DOI:
10.1039/C6RA10664A
(Paper)
RSC Adv., 2016,
6, 56035-56041
An unusual (4,4)-connected 3D porous cadmium metal–organic framework as a luminescent sensor for detection of nitrobenzene†
Received
25th April 2016
, Accepted 3rd June 2016
First published on 6th June 2016
Abstract
The metal–organic framework {[Cd2(obtz)2(btec)(H2O)2]·3.5H2O}n (1) was synthesized and characterized (obtz = 1,2-bis(1,2,4-triazole-1-ylmethyl)benzene, btec = 1,2,4,5-benzenetetracarboxylate). 1 shows an unusual (4,4)-connected 3D porous network with the Schläfli symbol of (62·8 × 103)2(63 × 103). 1 exhibits a strong blue emission band maxima at 421 nm and a shoulder peak at 464 nm in the solid state. The luminescent properties of 1 dispersed in various kinds of solvents were investigated in detail. The high quenching efficiency of 1 is up to 98.2% at 160 ppm NBZ. 1 displays highly selectivity, sensitivity and recyclability in the detection of nitrobenzene.
Introduction
Metal–organic frameworks (MOFs) have been widely applied in various fields including magnetism, adsorption, chiral materials, catalysts and luminescent sensors.1–4 Nitrobenzene is widely used as an important organic solvent and intermediate for organic synthesis, including the production of aniline. However, nitrobenzene is a highly toxic substance and can pollute the environment.5 A trace amount of it can cause serious harm to human beings, but the detection of a small quantity of it is difficult. The development of an effective sensor for detecting and monitoring nitrobenzene is a meaningful challenge for environmental and safety considerations.
The MOFs containing the d10 metal can exhibit the intense luminescent signal at the solid state and in the liquid solvents. The luminescent MOF-based sensors have been developed for the detection of cations, anions and small organic molecules.6–8 Recently, the luminescent MOFs for sensing nitrobenzene have also been reported.9 For example, a MOF [NH2(CH3)2][Cd17(L)12(μ3-H2O)4(DMF)2(H2O)2]·solvent (H3L = 2,4,6-tris[1-(3-carboxyl-phenoxy)ylmethyl]mesitylene) which was reported by Tian and coworkers exhibits quick response to the very small amount of nitrobenzene in solution.10 It is significant to design and synthesize the efficient sensitive sensors by using the luminescent ligand and the d10 metal Cd(II).
The bis(triazole) N-donor ligands have been widely used for construction of novel MOFs with intriguing properties.11 In previous work, we synthesized a series of coordination polymers with unusual structures and interesting properties using flexible bis(triazole) ligands such as 1,2-bis(1,2,4-triazol-1-yl)ethane (bte), 1,4-bis(1,2,4-triazol-1-yl)butane (btb), 1,4-bis(1,2,4-triazol-1-ylmethyl)benzene (bbtz), and 1,2-bis(1,2,4-triazol-4-yl)ethane (btre).12 In the present work, a bis(triazole) ligand 1,2-bis(1,2,4-triazole-1-ylmethyl)benzene (obtz) was selected as the main ligand to construct new MOF as potential luminescent sensor because obtz exhibits the luminescent property but the MOFs based on obtz are few reported.13 A new MOF {[Cd2(obtz)2(btec)(H2O)2]·3.5H2O}n (1) (btec = 1,2,4,5-benzenetetracarboxylate) was successfully synthesized. 1 shows an unusual (4,4)-connected 3D porous network. 1 exhibits high selectivity, sensitivity, and recyclability in the detection of nitrobenzene.
Experimental section
Materials and physical measurements
All reagents were analytical grade and used without further purification. Elemental analyses for C, H and N were performed on a Perkin-Elmer 240C analyser. IR spectra were obtained for KBr pellets on a Nicolet 170SX FT-IR spectrophotometer in the 4000–400 cm−1 region. X-ray powder diffractions were performed on a D/MAX-3C diffractometer with the Cu Kα radiation (λ = 1.5406) at room temperature. The luminescence measurements were carried out at room temperature and the spectra were collected with a PerkinElmer LS50B spectrofluorimeter. The UV-vis spectra were measured on a Varian Cary 500 UV-vis spectrophotometer.
Synthesis of {[Cd2(obtz)2(btec)(H2O)2]·3.5H2O}n (1)
A solution of H4btec (0.10 mmol) in 5 mL H2O was adjusted to pH 6 with a dilute NaOH solution. Then Cd(NO3)2·4H2O (0.20 mmol) in 5 mL H2O and obtz (0.20 mmol) in 5 mL MeOH were added with stirred. The mixture was sealed in a Teflon-lined stainless steel vessel (25 mL) and heated to 160 °C for 48 h. It was allowed to cool to room temperature (20 °C) at the rate of 5 °C per hour. Colorless crystals 1 were collected with a yield of 42% (based on obtz). Anal. calcd for C34H37Cd2N12O13.5: C, 38.72; H, 3.54; N, 15.94%. Found: C, 38.70; H, 3.57; N, 15.91%. IR data (cm−1): 3456w, 3131m, 1603w, 1559m, 1507s, 1442m, 1423s, 1369s, 1278m, 1246w, 1130m, 1008w, 987w, 927w, 889w, 804m, 778m, 733s, 719m, 670m, 647w.
X-Ray data collection and structure determination
Suitable single crystal of 1 was carefully selected under an optical microscope and glued to thin glass fiber. The diffraction data were collected using a Rigaku Mercury CCD with a graphite monochromated Mo Kα radiation (λ = 0.71073). Intensities were collected by the scan technique. The structure was solved by direct method and refined with full-matrix least-squares technique using SHELXL crystallographic software package.14 The parameters of the crystal data collection and refinement of 1 are given in Table 1, selected bond lengths and angles are listed in Table S1.†
Table 1 Crystallographic data for 1
Compound |
1 |
Formula |
C34H37Cd2N12O13.5 |
Fw |
1054.56 |
T/K |
293(2) |
Crystal system |
Monoclinic |
Space group |
C2/c |
a/Å |
27.108(8) |
b/Å |
10.673(2) |
c/Å |
16.641(5) |
α (°) |
90 |
β (°) |
104.241(8) |
γ (°) |
90 |
V/Å3 |
4667(2) |
F(000) |
2116 |
Z |
4 |
ρ (g cm−3) |
1.501 |
μ (mm−1) |
0.981 |
Reflections collected |
21 031 |
Unique reflections |
4237 (R(int) = 0.0852) |
Parameter |
313 |
Goodness of fit |
1.259 |
R1 [I > 2 s(I)] |
0.0857 |
wR2 (all data) |
0.1943 |
Results and discussion
Crystal structure of {[Cd2(obtz)2(btec)(H2O)2]·3.5H2O}n (1)
1 shows the unusual (4,4)-connected 3D network. The asymmetric unit of 1 contains one Cd(II) atom, one obtz, half btec, one coordination water and disordered lattice water. Each Cd(II) atom is six-coordinated by three carboxylic oxygen atoms from two btec ligands, one coordination water oxygen atom and two triazole nitrogen atoms from two obtz ligands in a distorted octahedral geometry (Fig. 1a). Two carboxylate groups of one btec ligands (O1O2, O1EO2E) exhibit the chelating coordination mode. Two other carboxylate groups of one btec ligands (O3O4, O3EO4E) exhibit the monodentate coordination mode. All btec ligand connect four Cd(II) atoms (the btec ligand is 4-connected.) and extend to form the [Cd2(btec)]n two dimensional network (Fig. 1b and S1 in ESI†). A one-dimensional channel is formed.
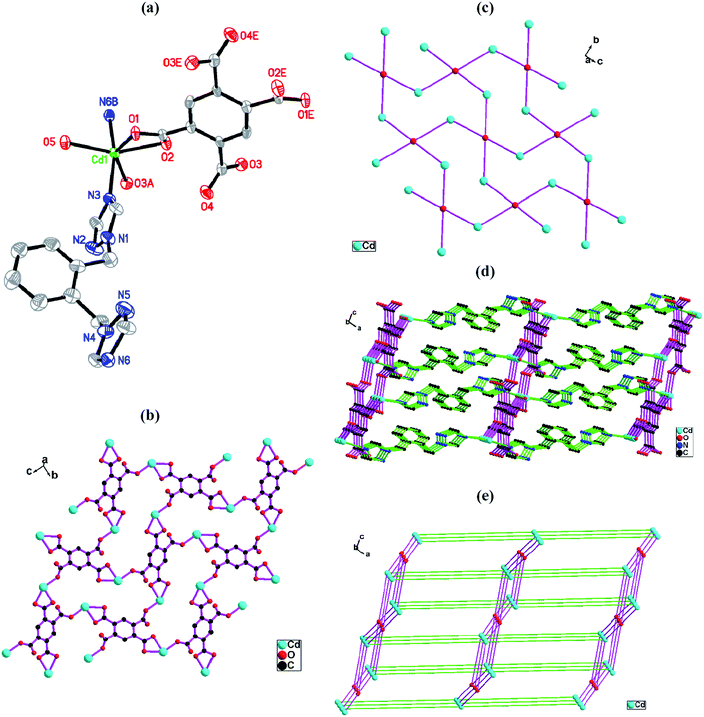 |
| Fig. 1 (a) The coordination environment of Cd(II) atom in 1, with displacement ellipsoids drawn at 30% probability level. (b) The [Cd2(btec)]n 2D network of 1. (c) and (d) The 3D network in 1 viewing along the b-axis and c-axis direction, respectively. (e) Schematic depiction of the (4,4)-connected 3D network of 1. The long bright green sticks represent the obtz ligands. The red balls present the 4-connected btec ligands. | |
The obtz ligand shows the anti–anti conformation and a 2-connected sticker and links two Cd(II) atoms with the Cd⋯Cd distance of 14.078(3). The [Cd2(btec)]n 2D networks are further linked by obtz ligands to build a 3D framework (Fig. 1c). Moreover, the large channel approximately 7 × 16 Å2 can be discovered along the c-axis.
Topologically, all Cd(II) atoms are 4-connected. All btec ligands are 4-connected. All obtz ligands are 2-connected. The 3D network in 1 can be described as the unusual (4,4)-connected 3D network with the Schläfli symbol of (62·8 × 103)2(63 × 103) analyzed by the TOPOS program (Fig. 1e).15 The coordination polymer showing such topo symbol is not reported as to the best of our knowledgement.
XRPD and luminescent properties
The measured and simulated PXRDs confirm the purity of 1 (Fig. S2 in ESI†). The luminescence of 1 and obtz ligand in the solid state was investigated at room temperature (Fig. 2). 1 exhibits a strong blue emission band maxima at 421 nm and a shoulder peak at 464 nm, upon the excitation at 325 nm. The free obtz ligand shows the emission maximum at 375 nm upon excitation at 290 nm or at 404 nm upon excitation at 350 nm. Emission was observed at 340 nm for free H4btec ligand as reported previously.16 Compared with the free obtz ligand or H4btec, the luminescence of 1 has red-shifted and stronger intensity, which can probably be attributed to the intra-ligand and ligand-to-ligand charge transition (LLCT).17
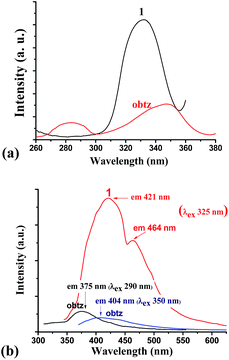 |
| Fig. 2 (a) Solid-state excitation spectra of 1 and the free obtz ligand at room temperature. (b) Solid-state emission spectra of 1 and the free obtz ligand at room temperature. | |
In order to study the luminescent sensitivity behavior of 1 for nitrobenzene, the luminescent properties of 1 dispersed in various kinds of solvents were investigated. The finely ground sample of 1 (3 mg) was dispersed in different solvents (3 mL) acetonitrile, methanol, ethanol, acetone, tetrahydrofuran (THF), nitrobenzene (NBZ), benzene (BZ) and carbon tetrachloride (CCl4), treated by ultrasonication for 30 minutes and aged for 1 day to form a stable solid layer and solution, respectively. The emission spectra upon the excitation at 325 nm exhibits the most significant enhancing and quenching effects, respectively (Fig. 3a). The relative intensities of 1 exposed to the above organic molecules are shown in Fig. 3b. The luminescence intensity of the 1 in THF, acetone and ethanol is obviously stronger than it in BZ, CCl4, NBZ. It is remarkable that the luminescence of 1 could be absolutely quenched in NBZ. The quenching behaviours in nitrobenzene (NBZ) could be attributed to the electron transfer from the electron donating frameworks of complexes to the highly electron deficient nitrobenzene that adhered to the surface of the MOF.9
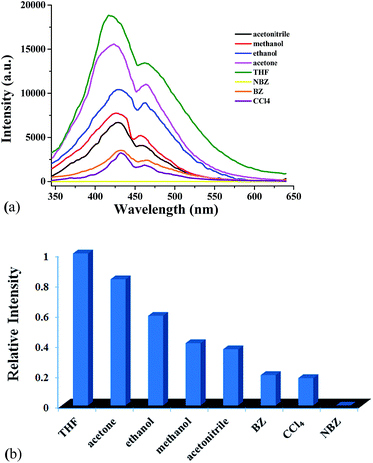 |
| Fig. 3 (a) Emission spectra of 1 in different solvents (excited at 325 nm). (b) Comparison of the relative luminescent intensities of 1 in different solvents. | |
Luminescent sensing of nitrobenzene
To explore the ability of 1 to detect nitrobenzene, the luminescence-quenching experiment was performed with incremental addition of nitrobenzene (NBZ) to 1 dispersed in acetone solution. 1 responds quickly to the nitrobenzene in acetone solution. The luminescent intensity of the suspension of 1@acetone gradually decreased with the concentration of NBZ increased (20, 40, 60, 80, 100, 120, 140, 160 ppm). High luminescence quenching was observed upon incremental addition of NBZ solution (Fig. 4a and b). The high quenching efficiency is up to 98.2% at 160 ppm NBZ.
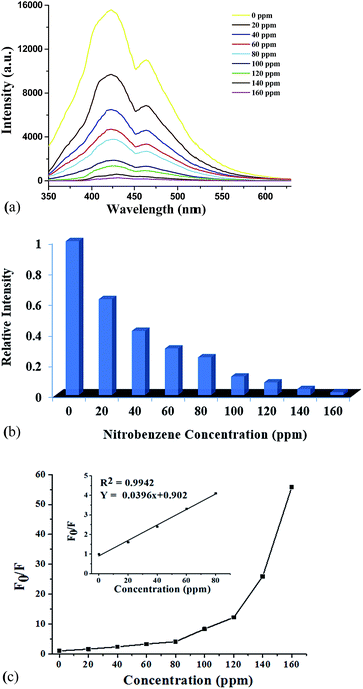 |
| Fig. 4 (a) Emission spectra of 1 dispersed in acetone in presence of different concentration of nitrobenzene solvent (excited at 325 nm). (b) Comparison of the relative luminescent intensities of 1@acetone in presence of different concentration of nitrobenzene solvent. (c) The relationship between F0/F and different concentration of NBZ (F0 and F refer to the luminescent intensity of 1@acetone at λex = 325 nm without and with NBZ, respectively). Insert: linear plot of F0/F and low NBZ concentration. | |
The quenching efficiencies could be shown by using the formula F0/F (F0 is the initial 1@acetone luminescent intensity without NBZ, F represents the fluorescence intensity with the increasing NBZ concentration). The F0/F and the increasing low concentration of NBZ (Fig. 4c), which has nearly linearly trend of the Stern–Volmer plot: F0/F = Ksv[A] + 1 ([A] is the increasing NBZ concentration, Ksv is the quenching constant). The quenching constant Ksv of 1 is 0.0396. The S–V plot for NBZ was nearly linear trend at low concentrations (≤80 ppm) and gradually deviated from linearity, the energy transfer or the combination of static and dynamic quenching mechanisms can explain this nonlinear phenomenon because of the presence of the inner filter effect.9g,18
The solid state UV-vis absorption spectra of 1, the free obtz and H4btec ligands were investigated at room temperature (Fig. 5). The UV-vis spectra exhibit absorption bands from 235 to 308 nm for 1, 238 to 279 nm for obtz, 238 to 308 nm for H4btec, which can be assigned to π–π* transition of the ligand.
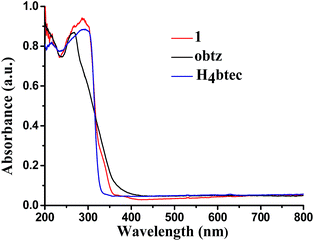 |
| Fig. 5 UV-vis absorption spectra of 1, the free obtz ligand and H4btec ligand at the solid state at room temperature. | |
UV-vis absorption spectra of 1 dispersed in acetone solvent (1@NBZ@acetone) in presence of different concentration of nitrobenzene (NBZ) was shown in Fig. S3 in ESI.† The absorption band from 330 to 370 nm gradually increased with the concentration of NBZ increased (20, 40, 60, 80, 100, 120, 140, 160 ppm), which can be assigned to NBZ sensor.
For comparison, the quenching efficiency of some luminescent MOF sensors for NBZ is summarized in Table 2. The high quenching efficiency of 1 is up to 98.2% at 160 ppm NBZ, which is comparable to MOF sensor [NH2(CH3)2][Cd17(L)12(μ3-H2O)4(DMF)2(H2O)2]·solvent in literature 10, and is high than other MOF sensors for nitrobenzene (NBZ) in Table 2.9,10,19,20 Recently, LaDuca and co-worker reported an interesting MOF sensor {[Cd(dms)(4-pmina)]·2.5H2O}n (dms = 2,2-dimethyl-succinate, 4-pmina = 4-pyridylmethylisonicotinamide) which exhibit 68% luminescent quenching efficiency when 100 uL DMSO solution of 1 × 10−4 M NBZ is added to 5 mL ethanol solution of 5 mg MOF sensor.21
Table 2 Summary of the quenching efficiency of luminescent MOF sensors for NBZa
MOF |
Quantity (mg)/solvent (mL) |
NBZ concentration |
Quenching efficiency |
Ref. |
The values were estimated from the literature 9a. The values were estimated from the literature 9b. The quantity of MOF is missed in literature 9g. The values were estimated from the literature 9j. The volumes of solvents are missed in literature 9q. The quantity of MOF and the volume of solvent are missed in literature 10. The volume of solvent is missed in literature 20. |
[Tb(L1)2/3(L2)1/2(H2O)2]·2H2O |
3 mg/5 mL |
1000 ppm |
60%a |
9a |
{[(UO2)2(H2TTHA)(H2O)]·4,4′-bipy·2H2O}n |
2 mg/3 mL |
1000 ppm |
90%b |
9b |
[Zn3(TDPAT)(H2O)3] |
4 mg/4 mL |
5000 ppm |
90% |
9c |
{[Me2NH2]2[Zn5(L)2(H2O)4]·16H2O}n |
1.5 mg/5 mL |
2000 ppm |
98% |
9d |
[Cd(H2ttac)(bpp)]n |
5 mg/3 mL |
3050 ppm |
100% |
9e |
Mg4(L)(DMF)4(H2O)4(DMF)0.5 |
5 mg/3 mL |
1500 ppm |
100% |
9f |
[Cd3(NTB)2(DMA)3]·2DMA |
c/3 mL |
600 ppm |
79% |
9g |
[Zn2(L)(bipy)(H2O)2]·(H2O)3(DMF)2 |
3 mg/3 mL |
2000 ppm |
90% |
9h |
[Pb(bpp)3(H2sba)2] |
3 mg/3 mL |
700 ppm |
89.9% ± 1.8% |
9i |
[Eu(L)1.5(DEF)]n |
3 mg/5 mL |
970 ppmd |
100% |
9j |
[(CH3)2NH2][Zn3(HL)(H2O)2]·4H2O |
2 mg/3 mL |
3000 ppm |
99.3% |
9k |
[Zn2(trz)2(bpdc)]·DMA |
3 mg/3 mL |
500 ppm |
89% |
9l |
Tb3+@NENU-522 |
3 mg/3 mL |
2000 ppm |
100% |
9m |
[Cd(ppvppa)(1,4-NDC)]n |
2 mg/2 mL |
800 ppm |
94% |
9n |
Cd2.5Na(NTB)2(DMF)4]·3DMF |
0.3 mg/3 mL |
500 ppm |
83% |
9o |
[Zn3(HL)2(fma)2]·DMA·H2O |
0.3 mg/3 mL |
300 ppm |
92% |
9p |
[NH2(CH3)2][Cd6(L)4(DMF)6(HCOO)] |
3 mg/e |
300 ppm |
97% |
9q |
[NH2(CH3)2][Cd17(L)12(μ3-H2O)4(DMF)2(H2O)2]·solvent |
f |
100 ppm |
92.5% |
10 |
[(CH3)2NH2]4[Cd3(H2L)] |
2 mg/3 mL |
1000 ppm |
92% |
19 |
{[Zn(L)]·DMA}n |
5 mg/g |
750 ppm |
93% |
20 |
{[Cd2(obtz)(Meip)2]·H2O}n |
3 mg/3 mL |
160 ppm |
98.2% |
This work |
The detection of NBZ using 1 as sensor is fully reversible. After quenching, the luminescence of 1 can be recovered by simply washing the sample with acetone (3 mg sample, 3 mL acetone solvent was used under ultrasonic washing condition for two minutes several times). As depicted in Fig. 6, after 5 time recycles, 1 showed almost identically rapid and evident responses to NBZ. This recycle experiment shows that 1 can be reused for the detection of NBZ.
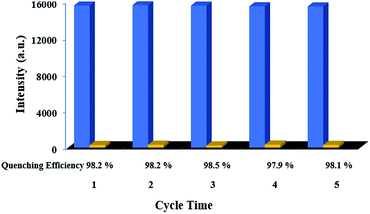 |
| Fig. 6 Reproducibility of the quenching ability of 1 dispersed in acetone and in the presence of 160 ppm NBZ. | |
To better understand the high selectivity of 1 towards NBZ, the quenching mechanism was investigated. Because 1 is the porous framework, which can provide the channels for NBZ. The quenching mechanism could be attributed to the electron transfer from the electron donating framework of MOF to the highly electron deficient nitrobenzene (NBZ) that adhered to the surface or in the MOF. The HOMO and LUMO energies of electron-deficient nitrobenzene and electron-rich obtz ligand were calculated by density functional theory at the level of B3LYP/6-31G*.19,20 The obtz ligand gives the LUMO energy −0.714 eV and HOMO energy −6.966 eV (Fig. 7). However nitrobenzene shows the LUMO energy −2.435 eV and HOMO energy −7.601 eV. The LUMO energy of obtz (−0.714 eV) is higher than the LUMO energy (−2.435 eV) of electron-deficient nitrobenzene. Therefore, the excited state electron from LUMO orbit of MOF can easily transfer to the LUMO orbit of nitrobenzene, which can explain the luminescent quenching phenomenon by frontier molecular orbital theory.
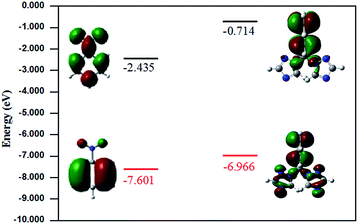 |
| Fig. 7 The molecular orbital energy of nitrobenzene and obtz ligand. | |
Conclusions
A porous metal–organic framework (MOF) 1 was successfully synthesized and characterized. 1 shows an unusual (4,4)-connected 3D network. 1 exhibits strong blue emission band maxima at 421 nm and a shoulder peak at 464 nm, upon the excitation at 325 nm in the solid state. The luminescent properties of 1 dispersed in various kinds of solvents were investigated in detail. 1 responds quickly to the nitrobenzene (NBZ) in acetone solution. The high quenching efficiency is up to 98.2% at 160 ppm NBZ. 1 can act as a high efficient luminescent sensor for detection of nitrobenzene and can be reused by simply washing with fresh solvent.
Acknowledgements
This work is supported by the Natural Science Foundation of China (No. 21171126), the Priority Academic Program Development of Jiangsu Higher Education Institutions, State and Local Joint Engineering Laboratory for Functional Polymeric Materials and Key laboratory of Organic Synthesis of Jiangsu Province.
References
-
(a) E. Coronado and M. Espallargas, Chem. Soc. Rev., 2013, 42, 1525 RSC;
(b) Y. L. Liu, J. F. Eubank, A. J. Cairns, J. Eckert, V. C. Kravtsov, R. Luebke and M. Eddaoudi, Angew. Chem., Int. Ed., 2007, 46, 3278 CrossRef CAS PubMed;
(c) S. T. Zheng, T. Wu, C. Chou, A. Fuhr, P. Y. Feng and X. H. Bu, J. Am. Chem. Soc., 2012, 134, 4517 CrossRef CAS PubMed.
-
(a) Q. P. Lin, T. Wu, S. T. Zheng, X. H. Bu and P. Y. Feng, Chem. Commun., 2011, 47, 11852 RSC;
(b) P. Y. Wu, C. He, J. Wang, X. J. Peng, X. Z. Li, Y. L. An and C. Y. Duan, J. Am. Chem. Soc., 2012, 134, 14991 CrossRef CAS PubMed.
-
(a) J. W. Liu, L. F. Chen, H. Cui, J. Y. Zhang, L. Zhang and C. Y. Su, Chem. Soc. Rev., 2014, 43, 6011 RSC;
(b) M. Y. Masoomi, S. Beheshti and A. Morsali, J. Mater. Chem. A, 2014, 2, 16863 RSC;
(c) A. Dhakshinamoorthy, M. Alvaro and H. Garcia, Chem. Commun., 2012, 48, 11275 RSC.
-
(a) M. M. Shi, C. H. Zeng, L. Wang, Z. W. Nie, Y. X. Zhao and S. L. Zhong, New J. Chem., 2015, 39, 2973 RSC;
(b) J. N. Hao and B. Yan, Chem. Commun., 2015, 51, 7737 RSC;
(c) S. K. Henninger, H. A. Habib and C. Janiak, J. Am. Chem. Soc., 2009, 131, 2776 CrossRef CAS PubMed;
(d) C. Janiak and J. K. Vieth, New J. Chem., 2010, 34, 2366 RSC;
(e) P. Ramaswamy, N. E. Wong and G. K. H. Shimizu, Chem. Soc. Rev., 2014, 43, 5913 RSC;
(f) B. Bhattacharya, D. Saha, D. K. Maity, R. Dey and D. Ghoshal, CrystEngComm, 2014, 16, 4783 RSC.
- Agency for Toxic Substance and Disease Registry, U.S. Toxicological profile of nitrobenzene, Department of Health and Human Services, Public Health Services, Atlanta, GA, 1990 Search PubMed.
-
(a) S. Dang, E. Ma, Z. M. Sun and H. Zhang, J. Mater. Chem., 2012, 22, 16920 RSC;
(b) W. Yang, J. Feng and H. Zhang, J. Mater. Chem., 2012, 22, 6819 RSC;
(c) Z. C. Liu, Z. Y. Yang, T. R. Li, B. D. Wang, Y. Li, D. D. Qin, M. F. Wang and M. H. Yan, Dalton Trans., 2011, 40, 9370 RSC;
(d) Z. Hao, X. Song, M. Zhu, X. Meng, S. Zhao, S. Su, W. Yang, S. Song and H. Zhang, J. Mater. Chem. A, 2013, 1, 11043 RSC.
-
(a) S. Liu, Z. Xiang, Z. Hu, X. Zheng and D. Cao, J. Mater. Chem., 2011, 21, 6649 RSC;
(b) S. Bai, T. Sheng, C. Tan, Q. Zhu, Y. Huang, H. Jiang, S. Hu, R. Fu and X. Wu, J. Mater. Chem. A, 2013, 1, 2970 RSC;
(c) Y. Q. Chen, G. R. Li, Z. Chang, Y. K. Qu, Y. H. Zhang and X. H. Bu, Chem. Sci., 2013, 4, 3678 RSC;
(d) B. L. Chen, L. B. Wang, F. Zapata, G. D. Qian and E. B. Lobkovsky, J. Am. Chem. Soc., 2008, 130, 6718 CrossRef CAS PubMed.
-
(a) X. G. Liu, H. Wang, B. Chen, Y. Zou, Z. G. Gu, Z. J. Zhao and L. Shen, Chem. Commun., 2015, 51, 1677 RSC;
(b) Z. Y. Guo, X. Z. Song, H. P. Lei, H. L. Wang, S. Q. Su, H. Xu, G. D. Qian, H. J. Zhang and B. L. Chen, Chem. Commun., 2015, 51, 376 RSC;
(c) G. Lu and J. T. Hupp, J. Am. Chem. Soc., 2010, 132, 7832 CrossRef CAS PubMed;
(d) S. Khatua, S. Goswami, S. Biswas, K. Tomar, H. Jena and S. Konar, Chem. Mater., 2015, 27, 5349 CrossRef CAS;
(e) J. H. Wang, M. Li and D. Li, Chem. Sci., 2013, 4, 1793 RSC.
-
(a) J. Z. Wang, W. Sun, S. Y. Chang, H. T. Liu, G. N. Zhang, Y. Q. Wang and Z. L. Liu, RSC Adv., 2015, 5, 48574 RSC;
(b) J. Song, X. Gao, Z. N. Wang, C. R. Li, Q. Xu, F. Y. Bai, Z. F. Shi and Y. H. Xing, Inorg. Chem., 2015, 54, 9046 CrossRef CAS PubMed;
(c) D. X. Ma, B. Y. Li, X. J. Zhou, Q. Zhou, K. Liu, G. Zeng, G. H. Li, Z. Shi and S. H. Feng, Chem. Commun., 2013, 49, 8964 RSC;
(d) X. Y. Wan, F. L. Jiang, L. Chen, J. Pan, K. Zhou, K. Z. Su, J. D. Pang, G. X. Lyu and M. C. Hong, CrystEngComm, 2015, 17, 3829 RSC;
(e) L. Y. Pang, G. P. Yang, J. C. Jin, M. Kang, A. Y. Fu, Y. Y. Wang and Q. Z. Shi, Cryst. Growth Des., 2014, 14, 2954 CrossRef CAS;
(f) S. L. Xie, H. F. Wang, Z. H. Liu, R. Dai and L. Z. Huang, RSC Adv., 2015, 5, 7172 Search PubMed;
(g) X. L. Hu, F. H. Liu, C. Qin, K. Z. Shao and Z. M. Su, Dalton Trans., 2015, 44, 7822 RSC;
(h) G. Y. Wang, L. L. Yang, Y. Li, H. Song, W. J. Ruan, Z. Chang and X. H. Bu, Dalton Trans., 2013, 42, 12865 RSC;
(i) B. B. Liu, X. L. Lin, H. Li, K. X. Li, H. Huang, L. Bai, H. L. Hu, Y. Liu and Z. H. Kang, Cryst. Growth Des., 2015, 15, 4355 CrossRef CAS;
(j) S. N. Zhao, X. Z. Song, M. Zhu, X. Meng, L. L. Wu, S. Y. Song, C. Wang and H. J. Zhang, RSC Adv., 2015, 5, 93 RSC;
(k) H. Zhang, W. Jiang, J. Yang, Y. Y. Liu, S. Y. Song and J. F. Ma, CrystEngComm, 2014, 16, 9939 RSC;
(l) F. H. Liu, C. Qin, Y. Ding, H. Wu, K. Z. Shao and Z. M. Su, Dalton Trans., 2015, 44, 1754 RSC;
(m) W. Xie, S. R. Zhang, D. Y. Du, J. S. Qin, S. J. Bao, J. Li, Z. M. Su, W. W. He, Q. Fu and Y. Q. Lan, Inorg. Chem., 2015, 54, 3290 CrossRef CAS PubMed;
(n) M. M. Chen, X. Zhou, H. X. Li, X. X. Yang and J. P. Lang, Cryst. Growth Des., 2015, 15, 2753 CrossRef CAS;
(o) X. L. Hu, C. Qin, X. L. Wang, K. Z. Shao and Z. M. Su, New J. Chem., 2015, 39, 7858 RSC;
(p) X. L. Hu, C. Qin, L. Zhao, F. H. Liu, K. Z. Shao and Z. M. Su, RSC Adv., 2015, 5, 49606 RSC;
(q) D. Tian, R. Y. Chen, J. Xu, Y. W. Li and X. H. Bu, APL Mater., 2014, 2, 124111 CrossRef.
- D. Tian, Y. Li, R. Y. Chen, Z. Chang, G. Y. Wang and X. H. Bu, J. Mater. Chem. A, 2014, 2, 1465 CAS.
-
(a) G. Aromí, L. A. Barrios, O. Roubeau and P. Gamez, Coord. Chem. Rev., 2011, 255, 485 CrossRef;
(b) M. L. Han, J. G. Wang, L. F. Ma, H. Guo and L. Y. Wang, CrystEngComm, 2012, 14, 2691 RSC;
(c) N. Wang, J. G. Ma, W. Shi and P. Cheng, CrystEngComm, 2012, 14, 5198 RSC;
(d) J. Zhao, D. S. Li, X. J. Ke, B. Liu, K. Zou and H. M. Hu, Dalton Trans., 2012, 41, 2560 RSC;
(e) M. L. Han, J. G. Wang, L. F. Ma, H. Guo and L. Y. Wang, CrystEngComm, 2012, 14, 2691 RSC;
(f) X. R. Meng, Y. L. Song, H. W. Hou, H. Y. Han, B. Xiao, Y. T. Fan and Y. Zhu, Inorg. Chem., 2004, 43, 3528 CrossRef CAS PubMed.
-
(a) X. Zhu, S. Zhao, Y. F. Peng, B. L. Li and B. Wu, CrystEngComm, 2013, 15, 9154 RSC;
(b) J. G. Ding, X. Zhu, Y. F. Cui, N. Liang, P. P. Sun, Q. Chen, B. L. Li and H. Y. Li, CrystEngComm, 2014, 16, 1632 RSC;
(c) X. Zhu, Q. Chen, Z. Yang, B. L. Li and H. Y. Li, CrystEngComm, 2013, 15, 471 RSC;
(d) X. Zhu, L. Y. Wang, X. G. Liu, J. Wang, B. L. Li and H. Y. Li, CrystEngComm, 2011, 13, 6090 RSC;
(e) J. G. Ding, C. Yin, L. Y. Zheng, S. S. Han, B. L. Li and B. Wu, RSC Adv., 2014, 4, 24594 RSC;
(f) Y. F. Cui, P. P. Sun, Q. Chen, B. L. Li and H. Y. Li, CrystEngComm, 2012, 14, 4161 RSC;
(g) Y. F. Peng, S. Zhao, K. Li, L. Liu, B. L. Li and B. Wu, CrystEngComm, 2015, 17, 2544 RSC;
(h) S. S. Han, L. L. Shi, K. Li, S. Zhao, B. L. Li and B. Wu, RSC Adv., 2015, 5, 107166 RSC;
(i) L. Liu, Y. F. Peng, X. X. Lv, K. Li, B. L. Li and B. Wu, CrystEngComm, 2016, 18, 2490 RSC.
-
(a) J. L. Du, T. L. Hu, S. M. Zhang, Y. F. Zeng and X. H. Bu, CrystEngComm, 2008, 10, 1866 RSC;
(b) X. Zhu, P. P. Sun, J. G. Ding, B. L. Li and H. Y. Li, Cryst. Growth Des., 2012, 12, 3992 CrossRef CAS;
(c) S. Zhao, Y. Yang, Z. Yang, B. L. Li and Y. Zhang, J. Coord. Chem., 2013, 66, 789 CrossRef CAS.
-
(a) G. M. Sheldrick, Acta Crystallogr., Sect. A: Found. Crystallogr., 2008, 64, 112 CrossRef CAS PubMed;
(b) G. M. Sheldrick, Acta Crystallogr., Sect. C: Struct. Chem., 2015, 71, 3 CrossRef PubMed.
-
(a) V. A. Blatov, M. O'keeffe and D. M. Proserpio, CrystEngComm, 2010, 12, 44 RSC;
(b) E. V. Alexandrov, V. A. Blatov, A. V. Kochetkova and D. M. Proserpio, CrystEngComm, 2011, 13, 3947 RSC;
(c) M. O'Keeffe and O. M. Yaghi, Chem. Rev., 2012, 112, 675 CrossRef PubMed;
(d) V. A. Blatov, A. P. Shevchenko and D. M. Proserpio, Cryst. Growth Des., 2014, 14, 3576 CrossRef CAS.
-
(a) Q. Hua, Y. Zhao, G. C. Xu, M. S. Chen, Z. Su, K. Cai and W. Y. Sun, Cryst. Growth Des., 2010, 10, 2553 CrossRef CAS;
(b) S. S. Chen, R. Qiao, L. Q. Sheng, Y. Zhao, S. Yang, M. M. Chen, Z. D. Liu and D. H. Wang, CrystEngComm, 2013, 15, 5713 RSC.
-
(a) Y. Yang, P. Du, Y. Y. Liu and J. F. Ma, Cryst. Growth Des., 2013, 13, 4781 CrossRef CAS;
(b) H. H. Li, Z. Niu, L. Chen, H. B. Jiang, Y. P. Wang and P. Cheng, CrystEngComm, 2015, 17, 5101 RSC;
(c) L. Yang, C. Qin, B. Q. Song, X. Li, C. G. Wang and Z. M. Su, CrystEngComm, 2015, 17, 4517 RSC;
(d) Y. P. Xia, Y. W. Li, D. C. Li, Q. X. Yao, Y. C. Du and J. M. Dou, CrystEngComm, 2015, 17, 2459 RSC.
- S. Sanda, S. Parshamon, S. Biswas and S. Konar, Chem. Commun., 2015, 51, 6576 RSC.
- Y. C. He, H. M. Zhang, Y. Y. Liu, Q. Y. Zhai, Q. T. Sheng, S. Y. Song and J. F. Ma, Cryst. Growth Des., 2014, 14, 3174 CAS.
- D. M. Chen, X. Z. Ma, W. Shi and P. Cheng, Cryst. Growth Des., 2015, 5, 3999 Search PubMed.
- C. L. White and R. L. LaDuca, Inorg. Chim. Acta, 2016, 441, 169 CrossRef CAS.
Footnote |
† Electronic supplementary information (ESI) available: The bond lengths and angles, the figure of crystal, PXRD and X-ray crystallographic file in CIF format. CCDC 1471709. For ESI and crystallographic data in CIF or other electronic format see DOI: 10.1039/c6ra10664a |
|
This journal is © The Royal Society of Chemistry 2016 |