DOI:
10.1039/C6RA12379A
(Paper)
RSC Adv., 2016,
6, 77944-77952
The role of a xylose isomerase pathway in the conversion of xylose to lipid in Mucor circinelloides
Received
12th May 2016
, Accepted 5th August 2016
First published on 5th August 2016
Abstract
The xylose isomerase (XI) pathway, which converts xylose in lignocellulosic materials into intermediate metabolites, does not commonly exist in filamentous fungi. The genomic sequence of Mucor circinelloides shows that it has the XI pathway but it has never been characterized. In this study, the genes coding for XI and xylulokinase (XK) were overexpressed in Mucor circinelloides to examine their effects on xylose utilization and the xylose metabolism pathway. The results showed that overexpressing XI or XK increased the total consumption rate of xylose and the lipid yield. In the lipid accumulation phase, the mRNA levels of XI and XK, and activities of XI, XK, glucose 6-phosphate dehydrogenase (G6PDH) and 6-phosphogluconate dehydrogenase (6PGDH) in recombinant strains were higher than the control. Our study suggested that the XI pathway plays an important role in xylose utilization and lipid accumulation in the filamentous fungus Mucor circinelloides.
1. Introduction
Microbial lipids, also named single cell oils (SCO), have become an alternative to traditional feedstocks to produce third-generation biodiesel due to their high productivity and low land requirement.1,2 However, the high production costs of fermentation obstruct commercial production of SCO. It has been reported that about 60–75% of the total costs of microbial lipid production comes from the feedstock or carbon source.3 Therefore, a sustainable and economically-viable source could generate notable economic benefits for lipid production. As the most abundant renewable bioresource and low-cost biomass on earth,4 lignocellulosic biomass consists of cellulose (13.2–66.59%), hemicelluloses (14.8–50%), and lignin (10–40%).5,6 The biochemical route of lipid production from lignocellulosic biomass typically involves biomass pretreatment, enzymatic hydrolysis of the pretreated biomass to release monomeric sugars (a mixture of pentoses and hexoses), and fermentation of the sugars to lipids.7 Among these released monomeric sugars, glucose and xylose with the ratio of 1
:
1 to 3
:
1 (w/w) are the most abundant sugars. Efficient utilization of xylose can significantly increase the utilization ratio of lignocelluloses and generate higher economic benefits, as xylose is the second most abundant sugar in lignocellulose hydrolysate after glucose. For example, when both glucose and xylose are utilized by Saccharomyces cerevisiae, the utilization ratio of lignocellulose could increase from 30% to 50% compared with the situation where only glucose is used.8,9 Hence, finding the xylose-fermenting strain or engineering xylose metabolism in microbial cell factories is important for lipid biosynthesis from lignocellulosic biomass.10
The utilization of xylose in most microorganisms is involved in two pathways: the xylose reductase (XR: EC 1.1.1.21)/xylitol dehydrogenase (XDH: EC 1.1.1.9) pathway, and the xylose isomerase (XI: EC 1.1.1.21) pathway. The xylose reductase/xylitol dehydrogenase pathway is commonly found in yeasts and fungi, in which D-xylose is reduced to xylitol by XR and then xylitol is transformed to D-xylulose by XDH.11 In the xylose isomerase pathway, D-xylose is transformed catalytically to D-xylulose by xylose isomerase via isomerization. This pathway generally exists in prokaryotes, such as E. coli and Clostridium acetobutylicum.12 The xylose reductase/xylitol dehydrogenase pathway has an imbalanced co-factor preference of NADPH-preferred XR and NAD+-preferred XDH, and accumulates the intermediate by-product xylitol. While the xylose isomerase pathway can avoid these problems, it is more desirable to engineer the xylose isomerase pathway to enhance the utilization of xylose.13–15 However, although XI commonly occurs in bacteria, the species boundary makes xylose isomerase fail to be functionally expressed in yeasts and fungi.13,14 The first reported XI in a fungus was the anaerobic fungus Orpinomyces,16 which could be expressed in yeast and showed appreciable enzyme activity.15
Currently, many more studies focus on lipid production by filamentous fungi. They can produce high levels of lipids that are typically mixtures of TAGs with significant quantities of very high-value polyunsaturated FAs such as γ-linolenic acid (GLA, n-6), arachidonic acid (ARA, n-6), and docosahexaenoic acid (DHA, n-3). Mucor circinelloides is a well-known oleaginous fungi belonging to the zygomycota phylum. It produces high levels of GLA which is a valuable omega-6 polyunsaturated fatty acid.17 A lot of studies have demonstrated that M. circinelloides strains can grow on multiple carbon sources including glucose, xylose, mannose and cellobiose.18–20 However, little research has focused on the mechanism of xylose metabolism in M. circinelloides.
Based on the sequenced genome of M. circinelloides CBS 277.49, we identified the gene xylA encoding XI, xyl2 encoding XDH and xks1 encoding xylulokinase (XK: EC 2.7.1.17). Therefore, we suggest that the xylose utilizing pathway in M. circinelloides CBS 277.49 is as follows: D-xylose is firstly converted to D-xylulose by XI, which is then phosphorylated into xylulose-5-phosphate (X5P) by XK. Xylulose-5-phosphate will enter the pentose phosphate pathway (PPP). In addition, a part of the xylulose may be converted to xylitol by XDH (Fig. 1B).
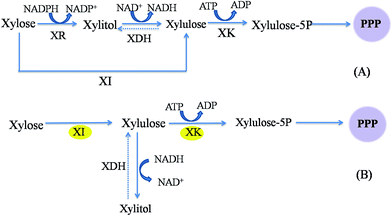 |
| Fig. 1 (A) Xylose utilizing pathway in microorganisms and (B) the xylose isomerase pathway in M. circinelloides CBS 277.49 where XR, XI, XDH and XK represent xylose reductase, xylose isomerase, xylitol dehydrogenase and xylulose kinase, respectively. | |
In the present study, we firstly investigated the utilization of xylose in strains M. circinelloides CBS 277.49, CBS 108.16 and WJ11. Then, we compared differences in biomass, lipid content, and fatty acid composition of M. circinelloides when glucose or xylose was taken as the only carbon source. Then the effect of the XI pathway on xylose assimilation and lipid production was investigated by overexpression of XI and XK genes, respectively, in M. circinelloides CBS 277.49. To our knowledge, this is the first report of the role of the XI pathway in M. circinelloides.
2. Materials and methods
2.1 Microorganisms and cultivation
All the strains and plasmids used in this work are listed in Table 1, and the primers are listed in Table 2. M. circinelloides CBS 277.49, WJ11 and 108.16 were used to assess the capacity of xylose metabolism. Pleu-MU402 is an auxotrophic strain for uridine, used for vector transformation. Escherichia coli strain Top10 was used in all cloning experiments and plasmid amplifications.
Table 1 Plasmids and strains used in the present study
Plasmids/strains |
Relevant description |
Reference or source |
Plasmids |
pMAT1552 |
|
Garre lab21 |
pMAT1552-xylA |
pMAT1552 containing xylA |
This study |
pMAT1552-xks1 |
pMAT1552 containing xks1 |
This study |
![[thin space (1/6-em)]](https://www.rsc.org/images/entities/char_2009.gif) |
Strains |
E. coil Top10 |
|
|
M. circinelloides CBS 277.49 |
|
|
M. circinelloides 108.16 |
|
|
M. circinelloides WJ11 |
|
Our lab26 |
Pleu-MU402 |
|
Our lab32 |
Mc-1552 |
Pleu-MU402 integrated with the plasmid pMAT155 |
Our lab32 |
Mc-XI |
Pleu-MU402 integrated with the recombinant plasmid pMAT1552-xylA |
This study |
Mc-XK |
Pleu-MU402 integrated with the recombinant plasmid pMAT1552-xks1 |
This study |
Table 2 Primers used in this study
Primers |
Sequence (5′ to 3′) |
XI-F |
CTTTTATATACAAAATAACTAAATCTCGAGATGCATAATGTATACAGCTATGAAATCG |
XI-R |
GTACTACTAGTCGCAATTGCCGCGGCTCGAGTTACTTCTTCTTTCTGGCAGCC |
XK-F |
CTTTTATATACAAAATAACTAAATCTCGAGATGGAACACTATTATCTCGGTC |
XK-R |
GTACTACTAGTCGCAATTGCCGCGGCTCGAGTTATTGAACAATGCTCATTTCT |
XI-qF |
AAATGGAAAGAAGAGCCCACAGT |
XI-qR |
CCAAACATCATCGTCTACCGTCT |
XK-qF |
ATTAGGCACATCGGATACAGTTC |
XK-qR |
ATTGCTATCAGCGTATTCGTCTC |
1552-F |
CTTTTATATACAAAATAACTAAATCTCGAG |
1552-R |
GTACTACTAGTCGCAATTGCCGCGGCTCGAG |
M. circinelloides was preserved via spore suspension in 30% (v/v) glycerol at −80 °C, and the concentration of spores was approx. 107 spores per mL. Growth of the cultures was performed in modified K & R medium. For lipid accumulation, the culture medium contained 2 g L−1 of ammonium tartrate, 80 g L−1 of xylose or glucose; other ingredients were the same as the modified K & R medium. For lipid accumulation of the overexpressing strains, the initial concentration of the carbon source was 50 g L−1. 100 μL of spores was used to inoculate 100 mL growth medium in a 500 mL baffled flask at 30 °C with 150 rpm agitation for 24 h, and then used at 10% (v/v) to inoculate 200 mL of liquid medium in 1 L baffled flasks. All experiments were carried out in triplicate.
All reagents used in cultivations were purchased from Sinopharm Chemical Reagent Co., Ltd (Shanghai, China). All of them were standard commercial products of analytical grade unless otherwise indicated.
2.2 Construction of recombinant plasmids and transformation
The XI and XK genes were cloned from genomic cDNA of M. circinelloides 277.49 using XI-F and XT-R, XK-F and XK-R, respectively. The fragments contain 30 bp homologous sequences of both sides of the XhoI restriction sites in pMAT1552 (which was kindly provided by Prof V. Garre from the University of Murcia, Spain). Then we ligated the fragments to pMAT1552 using XhoI under the control of the strong zrt1 promoter of M. circinelloides to create the recombinant plasmids pMAT1552-xylA and pMAT1552-xks1 (One step cloning kit, Takara). The plasmid contained the M. circinelloides pyrG gene (coding for uridine) as a selectable marker and was surrounded up- and down-stream by 1 kb of CarRP sequences corresponding to regions surrounding the carotenegenic CarRP gene to allow its chromosomal integration by homologous recombination. The recombinant plasmids were separately transformed into the uridine auxotrophic strain, pleu-MU402, as described previously21 to get the transformants and screened on a YNB agar plate, generating the recombinant Mc-XI (xylA-overexpression) and Mc-XK (xks1-overexpression), respectively. The plasmid pMAT1552 was also transformed into pleu-MU402 to get the recombinant Mc-1552 as the control strain.
2.3 Genomic DNA preparation, RNA extraction and RT-qPCR for transcriptional analysis
Genomic DNA was prepared using the DNA Quick Plant System kit (Tiangen Biotech Co., Ltd), and extracted from the mycelium. Total RNA of M. circinelloides was prepared using the TRIzol method. About 2 μg of total RNA was reversed to cDNA by the PrimeScript™ 1st strand cDNA Synthesis Kit (Takara).
The RTq-PCR was performed in the CFX connect Real-Time System (Bio-Rad) using the corresponding primers XI-qF/qR and XK-qF/qR and the conditions of the qPCR reactions were as follows: 95 °C for 3 min, 40 cycles of 95 °C for 15 s and 50 °C for 30 s. The relative transcript levels of the XI and XK genes were analyzed using the 2−ΔΔCT (Livak) method with 18S rRNA mRNA as the endogenous control. All experiments were carried out in triplicate.
2.4 Enzyme activity determination
The activities of the enzymes involved in the catabolism of xylose in M. circinelloides were determined as follows.
All the strains were harvested by filtration, disrupted by using a mortar in liquid N2 and suspended in buffer containing 100 mM KH2PO4/KOH, 20% (w/v) glycerol, 1 mM benzamidine HCl and 1 mM DTT, at pH 7.5, then centrifuged at 4 °C and 10
000 g for 10 min to prepare the crude extract. Protein concentrations were determined by the Bradford method with bovine serum albumin (BSA) as a standard.22
The XI activity was measured as described by Kuyper, et al.23 The activity of XK was determined as described by Komeda, et al.24 The activity of glucose 6-phosphate dehydrogenase (G6PD) and 6-phosphogluconate dehydrogenase (6P GD) was assayed as described by Wynn et al.25 Enzyme activity was measured by monitoring the rate of NADPH appearance or NADH disappearance at 340 nm using a spectrophotometer. One unit of enzyme activity was defined as the amount of enzyme required to reduce 1 μmol NADP per min or oxidize 1 μmol NADH per min. Benzamidine HCl and 1 mM DTT were purchased from Sinopharm Chemical Reagent Co., Ltd (Shanghai, China). Other reagents used in enzyme activity determination such as NADP and NADH were purchased from Sigma-Aldrich.
2.5 Other analyses
The biomass, lipid content of biomass and relative proportions of fatty acids in lipid were measured according to the procedures reported in a previous study.26 Residual glucose concentration in the medium was measured using a glucose oxidase kit.
The concentrations of xylose, ethanol and xylitol in the medium were determined by HPLC (waters 600), using a differential refractive index detector and a Sugarpak1 column (6.5 mmid × 300 mm). The HPLC system was operated at 85 °C, and the mobile phase was distilled water at a flow rate of 0.4 mL min−1.
2.6 Statistical analysis
The experimental data from three independent biological replicates were used to calculate the mean values and the standard deviation using SPSS 16.0 for Windows (SPSS Inc., Chicago, IL). Student’s t-test was used to evaluate differences between the data and P-values under 0.05 was considered significantly different.
3. Results and discussion
3.1 Effect of xylose on growth in M. circinelloides
To investigate lipid production by M. circinelloides with xylose as a carbon source, M. circinelloides CBS 277.49, WJ11 and 108.16 were respectively inoculated into K & R medium with the addition of xylose at an initial concentration of 80 g L−1, and glucose was used in the control experiments. After cultivation for 72 h, the residual sugar and xylitol in the cultures of M. circinelloides CBS 277.49, WJ11 and 108.16 were measured (Table 3). It showed that all strains could assimilate xylose as a carbon source for their growth although the xylose utilizing efficiency is lower than that of glucose. Among the three strains, M. circinelloides CBS 277.49 and WJ11 showed similar xylose consumption rates of 0.5 g h−1, which are faster than that in 108.16. Xylitol was not detected in the culture medium of WJ11 and 108.16, but there was 0.63 g L−1 of xylitol in the culture of CBS 277.49. This result indicated that xylose may be utilized in WJ11 and 108.16 through the xylose isomerase pathway, and supported our hypothesis about the xylose utilizing pathway in M. circinelloides 277.49 as described in the introduction. It had been reported that M. circinelloides could generate ethanol during fermentation,27 so we also analyzed the amount of ethanol in the fermentation supernatant by HPLC. The amount of ethanol produced in the xylose culture was about 0.09 to 2 g L−1, while the ethanol production in the glucose culture was about 2.50 to 8.21 g L−1 (Table 4). The yield of ethanol in the xylose culture was lower than that in the glucose culture. This result is consistent with the previous study.27
Table 3 The concentration of residual carbon source and xylitol in the culture supernatant of M. circinelloides CBS 277.49, WJ11 and 108.16 after cultivation for 72 h. All values are the mean of three biological replicates ± the standard deviations
|
Glucose (g L−1) |
Xylose (g L−1) |
Xylitol (g L−1) |
CBS 277.49 |
7.33 ± 1.60 |
43.89 ± 1.76 |
0.63 ± 0.18 |
WJ11 |
22.91 ± 4.19 |
43.83 ± 2.28 |
0 |
108.16 |
15.08 ± 2.21 |
49.44 ± 4.73 |
0 |
Table 4 The concentration of ethanol in the culture supernatant of M. circinelloides CBS 277.49, 108.16 and WJ11 after cultivation for 72 h. All values are the mean of three biological replicates ± the standard deviations
|
Xylose substrate |
Glucose substrate |
CBS 277.49 |
108.16 |
WJ11 |
CBS 277.49 |
108.16 |
WJ11 |
Ethanol (g L−1) |
1.52 ± 0.39 |
1.98 ± 0.23 |
0.09 ± 0.02 |
8.21 ± 1.04 |
4.90 ± 0.87 |
2.50 ± 0.63 |
The biomass, lipid productions and the fatty acid profiles are shown in Fig. 2. After 72 h of culture, three strains presented appreciable biomass on xylose with the dry cell weight (DCW) of 11 to 13 g L−1. The lipid content varied among different strains ranging from 10% to 32% of the DCW, and the yields of lipid ranged from 1 to 4.3 g L−1. As the fatty acid profiles showed, the major fatty acids in M. circinelloides were palmitic (C16:0), stearic (C18:0), oleic (C18:1), linoleic (C18:2) and γ-linolenic (C18:3γ, GLA) acid. Oleic acid was the dominant fatty acid with a percentage of 30–50% of the total fatty acids for all the strains grown on both glucose and xylose. Significant differences were observed in the fatty acid compositions for different strains, while the GLA content in the fungus when grown on xylose as the carbon source did not show significant difference compared with glucose as the carbon source. Although the consumed amount of xylose after 72 h of culture was 43% lower than the consumed amount of glucose, the biomass and lipid productions in M. circinelloides grown on xylose were almost same as those of that grown on glucose. This result suggested that the conversion rate of xylose to lipid is higher than that of glucose.
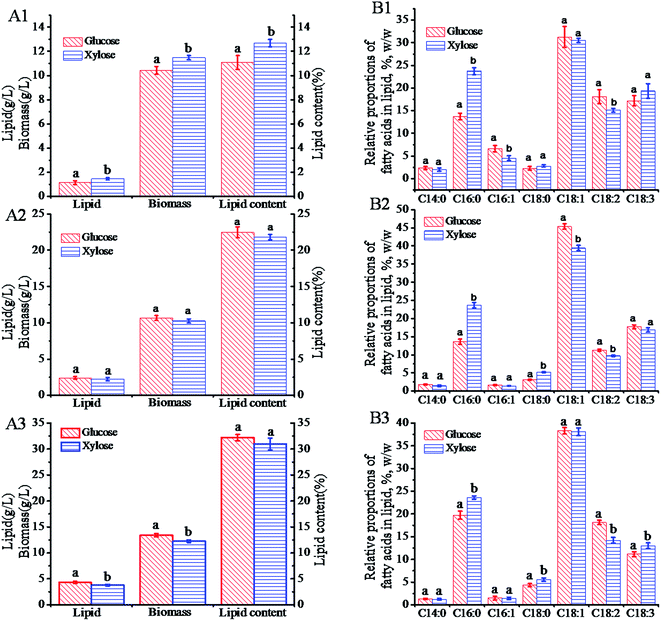 |
| Fig. 2 The biomass, lipid content and fatty acid profiles of M. circinelloides CBS 277.49 (A1 and B1), 108.16 (A2 and B2), and WJ11 (A3 and B3) grown in N-limited medium containing 80 g L−1 glucose or xylose. Different letters mean significant difference between the two carbon sources of xylose and glucose, P < 0.05. | |
As it is the second most abundant sugar in lignocellulose hydrolysate, efficient utilization of xylose is essential for commercial conversion of lignocelluloses to lipids. However, compared with glucose, many oleaginous fungi could not utilize xylose as a substrate or utilize xylose at a low rate though the XR/XDH pathway. Moreover, the biomass and lipid yield of fungi grown on xylose are still significantly lower than those grown on glucose, which limits the industrial application of oleaginous fungi. In the present work, M. circinelloides exhibited similar biomass and lipid yield grown on xylose compared with those grown on glucose. Close comparisons of the fermentation performance by M. circinelloides grown on different substrates revealed synergistic effects of the pathway integration (Fig. 3).
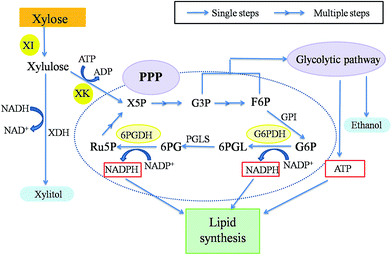 |
| Fig. 3 Xylose metabolic pathway in M. circinelloides. Note: X5P: xylulose 5-phosphate; G3P: glyceraldehyde-3-phosphate; F6P: fructose-6-phosphate; GPI: glucose 6-phosphate isomerase; G6P: glucose 6-phosphate; G6PDH: glucose 6-phosphate dehydrogenase; 6PGL: 6-phosphogluconolaetone; PGLS: phosphorgluconolactonase; 6 PG: 6-phosphogluconic acid; 6PGDH: 6-phosphogluconate dehydrogenase; Ru5P: ribose 5-phosphate. | |
For xylose metabolism in M. circinelloides, xylose was transformed to X5P and entered the non-oxidative PPP to complete the subsequent metabolism. Actually, previous studies suggested that the XI pathway did not need any redox cofactor, and the xylose metabolism could go through the non-oxidative PPP into central metabolism. So, when xylose is the sole carbon source, the carbon flux through the non-oxidative PPP would be higher than when glucose is the sole carbon source.12,28,29 The up-regulated carbon flux through the non-oxidative PPP might produce more glyceraldehyde-3-phosphate (G3P) and fructose-6-phosphate (F6P) entered the oxidative PPP and glycolytic pathway. The lower ethanol yield on xylose indicated that xylose metabolism might accumulate fewer by-products than glucose metabolism, which may be the cause of the higher conversion of xylose to lipid.
3.2 Generation of XI-overexpressing and XK-overexpressing strains of M. circinelloides by genetic engineering
In order to enhance the xylose utilizing efficiency in M. circinelloides, and study their effect on the growth and lipid accumulation of the fungus, we constructed the XI- and XK-overexpressing recombinant plasmids pMAT1552-xylA (Fig. 4A) and pMAT1552-xks1 (Fig. 4B), which were transformed into pleu-MU402 to get the recombinant strains Mc-XI and Mc-XK. The expression of the XI and XK genes in Mc-XI and Mc-XK was confirmed by PCR analysis using a primer pair (1552-F/R). The PCR product fragments for each recombinant were 1694 bp (1594 bp + 100 bp) and 859 bp (759 bp + 100 bp), which corresponded to the length of the XI and XK genes, respectively. The additional 100 bp fragment was amplified from the control strain Mc-1552 (Fig. 4C). PCR amplification results confirmed that the target genes had been integrated into the genome of the recombinant strains.
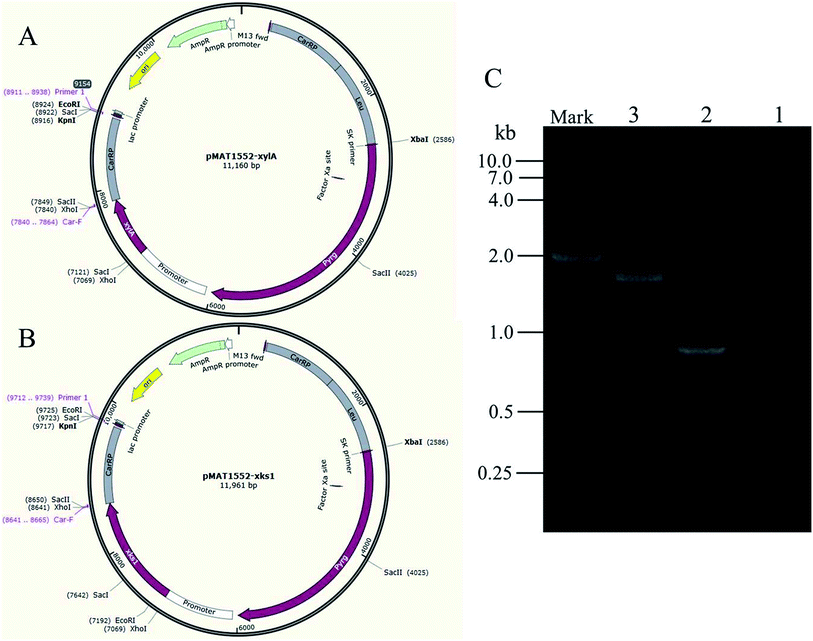 |
| Fig. 4 (A) Structure of the expression vector pMAT1552-xylA containing the xylA gene. (B) Structure of the expression vector pMAT1552-xks1 containing the xks1 gene. (C) PCR amplification from the genome of the overexpressing strains and the control strain Mc-1552 with the primers 1552-F/R. Lane 1, Mc-1552 (control); lane 2, Mc-XI; lane 3, Mc-XK; lane M, Gene Ruler DNA Ladder Mix. Sizes in kb of the relevant maker fragments are indicated. | |
3.3 Xylose utilization, growth and xylose metabolic pathway of the XI- and XK-overexpressing strains
The transformants Mc-XI, Mc-XK and Mc-1552 were grown in modified K & R medium with 50 g L−1 xylose, to evaluate the effect of XI and XK genes on xylose metabolism. Our preliminary data showed that the biomass of the three strains was almost the same during the fermentation, the biomass could reach about 11 g L−1 at 120 h. So we just compared the time course of xylose consumption and lipid content (Fig. 5). The concentrations of xylitol and ethanol in the medium are also shown in Table 5.
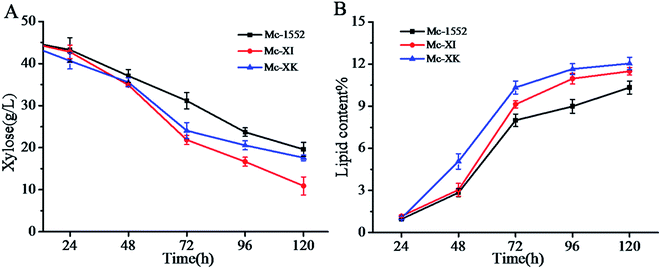 |
| Fig. 5 Xylose consumption (A) and lipid content (B) of the transformants Mc-1552, Mc-XI and Mc-XK grown in N-limited media. | |
Table 5 The concentration of xylitol and ethanol in the culture supernatant of the transformants Mc-1552, Mc-XI and Mc-XK during the cultivation. All values are the mean of three biological replicates ± the standard deviations
Time (h) |
Xylitol (g L−1) |
Ethanol (g L−1) |
Mc-1552 |
Mc-XI |
Mc-XK |
Mc-1552 |
Mc-XI |
Mc-XK |
24 |
0.18 ± 0.01 |
0.21 ± 0.02 |
0.19 ± 0.02 |
1.43 ± 0.16 |
1.90 ± 0.23 |
1.50 ± 0.13 |
48 |
0.13 ± 0.03 |
0.42 ± 0.03 |
0.06 ± 0.02 |
1.54 ± 0.07 |
3.46 ± 0.67 |
1.17 ± 0.25 |
72 |
0.08 ± 0.01 |
0.48 ± 0.08 |
0.07 ± 0.01 |
2.39 ± 0.56 |
4.54 ± 0.39 |
1.54 ± 0.39 |
96 |
0.14 ± 0.09 |
0.47 ± 0.06 |
0.08 ± 0.01 |
2.94 ± 0.52 |
6.91 ± 0.48 |
1.38 ± 0.30 |
120 |
0.07 ± 0.03 |
0.31 ± 0.07 |
0.04 ± 0.02 |
2.59 ± 0.51 |
8.23 ± 0.53 |
2.18 ± 0.21 |
In the XI-overexpressing strain, the residual xylose concentration of Mc-XI was 11.9 g L−1, while it was 19.6 g L−1 in Mc-1552 (the control). The consumption rate of xylose in Mc-XI was increased 1.4-fold compared with Mc-1552. It showed that overexpressing XI significantly improved the xylose catabolism capability. The increased consumption rate of xylose led to the lipid content increasing by 21% at 96 h. Besides, Mc-XI produced 0.31 g L−1 of xylitol and 8.23 g L−1 of ethanol within 120 h, which is higher than the control.
In theory, the increased consumption rate of xylose would result in a higher lipid yield while the lipid yield increased slightly in this study. However, the amount of ethanol increased 4-fold compared with the control Mc-1552. According to the xylose metabolism in M. circinelloides, a higher consumption rate of xylose would increase carbon flux through the non-oxidative PPP and the oxidative PPP was not significantly influenced. Meanwhile, the higher accumulation of xylitol in Mc-XI indicated that the XK activity might not be enough to phosphorylate all of the D-xylulose into xylulose-5-phosphate (X5P) with the consumption of ATP. Some of the D-xylulose was converted to xylitol by XDH with the consumption of NADH. The increased carbon flux through the non-oxidative PPP and the consumption of ATP and NADH might prompt most of the F6P and G3P produced in the non-oxidative PPP to enter into the glycolytic pathway to produce ATP, which may lead to increased accumulation of ethanol.
In the XK-overexpressing strain, the consumption rate of xylose in Mc-XK increased by 8% compared with that in Mc-1552. Overexpression of XK increased the lipid content at 96 h by 28%, and the amount of xylitol in the medium of Mc-XK was lower than in the control.
Overexpressing XI and XK could enhance the consumption rate of xylose, which is easy to understand due to their essential role in xylose conversion. The consumption rate of xylose in Mc-XK was lower than in Mc-XI, suggesting that XI might be more important to xylose consumption compared with XK. Previous reports demonstrated that overexpression of XK could result in accumulation of X5P and depletion of ATP, which would inhibit the cell growth.30,31 In this work, the biomass in Mc-XK did not show significant difference compared with others. The discrepancy could be explained by two reasons. First, the produced D-xylulose by xylose isomerase was not high enough to result in high levels of accumulation of X5P and depletion of ATP. Second, M. circinelloides possesses a strong capacity for substrate utilization, and the PPP in M. circinelloides could maintain metabolic flux at a steady state and therefore provide enough substrate for ATP synthesis in the glycolytic pathway.
3.4 Expression levels of the XI and XK genes in overexpressing strains
The mRNA levels of XI and XK in the overexpressing strains Mc-XI, Mc-XK and the control strain Mc-1552 were investigated by real time qPCR. The results showed that XK transcription was increased 7-fold in Mc-XK at 48 h (Fig. 6), while the transcription level of XI was almost similar to Mc-1552 during the fermentation. XI transcription was increased 10-fold at 48 h in Mc-XI. Interestingly, the mRNA level of XK in Mc-XI was also increased 3-fold at the same time. It suggested that the expression of the XI gene could induce the expression of the XK gene. This may be due to the accumulation of D-xylulose motivating the expression of the XK gene. Since XI and XK have already reached a high expression level, further work could try co-expression of XI and XK in M. circinelloides or combination with genetic modification of the PPP to improve the consumption of xylose and lipid production.
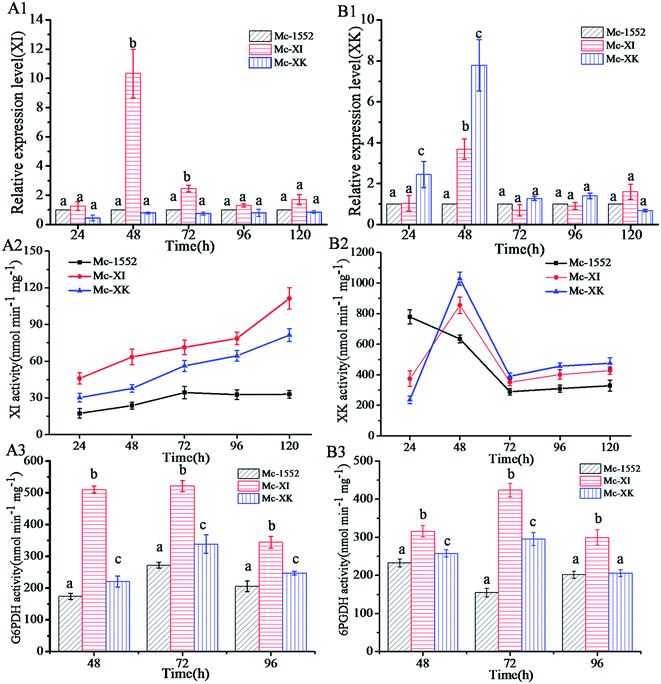 |
| Fig. 6 Expression levels of XI (A1) and XK (B1) genes in the overexpressing strains Mc-XI, Mc-XK and Mc-1552 (as control), analyzed by RT-qPCR. Specific enzyme activities of XI (A2) and XK (B2) in cell extracts of the tranformants. Activities of G6PDH (A3) and 6PGDH (B3) in cell extracts of the transformants at 48 h, 72 h, and 96 h of cultivation. Different letters mean significant difference among the three strains, P < 0.05. | |
3.5 Activities of XI and XK in the overexpressing strains
To estimate whether the enzymes encoded by the integrated genes in the overexpressing strains are active or not, the activities of XI and XK of the transformants grown on xylose were measured. As shown in Fig. 6, the activities of XI and XK in strains Mc-XI and Mc-XK were significantly higher than in Mc-1552, which is consistent with the increased xylose consumption rate in Mc-XI and Mc-XK. Although the transcription level of XI did not change, the activity of XI increased in Mc-XK.
The activity of XI increased throughout the fermentation. Conversely to the XI activity, the activity of XK in the control was high at 24 h, and then decreased with the progression of the fermentation. In the over-expressing strains, the highest activity of XK was achieved at 48 h, and then decreased with the cultivation time. Though the increased activity of XI could promote the consumption of xylose, the low XK activity may still affect the conversion of xylose. In general, D-xylulose must be converted to xylulose-5-phosphate (X5P) by XK. If the XK activity is low, it would accumulate xylitol and influence the xylose flux into the PPP. The XK activity of all the strains decreased after 48 h, which may affect the conversion of xylose to lipid eventually. The XK activity might be the limiting factor for xylose metabolism.
3.6 Activities of G6PDH and 6PGDH in the overexpressing strains
The PPP is not only an essential pathway for xylose metabolism, but it also plays an important role in the production of NADPH for lipid biosynthesis. Our previous studies had proved that over-expressing the enzymes glucose 6-phosphate dehydrogenase (G6PDH) and 6-phosphogluconate dehydrogenase (6PGDH) of the PPP can enhance the lipid yield.32 To investigate whether the xylose utilizing phenotype could affect lipid production of the fungi grown on xylose, the activities of G6PDH and 6PGDH in the overexpressing strains were also analyzed. The results showed that both the activities of G6PDH and 6PGDH in Mc-XI and Mc-XK were increased compared to the control strain and the activities of G6PDH and 6PGDH in Mc-XI were much higher than in Mc-XK (Fig. 6). These results were consistent with the previous hypothesis: xylose metabolism may increase carbon flux through the oxidative PPP. The increased yield of lipid can be attributed to the carbon flux of the oxidative PPP.
4. Conclusion
In this study, for the first time, we characterized the xylose isomerase pathway in M. circinelloides. The comparison study of lipid production by M. circinelloides grown on xylose and glucose showed that the xylose metabolism has a higher conversion rate of xylose to lipid compared with the glucose metabolism. Overexpressing XI and XK could increase the xylose specific consumption rate and the lipid production. Xylose assimilation is closely connected with the PPP, and regulation of the PPP could influence the conversion rate of xylose to lipid. M. circinelloides might be considered a potential organism to produce lipid from lignocellulosic biomass. The XI from this organism could be introduced to other organisms that lack the ability to ferment xylose by genetic modification in the future.
Acknowledgements
This research was supported by the National Natural Science Foundation of China (31271812 and 21276108), the National High Technology Research and Development Program of China (2012AA022105C), the Program for Changjiang Scholars and Innovative Research Team in University (IRT1249), the Program for New Century Excellent Talents (NCET-13-0831).
References
- G. S. Andrade, A. K. Carvalho, C. M. Romero, P. C. Oliveira and H. F. de Castro, Bioprocess Biosyst. Eng., 2014, 37, 2539–2548 CrossRef CAS PubMed.
- G. B. Leite, K. Paranjape, A. E. M. Abdelaziz and P. C. Hallenbeck, Bioresour. Technol., 2015, 184, 123–130 CrossRef CAS PubMed.
- R. Subramaniam, S. Dufreche, M. Zappi and R. Bajpai, J. Ind. Microbiol. Biotechnol., 2010, 37, 1271–1287 CrossRef CAS PubMed.
- C. Huang, X. F. Chen, L. Xiong, X. D. Chen, L. L. Ma and Y. Chen, Biotechnol. Adv., 2013, 31, 129–139 CrossRef CAS PubMed.
- V. Sanchez Nogue and K. Karhumaa, Biotechnol. Lett., 2015, 37, 761–772 CrossRef CAS PubMed.
- B. C. Saha, N. Qureshi, G. J. Kennedy and M. A. Cotta, Bioresour. Technol., 2015, 190, 182–188 CrossRef CAS PubMed.
- M. J. Jin, P. J. Slininger, B. S. Dien, S. Waghmode, B. R. Moser, A. Orjuela, L. D. Sousal and V. Balan, Trends Biotechnol., 2015, 33, 43–54 CrossRef CAS PubMed.
- P. Chandrakant and V. S. Bisaria, Appl. Microbiol. Biotechnol., 2000, 5, 301–309 CrossRef.
- J. Perez, J. Munoz-Dorado, T. de la Rubia and J. Martinez, Int. Microbiol., 2002, 5, 53–63 CrossRef CAS PubMed.
- E. M. Rubin, Nature, 2008, 454, 841–845 CrossRef CAS PubMed.
- T. W. Jeffries, I. V. Grigoriev, J. Grimwood, J. M. Laplaza, A. Aerts, A. Salamov, J. Schmutz, E. Lindquist, P. Dehal, H. Shapiro, Y. S. Jin, V. Passoth and P. M. Richardson, Nat. Biotechnol., 2007, 25, 319–326 CrossRef CAS PubMed.
- L. X. Liu, L. Zhang, W. Tang, Y. Gu, Q. Hua, S. Yang, W. H. Jiang and C. Yang, J. Bacteriol., 2012, 194, 5413–5422 CrossRef CAS PubMed.
- B. Peng, S. Huang, T. Liu and A. Geng, Microb. Cell Fact., 2015, 14, 70 CrossRef PubMed.
- J. Hou, Y. Shen, C. Jiao, R. Ge, X. Zhang and X. Bao, J. Biosci. Bioeng., 2016, 121, 160–165 CrossRef CAS PubMed.
- P. F. Li, H. B. Sun, Z. Chen, Y. Li and T. C. Zhu, Microb. Cell Fact., 2015, 14, 22 CrossRef PubMed.
- A. Madhavan, S. Tamalampudi, K. Ushida, D. Kanai, S. Katahira, A. Srivastava, H. Fukuda, V. S. Bisaria and A. Kondo, Appl. Microbiol. Biotechnol., 2009, 82, 1067–1078 CrossRef CAS PubMed.
- A. K. F. Carvalho, J. D. Rivaldi, J. C. Barbosa and H. F. de Castro, Bioresour. Technol., 2015, 181, 47–53 CrossRef CAS PubMed.
- C. E. R. Reis, J. G. Zhang and B. Hu, Appl. Biochem. Biotechnol., 2014, 174, 411–423 CrossRef CAS PubMed.
- Y. B. Zheng, X. C. Yu, J. J. Zeng and S. L. Chen, Biotechnol. Biofuels, 2012, 5, 50 CrossRef CAS PubMed.
- C. Xia, J. Zhang, W. Zhang and B. Hu, Biotechnol. Biofuels, 2011, 4, 15 CrossRef PubMed.
- S. Torres-Martinez, R. M. Ruiz-Vazquez, V. Garre, S. Lopez-Garcia, E. Navarro and A. Vila, Methods Mol. Biol., 2012, 898, 85–107 CAS.
- B. M. Marion, Anal. Biochem., 1976, 248–254 Search PubMed.
- M. Kuyper, H. Harhangi, A. Stave, A. Winkler, M. Jetten, W. Delaat, J. Denridder, H. Opdencamp, J. Vandijken and J. Pronk, FEMS Yeast Res., 2003, 4, 69–78 CrossRef CAS PubMed.
- H. Komeda, S. Yamasaki-Yashiki, K. Hoshino and Y. Asano, FEMS Microbiol. Lett., 2014, 360, 51–61 CrossRef CAS PubMed.
- J. P. Wynn, A. bin Abdul Hamid and C. Ratledge, Microbiology, 1999, 145, 1911–1917 CrossRef CAS PubMed.
- X. Tang, H. Chen, Y. Q. Chen, W. Chen, V. Garre, Y. Song and C. Ratledge, PLoS One, 2015, 10, e0128396 Search PubMed.
- T. L. L. b. s. J. N. M. McIntyre, Appl. Microbiol. Biotechnol., 2004, 63, 543–548 CrossRef PubMed.
- R. R. Bommareddy, W. Sabra, G. Maheshwari and A. P. Zeng, Microb. Cell Fact., 2015, 14, 36 CrossRef PubMed.
- T. M. Wasylenko and G. Stephanopoulos, Biotechnol. Bioeng., 2015, 112, 470–483 CrossRef CAS PubMed.
- Y. S. Jin, H. Ni, J. M. Laplaza and T. W. Jeffries, Appl. Environ. Microbiol., 2003, 69, 495–503 CrossRef CAS PubMed.
- X. Lu, X. Fu, H. Zong and B. Zhuge, J. Microbiol. Biotechnol., 2016, 26, 1252–1258 CrossRef PubMed.
- L. Zhao, X. Tang, X. Luan, H. Chen, Y. Q. Chen, W. Chen, Y. Song and C. Ratledge, RSC Adv., 2015, 5, 97658–97664 RSC.
|
This journal is © The Royal Society of Chemistry 2016 |