. V. Pinhoemelo, Eur. J. Org. Chem., 2015, 6146 CrossRef CAS;
(b) O. Attanasi, L. Crescentini, G. Favi, F. Mantellini, S. Mantenuto and S. Nicolini, J. Org. Chem., 2014, 79, 8331 CrossRef CAS PubMed;
(c) R. Faragher and T. Gilchrist, J. Chem. Soc., Perkin Trans. 1, 1979, 249 RSC;
(d) T. Gilchrist, R. Wasson, F. King and G. Wootton, J. Chem. Soc., Perkin Trans. 1, 1987, 2511 RSC;
(e) K. Banert and M. Hagedorn, Tetrahedron Lett., 1992, 33, 7331 CrossRef CAS;
(f) X. Zhong, J. Lv and S. Luo, Org. Lett., 2015, 17, 1561 CrossRef CAS PubMed.
- J.-R. Chen, W.-R. Dong, M. Candy, F.-F. Pan, M. Jörres and C. Bolm, J. Am. Chem. Soc., 2012, 134, 6924 CrossRef CAS PubMed.
Footnote |
† Electronic supplementary information (ESI) available. See DOI: 10.1039/c6ra13985j |
|
This journal is © The Royal Society of Chemistry 2016 |
Click here to see how this site uses Cookies. View our privacy policy here.