DOI:
10.1039/C6RA12238H
(Paper)
RSC Adv., 2016,
6, 96182-96189
Development and digestion of resistant malate starch produced by L-malic acid treatment
Received
12th May 2016
, Accepted 26th September 2016
First published on 26th September 2016
1. Introduction
Starch, which is the major dietary source of carbohydrate polymers, is the main component of cereal grains and tubers and is a type of carbohydrate consisting of many glucose units joined by glycosidic bonds.1,2 Furthermore, starch is the most important, natural, renewable, and digestible polysaccharide produced by many plants as a source of energy store.3 It is one of the most important biodegradable carbohydrate polymers in the human diet and the major constituent of many foods.4,5 However, obesity and diabetes rates have been increasing among adults in China over the past decade. The increase in the number of obesity and diabetes cases reported corresponds to the rise in the degree of cardiovascular risk.6,7 The investigators believed that the findings were related to a Chinese population that is becoming increasingly overweight and obesity and diabetes are becoming global health problems in adults as well as children.8,9 The rate of starch digestion depends on the amount of different dietary starches present.10 Resistant starch (RS) has digestive and absorptive properties and thus it would provide a reasonable diet. RS is not gelatinized during baking, thus, it can be considered as a dietetic fiber. RS is added as an alternative to increase fiber ingestion whereas the enzymes are used to overcome the gluten dilution. However, dietary RS is unique in that it is not rapidly digested like ordinary starch and provides important health benefits, some that are similar to those provided by dietary fiber (DF).11,12 Therefore, these are called “resistant starches (RS),” and extensive research has shown that RS had physiological functions similar to those of “DF”.13,14
Traditionally, malic acid is synthesized artificially from maleic acid and fumaric acid. However, pure L-malic acid (used, for example, for the production of medicine and food supplies) is biofermented from fumarate (synthesized from maleic anhydride) using enantioselective hydrolysis with fumarase, using either isolated enzyme or immobilized microorganisms. In industry, malic acid has been available for the preparation of food additives and as precursor of various fine chemicals.15
For digestive purposes, starches are classified into three types based on their rate of resisting enzymatic and non-enzymatic degradation: rapidly digestible starch (RDS), slowly digestible starch (SDS) and RS.16,17 However, the potential metabolic benefits of RS, which only retains its low degradability in the large intestine, includes the production of short-chain malate starch or L-malic acid, a decrease in colonic pH, augmentation of excrement production and output, and changes in intestinal microbiotic activity.18 Compared to mineral acid, L-malic acid is nutritionally healthy, and the yield of degradation of the malate resistant starch by amylase, lipase, and protease decreases with the increase in the degree of substitution (DS).19,20 The RS content of starches was reported to be increased with acid hydrolysis with heat-moisture treatment.21 Compared to inorganic acids, citric acid is harmless, and the rate of digestion of the esterified starch by pancreatin decreases with the increase in the DS.22,23 However, no information is available about the relationship between DS and resistant malate starches with different heat treatments.24 In this study, the main objective was to optimize the conditions for the development of malate resistant starch containing RDS, SDS and RS fragments using an L-malic acid treatment, and estimate the digestion characteristics of malate resistant starch.
2. Materials and methods
2.1. Materials
Corn starch was obtained from the Shandong Hengren Industrial Group Co., Ltd. (Shangdong, China). α-Amylase (item number: 10080) from hog pancreas was purchased from Sigma (powder, ∼50 U mg−1), and amyloglucosidase (AMG) [amyloglucosidase 300 L, 300 AGU per mL] were supplied by Novozymes (Bagsværd, Denmark). L-Malic acid was obtained from Zhengzhou Yuanfeng Food Technology Co., Ltd. (Henan, China). All other chemicals were of analytical reagent grade.
2.2. Preparation of malic acid treated corn starch
L-Malate starches were produced using a method based on that of Klaushofer with some modifications.22,25 Corn starch (25 g) was dispersed in volumes of 50% (w/v) L-malic acid solution, and then the pH of the solution was adjusted to 1.5 with 1 M sodium hydroxide (NaOH), and then mixed thoroughly. Corn starch was mixed with L-malic acid solution in a 200 mm Petri dish and dried for 16 h at room temperature. The Petri dish was then placed in a constant temperature oven and dried at 50 °C for 8–12 h to a moisture content of 5–10% (w/w). All the different esterification conditions were carried out in triplicate. The production was grinded and reacted in an air dry oven for 3–8 h with a high temperature of 120–170 °C. After treatment, the samples were cooled at room temperature for about 30 min. The dry reaction mixture was washed with 2–3 L water to remove unreacted L-malic acid, and filtered using a Büchner funnel. The washed starch was then placed in an air drying oven and dried at 45 °C and finally milled. Corn starch without L-malic acid was used as the controls following the same procedure.
2.3. Determination of DS
The amount of esterified L-malic acid bound to corn starch was determined using the procedure of Klaushofer.25 Generally, acid treated corn starch (5 g; m1) was dispersed in 50 mL of deionized water with three drops of phenolphthalein as indicator. After titrating the solution with 0.1 M NaOH until the reddish color had disappeared, the solution was dissolved in 25 mL of 0.5 M NaOH. The soluble matter was then boiled in a water bath kettle for 40 min with magnetic stirring and heat. After the saponification, the solution was cooled to room temperature, the saponification solution was titrated with 0.5 M hydrochloric acid (HCl) solution until the color of indicator (red-violet) disappeared. The volume of HCl (V1) used was recorded.
Corn starch (5 g; m2) was weighed and measured using the previous measurement method. The volume of HCl (V2) used was recorded until the indicator color (red-violet) disappeared. DS was calculated using the following equation:
|
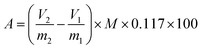 | (1) |
|
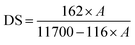 | (2) |
where
A (% by weight of substituent) is
L-malic acid substituent mass fraction, and
M is the concentration of standard solution (0.5 M). The values of 117 represents the molecular weight of the malic acid substituent group and 162 is the relative molecular weight of starch.
2.4. Resistant starch measurement
The digestibility of native corn starch (raw state) and different DS malate starches were determined according to the method of Englyst et al. with a slight modification in vitro.17 A sample (100 mg; M1 and M2) and 2 mL of water were added to a 50 mL screw-capped polypropylene centrifuge tubes and mixed using a magnetic stirrer for 5 min. Then, the mixtures were poured into 150 mL conical flasks with covers. Five glass beads and 20 mL of sodium acetate buffer (pH 5.8) were added to each flask. After equilibration at 37 °C for 20 min, 2 mL of porcine pancreatic α-amylase (7500 U mL−1) and AMG (300 U mL−1) were added, and then incubated in a water bath kettle at 37 °C with shaking (180 rpm). After 20 (G20) and 120 (G120) min of incubation, 20 mL of absolute alcohol was added to stop the enzymatic degradation. The glucose released in each enzymatic hydrolysate was determined using the dinitrosalicylic acid solution (DNS) method.26 The RDS, SDS, and RS percentage of each sample were calculated from the values of G120 (reducing sugar released after 120 min), and G20 (reducing sugar released after 20 min) as follows: |
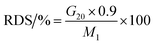 | (3) |
|
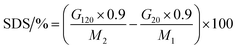 | (4) |
|
RS/% = 1 − RDS/% − SDS/%
| (5) |
2.5. Experimental design and data analysis
The effect of four independent variables on the response of the different DS malate starches used was evaluated using a three-level, four-factor factorial based on a Box–Behnken design (BBD) of response surface methodology (RSM).27 Four different factors were selected for investigation: reaction temperature (°C, X1), reaction time (min, X2), pH (X3), mass ratio (M
:
S, X4), and each variable was set at the three levels. The process variables were altered as shown in Table 1. A total number of 27 standard runs were used for the BBD matrix (Table 2). Each run was performed in duplicate and the DS produced was estimated as the response, Y.28 The BBD matrix with 27 standard runs in two blocks and three times of the midpoint are shown in Table 2. The predictive model of the BBD model could be written as the empirical second degree polynomial equation: |
 | (6) |
where Y is the predicted response (DS), β0 is the constant, and βi is the slope effect of the input factor Xi. However, βii is the linear interaction effect between the input factor Xi and Xj, and βij are the regression coefficients in the input factor Xi and Xj, respectively. Xi and Xj are the coded values of the factors.
Table 1 Box–Behnken response surface method design esterification parameters
Variable |
Minimum |
Mean |
Maximum |
Reaction temperature (°C) |
130 |
140 |
150 |
pH |
1 |
1.5 |
2 |
Reaction time (h) |
5 |
6 |
7 |
Mass ratio (M : S) |
0.4 |
0.5 |
0.6 |
Table 2 Response surface method results for malate starch production
Run |
Reaction temperature (°C) |
pH |
Reaction time (h) |
Mass ratio (M : S) |
Experimental values (DS) |
1 |
130 |
1.5 |
5 |
0.5 |
0.255 |
2 |
140 |
2 |
7 |
0.5 |
0.212 |
3 |
140 |
1.5 |
5 |
0.4 |
0.215 |
4 |
140 |
2 |
6 |
0.4 |
0.234 |
5 |
130 |
1 |
6 |
0.5 |
0.153 |
6 |
150 |
1.5 |
5 |
0.5 |
0.286 |
7 |
150 |
1.5 |
7 |
0.5 |
0.277 |
8 |
140 |
1 |
7 |
0.5 |
0.265 |
9 |
140 |
1.5 |
5 |
0.6 |
0.331 |
10 |
140 |
1.5 |
6 |
0.5 |
0.299 |
11 |
140 |
1.5 |
7 |
0.4 |
0.235 |
12 |
140 |
1.5 |
6 |
0.5 |
0.315 |
13 |
130 |
1.5 |
6 |
0.4 |
0.189 |
14 |
150 |
1.5 |
6 |
0.6 |
0.332 |
15 |
140 |
1 |
6 |
0.6 |
0.293 |
16 |
150 |
1 |
6 |
0.5 |
0.276 |
17 |
130 |
1.5 |
6 |
0.6 |
0.232 |
18 |
140 |
1.5 |
7 |
0.6 |
0.297 |
19 |
140 |
1 |
5 |
0.5 |
0.221 |
20 |
150 |
1.5 |
6 |
0.4 |
0.275 |
21 |
140 |
2 |
6 |
0.6 |
0.260 |
22 |
130 |
2 |
6 |
0.5 |
0.143 |
23 |
150 |
2 |
6 |
0.5 |
0.276 |
24 |
140 |
1 |
6 |
0.4 |
0.191 |
25 |
140 |
1.5 |
6 |
0.5 |
0.298 |
26 |
130 |
1.5 |
7 |
0.5 |
0.245 |
27 |
140 |
2 |
5 |
0.5 |
0.212 |
The software Design-Expert® 8.0.5 Trial (Stat-Ease Inc., Minneapolis, MN, USA) was used to determine the experimental design matrix, perform the data analysis and optimize the response of the dependent variables. The quality of the BBD model was expressed by the coefficient of determination (R2), whereas its statistical significance was checked using the sequential F-test. Linear and second order polynomials were fitted to the experimental runs to obtain the regression equations.
2.6. Spectral analysis of starch samples
The use of Fourier transform infrared (FT-IR) spectroscopy as a technique for routine analysis of DS of different starch samples was characterised. The range of FT-IR spectra was obtained by averaging 16 scans from 400 to 4000 cm−1 with the default resolution of 1 cm−1. Attenuated total reflectance (ATR) amendments were applied using the Spectrum One software (PerkinElmer) supplied with the device.
2.7. Statistical analysis
All the experimental runs were estimated in duplicate, and all the statistical results were evaluated using the statistical analysis software OriginLab 9.0 (OriginLab Corporation) and Matlab R2010a (The MathWorks, Inc.). Significant differences were established at p ≤ 0.05. The statistical results were reported as their average means ± standard deviation (SD), and the significant level was set at 0.05.
3. Results and discussion
3.1. Effect of reaction temperature on DS of malate starches
When L-malic acid was heated, it was sequentially dehydrated to anhydride. Corn starch was used as dehydrant in the heating process. The L-malic anhydride can then react with starch to form malate starch. A malate starch treated with L-malic acid at a concentration of 50% was reported to be optimum for the L-malic acid and starch reaction mixture.23 In this research, 50% L-malic acid with a pH of 1.5 was added to the corn starch for 6 h to produce malate starch, and the mass ratio (M
:
S) was 0.5. The effect of reaction temperature at 120–170 °C on the resistant starch content of malate corn starch was investigated. Fig. 1A shows that when the reaction time was 6 h and L-malic acid was 50% of the reaction mixture, the DS of malate starches increased from 0.171 to 0.292 as the heating temperature was increased from 120 to 140 °C, which confirmed that increasing the heating temperature may result in continuous dehydration of L-malic acid and produce maleic anhydride. The results showed that the higher reaction temperature resulted in a higher DS; however, the DS decreased from 0.292 to 0.286 when the heating temperature was 170 °C. However, malate starch obtained from corn starch would have a light brown color after 6 h at 150 °C, because the corn starch was obtained using pyrogenation.29 However, no color change was found at a heating temperature between 120 and 140 °C for 6 h.
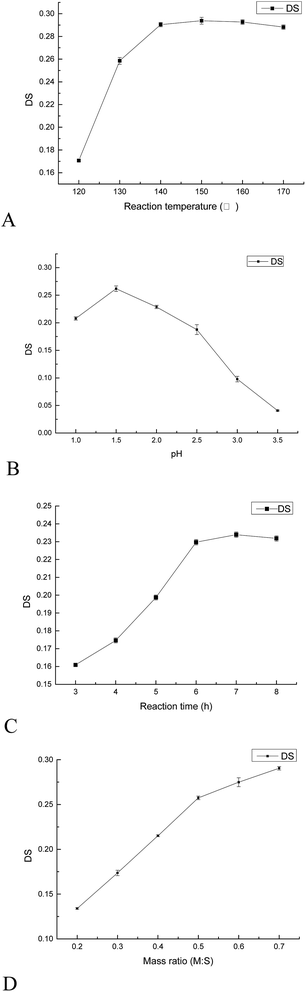 |
| Fig. 1 DS of corn starch treated with L-malic acid. The four univariate tests were reaction temperature (A), pH (B), reaction time (C), mass ratio (D). The values represent the mean of triplicate experiments and the error bars represent the SD. | |
3.2. Effect of pH on DS of malate starches
Following on from study of the effect of reaction temperature and time on DS of malate starches, to determine the effect of pH on DS of malate starches, the effect of pH from 1 to 3.5 on resistant starch content of malate corn starch was investigated. Fig. 1B shows that when the reaction time was 6 h and reaction temperature was 140 °C with 50% L-malic acid in the reaction mixture, the DS increased from 0.218 to 0.265 with a pH increase from 1 to 1.5. The results showed that the higher pH resulted in a higher DS; however, the DS decreased from 0.265 to 0.048 when the pH was 3.5, which indicated that increasing the pH may result in acidolysis of malate starches.
3.3. Effect of reaction time on DS of malate starches
Following on from study of the effect of reaction temperature on DS of malate starches, the reaction temperature was set at 140 °C to further study the effect of resistant starch content of malate starches, and 50% L-malic acid at pH 1.5 was added to the corn starch. The mass ratio (M
:
S) was 0.5. The effect of reaction time for 3–8 h on the resistant starch content of malate corn starch was investigated. Fig. 1C shows that the DS increased from 0.161 to 0.232 for corn starch when the heating reaction time increased from 3 h to 7 h at 140 °C, but was decreased to 0.229 when the heating reaction time was increased to 8 h. These results indicated that dissociation of the substituents from starch would happen when the reaction time was extended beyond 7 h. If corn starch was mixed with 50% L-malic acid before being heated to 140 °C for the dehydration reaction, this could allow more L-malic acid molecules to produce maleic anhydride, which then penetrated the starch granules to make more L-malic acid available for the esterification reaction. Also, the longer reaction time used in this study produced a more high performance thermochemical dehydration and esterification reaction. The highest DS malate starches were found when the heating reaction time was 7 h.
3.4. Effect of mass ratio on DS of malate starches
The reaction temperature was set at 140 °C for 6 h to determine the resistant starch content of malate starches, and 50% L-malic acid (pH 1.5) was added to the corn starch. To determine the effect of mass ratio on the DS of malate corn starches, a mass ratio from 0.2 to 0.7 was investigated. Fig. 1D shows that the DS of different malate starches increased from 0.132 to 0.281 with a mass ratio from 0.2 to 0.7, respectively. The results show that the higher mass ratio resulted in a higher DS; however, malate starch from corn starch was light brown in color when the mass ratio exceed 0.5.23 However, no color change was found using a mass ratio between 0.2 and 0.5.
3.5. Response surface optimization of malate starches
The results of BBD for optimizing the production of resistant corn starch using L-malic acid treatment are displayed in Table 3. A regression analysis of RSM variables indicated that the experimental results fit the quadratic model (P < 0.05). The determination coefficient (R2) of the BBD experimental design was 0.875, which showed that the current model does not contradict the observed data points. The data, after variance and multiple regression analysis, fit into a second-order polynomial model equation. The results yielded the following model curve: |
Y = 0.30 + 0.042X1 + 0.034X4 − 0.031X12 − 0.056X22
| (7) |
where Y was the predicted DS of malate starches production. Analysis of variance (ANOVA) results indicated that the model was reliable (P < 0.05) and the non-significant lack of fit value (P = 0.1082 > 0.05) demonstrated that the results from the RSM model agreed well with those obtained experimentally (Table 3). In this case A, D, A2, and B2 are significant model terms. It was apparent from the RSM model equation that the reaction temperature and pH were significant (P < 0.05) parameters for DS of resistant corn starch produced using malic acid treatment.
Table 3 ANOVA results for the selected quadratic model for DS in malate starch production
Term |
Coefficients |
P values |
Intercept |
0.3 |
0.0018 |
A-Temperature (°C) |
0.042 |
0.0001 |
B-pH |
−5.17 × 10−3 |
0.5024 |
C-Time (h) |
9.17 × 10−4 |
0.9044 |
D-M : S (M : S) |
0.034 |
0.0007 |
AB |
2.50 × 10−3 |
0.85 |
AC |
2.50 × 10−4 |
0.9849 |
AD |
3.50 × 10−3 |
0.7914 |
BC |
−0.011 |
0.412 |
BD |
−0.019 |
0.1678 |
CD |
−0.014 |
0.3174 |
A2 |
−0.031 |
0.0181 |
B2 |
−0.056 |
0.0003 |
C2 |
−0.017 |
0.1628 |
D2 |
−0.013 |
0.285 |
Lack of fit |
|
0.1082 |
R2 |
0.875 |
|
Fig. 2 shows that to obtain an optimal DS with RSM, the conditions required were: reaction temperature, 146 °C; pH, 1.46; reaction time, 5.52 h; and mass ratio, 0.60. After optimization assays, the DS of malate starch production at optimal conditions were determined to be 0.344, and experimentally a yield of 0.343 was obtained, confirming the validity of the RSM model.
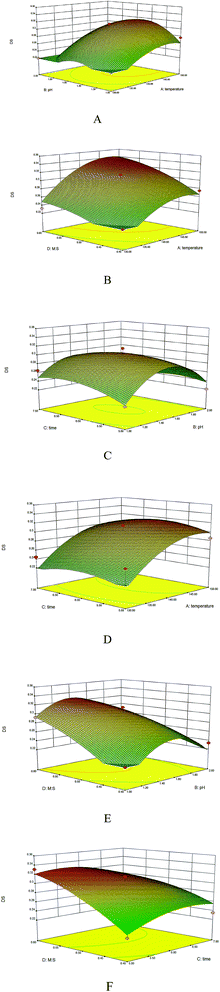 |
| Fig. 2 Response surface plots. (A) Surface plot showing the effect of reaction temperature and time on DS, (B) surface plot showing the effect of reaction temperature and pH on DS, (C) surface plot showing the effect of reaction temperature and mass ratio on DS, (D) surface plot showing the effect of reaction time and pH on DS, (E) surface plot showing the effect of mass ratio and pH on DS, (F) surface plot showing the effect of reaction time and mass ratio on DS. | |
3.6. The digestibility of resistant starch produced using malic acid treatment
The method used in the present study to classify resistant malate starches into RDS, SDS, and RS was analogous to that put forward by Englyst et al.17 However, DS of malate starch production and saponification reaction scales were slightly modified to allow the measurement of as many samples as possible. Therefore, our definition of the resistant starch fractions is slightly different from those of Englyst et al.17 The nutritional properties of RDS, SDS and RS contents of different DS of malate starches production after in vitro digestion are shown in Table 4. RS has been considered as the total amount of native starch and the products of malate starch digestion, which resisted degradation in the small intestine or could pass through to the large intestine after fermentation in vivo. RDS was rapidly and drastically hydrolyzed by amylopsin and glycosylase in the small intestine within 20 min. However, SDS, a desirable form of special dietary fiber, was slowly and progressively digested in the small intestine. It was presumed that the decrease in the SDS content of the malate starch production after L-malic acid treatment was because of the presence of RS type fractions formed by amylose–maleic anhydride complexing and linear–branch or branch–branch interactions. Also, the RS content of malate starch increased because of the increase in DS after L-malic acid treatment. After cooking at 100 °C, the L-malic treated corn starch contained substantial amounts of RS and SDS fractions (74.6%), although the RDS fraction still remained at about 25.4%. However, the slowly digestible and resistant starch fractions of the optimally acid-treated rice starch totalled 54.1%, which was 28.1% higher than that of the control.29
Table 4 The amount of rapid (RDS) and slow (SDS) digestible starches, and resistant starch (RS) of different DS malate starches after in vitro starch digestibility
DS |
RDS (%) |
SDS (%) |
RS (%) |
0.041 |
80.8 ± 3.2 |
15.7 ± 0.5 |
3.5 ± 0.02 |
0.097 |
38.9 ± 1.6 |
6.5 ± 0.2 |
54.6 ± 2.1 |
0.134 |
38.2 ± 1.2 |
3.9 ± 0.1 |
57.9 ± 2.2 |
0.171 |
37.9 ± 0.9 |
3.8 ± 0.2 |
58.3 ± 2.7 |
0.215 |
35.3 ± 1.2 |
3.6 ± 0.3 |
61.1 ± 3.1 |
0.258 |
28.4 ± 0.8 |
2.7 ± 0.1 |
68.9 ± 2.7 |
0.29 |
25.4 ± 1.4 |
1.45 ± 0.06 |
73.15 ± 3.3 |
3.7. Analysis with FT-IR spectroscopy
Analysis with FT-IR spectroscopy was used to elucidate the enhancement mechanism of the L-malic acid treated corn starch process. Fig. 3 shows the FT-IR spectra of the calibration and of various types of malate starch and is considered to be a valid means of determining the changes of the location of the chemical bonds in the carbohydrate polymers after the esterification process. The FT-IR spectra of different DS of malate starches production samples predicted that there were many differences of location changes in the region (4000–400 cm−1), which correspond to the ‘infrared fingerprints’ of starch. Data are shown in Fig. 3. Compared to the non-treated starch samples, the L-malic acid treated corn starch showed a reduction in the bands at 3300 to 2900 cm−1, which was an indication of O–H stretching because of the carbohydrate linked with the starch. The results showed that the intensity of these peaks was weaker compared with control corn starch, which indicated a decrease in surface starch after L-malic acid treatment. L-Malic acid treated corn starch showed a novel band at 1732 cm−1, which was an indication of the C
O stretching because corn starch was linked with maleic anhydride. Fig. 4 shows the reaction equation of malate starch production. However, a decrease in the intensity of peaks at the 1632 cm−1 (aromatic ring stretch) region was the transformation of the C–O bond.30
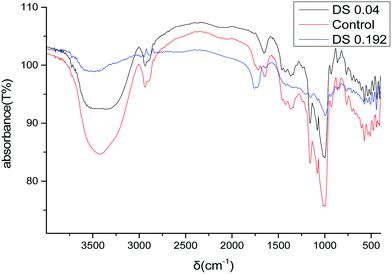 |
| Fig. 3 FT-IR spectra of the different DS of malate starches production. | |
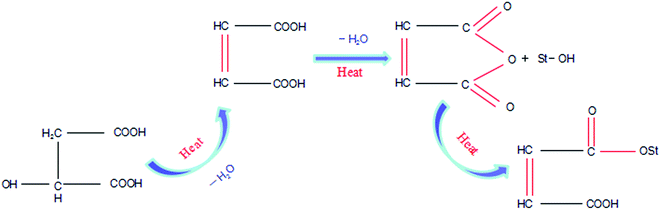 |
| Fig. 4 Reaction equation of malate starch production. | |
4. Conclusions
About the development and physicochemical characterization of resistant corn starch produced by malic acid treatment, the results showed that the optimal reaction conditions for the formation of malate resistant corn starch were: reaction temperature, 146 °C; pH, 1.46; reaction time, 5.52 h; and mass ratio (M
:
S), 0.60. Malate starches with different DS of resistant starches with different RS content were manufactured. Reaction temperature and mass ratio (M
:
S) had an important effect on the physicochemical properties of malate starch. The decrease of the SDS content on the malate starches produced after L-malic acid treatment was because of the presence of RS type fractions. Corn starch esterified by L-malic acid showed a novel band at 1732 cm−1, which was an indication of C
O stretching because the corn starch was linked with maleic anhydride.
Abbreviations
RSM | Response surface methodology |
RS | Resistant starch |
RDS | Rapidly digestible starch |
SDS | Slowly digestible starch |
DS | Degree of substitution |
DF | Dietary fiber |
TCA | Tricarboxylic acid |
DNS | Dinitrosalicylic acid solution |
FT-IR | Fourier transform infrared |
M : S | Malic : starch |
Acknowledgements
This research was financially supported by the high-level personnel Fund of HAUT (2016QNJH17) and Henan province modern corn agriculture industry technology system projects (S2010-02-G06).
Notes and references
- T. Witt, M. J. Gidley and R. G. Gilbert, Starch digestion mechanistic information from the time evolution of molecular size distribution, J. Agric. Food Chem., 2010, 58, 8444–8452 CrossRef CAS PubMed
. - D. J. Gallant, B. Bouchet and P. M. Baldwin, Microscopy of starch: evidence of a new level of granule organization, Carbohydr. Polym., 1997, 32, 177–191 CrossRef CAS
. - E. E. Blaak and W. H. M. Saris, Health aspects of various digestible carbohydrate, Nutr. Res., 1995, 10, 1547–1573 CrossRef
. - L. Iturriaga, B. Lopez and M. Anon, Thermal and physicochemical characterization of seven Argentine rice flours and starches, Food Res. Int., 2004, 37, 439–447 CrossRef CAS
. - A. K. Shrestha, J. Blazek, B. M. Flanagan, S. Dhital, O. Larroque and M. K. Morell, Molecular, mesoscopic and microscopic structure evolution during amylase digestion of maize starch granules, Carbohydr. Polym., 2012, 90, 23–33 CrossRef CAS PubMed
. - D. Pan, D. Zhang, J. Wu, C. Chen, Z. Xu and H. A. Yang, Novel proteogly can from Ganoderma lucidum fruiting bodies protects kidney function and amelio-rates diabetic nephropathy via its antioxidant activity in C57BL/6 db/db Mice, Food Chem. Toxicol., 2014, 63, 111–118 CrossRef CAS PubMed
. - B. S. Teng, C. D. Wang, D. Zhang, J. S. Wu, D. Pan and L. Pan, Hypo-glycemic effect and mechanism of a proteoglycan from Ganoderma lucidum onstreptozotocin-induced type 2 diabetic rats, Eur. Rev. Med. Pharmacol. Sci., 2012, 16, 166–175 CAS
. - G. Zhang, Z. Ao and B. R. Hamaker, Slow digestion property of native cereal starches, Biomacromolecules, 2006, 7, 3252–3258 CrossRef CAS PubMed
. - Y. Chen, M. Y. Xie, S. P. Nie, C. Li and Y. X. Wang, Purification, composition analysis and antioxidant activity of a polysaccharide from the fruiting bodies of Ganoderma atrum, Food Chem., 2008, 107, 231–241 CrossRef CAS
. - J. Y. Kim and K. C. Huber, Corn starch granules with enhanced load carrying capacity (LCC) via citric acid treatment, Carbohydr. Polym., 2013, 91(1), 39–47 CrossRef CAS PubMed
. - H. J. Chung, D. H. Shin and S. T. Lim, In vitro starch digestibility and estimated glycemic index of chemically modified corn starches, Food Res. Int., 2008, 41, 579–585 CrossRef CAS
. - M. Z. Nor Nadiha, A. Fazilah, R. Bhat and A. A. Karim, Comparative susceptibilities of sago, potato and corn starches to alkali treatment, Food Chem., 2010, 121, 1053–1059 CrossRef CAS
. - P. I. Akubor, Effect of ascorbic acid and citric acid treatments on the functional and sensory properties of yam flour, Int. J. Agr. Pol. Res., 2013, 1(4), 103–108 Search PubMed
. - N. Atichokudomchai and S. Varavinit, Characterization and utilization of acidmodified cross-linked tapioca starch in pharmaceutical tablets, Carbohydr. Polym., 2003, 53, 263–270 CrossRef CAS
. - E. Battat, Y. Peleg, A. Bercovitz, J. S. Rokem and I. Goldberg, Optimization of L-malic acid production by Aspergillus flavus in a stirred fermentor, Biotechnol. Bioeng., 1991, 37, 1108–1116 CrossRef CAS PubMed
. - A. Gunaratne and R. Hoover, Effect of heat-moisture treatment on the structure and physicochemical properties of tuber and root starches, Carbohydr. Polym., 2002, 49(4), 425–437 CrossRef CAS
. - H. N. Englyst, S. M. Kingman and J. H. Cummings, Classification and measurement of nutritionally important starch fractions, Eur. J. Clin. Nutr., 1992, 46, S33–S50 Search PubMed
. - P. Ambigaipalan, R. Hoover, E. Donner and Q. Liu, Starch chain interactions within the amorphous and crystalline domains of pulse starches during heat-moisture treatment at different temperatures and their impact on physicochemical properties, Food Chem., 2014, 143, 175–184 CrossRef CAS PubMed
. - S. Y. Moon, S. H. Hong, T. Y. Kim and S. Y. Lee, Metabolic engineering of Escherichia coli for the production of malic acid, Biochem. Eng. J., 2008, 40, 312–320 CrossRef CAS
. - R. J. Neufeid, Y. Peleg, J. S. Rokem, O. Pines and I. Goldberg, L-Malic acid formation by immobilized Saccharomyces cerevisiae amplified for fumarase, Enzyme Microb. Technol., 1991, 13, 991–996 CrossRef
. - S. I. Shin, J. Byun, K. H. Park and T. W. Moon, Effect of partial acid hydrolysis and heat-moisture treatment on formation of resistant tuber starch, Cereal Chem., 2004, 81, 194–198 CrossRef CAS
. - X. Xie and Q. Liu, Development and physicochemical characterization of new resistant citrate starch from different corn starches, Starch/Staerke, 2004, 56, 364–370 CrossRef CAS
. - S. I. Shin, C. J. Lee, M. J. Kim, S. J. Choi, H. J. Choi, Y. Kim and T. W. Moon, Structural characteristics of low-glycemic response rice starch produced by citric acid treatment, Carbohydr. Polym., 2009, 78, 588–595 CrossRef CAS
. - N. H. Kim, J. H. Kim, S. Lee, H. Lee, J. W. Yoon and R. Wang, Combined effect of autoclaving-cooling and cross-linking treatments of normal corn starch on the resistant starch formation and physicochemical properties, Starch/Staerke, 2010, 62(7), 358–363 CrossRef CAS
. - V. H. Klaushofer, E. Berghofer and R. Pieber, Quantitative Bestimmung von Citronensäure in Citratstärken, Starch/Staerke, 1979, 31, 259–261 CrossRef
. - A. L. Galant, R. C. Kaufman and J. D. Wilson, Glucose: detection and analysis, Food Chem., 2015, 154, 127–133 Search PubMed
. - S. Ferreira, A. P. Duarte, M. H. L. Ribeiro, J. A. Queiroz and F. C. Domingues, Response surface optimization of enzymatic hydrolysis of Cistus ladanifer and Cytisus striatus for bioethanol production, Biochem. Eng. J., 2009, 45, 192–200 CrossRef CAS
. - Q. G. Zhang, Z. Q. Zhang, X. F. Yue, X. H. Fan, T. Li and S. F. Chen, Response surface optimization of ultrasound-assisted oil extraction from autoclaved almond powder, Food Chem., 2009, 116, 513–518 CrossRef CAS
. - S. I. Shin, C. J. Lee, D. I. Kim, H. A. Lee, J.-J. Cheong, K. M. Chung, M.-Y. Baik, C. S. Park, C. H. Kim and T. W. Moon, Formation, characterization, and glucose response in mice to rice starch with low digestibility produced by citric acid treatment, J. Cereal Sci., 2007, 45, 24–33 CrossRef CAS
. - A. Flores-Morales, M. Jiménez-Estrada and R. Mora-Escobedo, Determination of the structural changes by FT-IR, Raman, and CP/MAS 13C NMR spectroscopy on retrograded starch of maize tortillas, Carbohydr. Polym., 2012, 87(1), 61–68 CrossRef CAS
.
|
This journal is © The Royal Society of Chemistry 2016 |
Click here to see how this site uses Cookies. View our privacy policy here.