DOI:
10.1039/C6RA10199B
(Paper)
RSC Adv., 2016,
6, 43518-43525
Benzocyclohexane oxide derivatives and neolignans from Piper betle inhibit efflux-related resistance in Staphylococcus aureus†
Received
20th April 2016
, Accepted 26th April 2016
First published on 27th April 2016
Abstract
This research seeks to address the problem of methicillin-resistant Staphylococcus aureus (MRSA) by discovering synergistic antibacterial natural substances from traditional Chinese herbs using antibacterial bioassays. Six compounds, including three neolignans (−)-acuminatin (1), (−)-denudatin B (2), puberulin D (3), and three benzocyclohexane oxide derivatives ferrudiol (4), ellipeiopsol B (5) and zeylenol (6), were isolated and purified by silica gel and reverse-phase silica gel column chromatography, and the chemical structures were determined through NMR spectroscopy, MS, together with CD and calculated CD spectroscopic methods. Synergistic activity was determined using strain SA1199B, a strain that overexpresses the major S. aureus multidrug transporter, NorA. Compounds 1–6 showed synergistic activity combined with norfloxacin against SA1199B, with FICI values of 0.13, 0.25, 0.25, 0.52, 0.08 and 0.27 respectively. The synergistic effects of the benzocyclohexane oxide derivatives with norfloxacin were further demonstrated through strain growth kinetics experiments. In the mechanistic experiment, the compounds showed significant accumulation and/or inhibition effects for EtBr efflux in SA1199B. These active compounds showed no toxicity to HEK293T cells at a concentration of 100 μM in the cytotoxicity evaluation experiments. Combined with efflux pump inhibitors, classic antibiotics that are substrates for efflux pumps may yet play a role in the therapy of drug-resistant bacteria where a lower dose could result in improved safety.
1. Introduction
Over the past few decades, there has been a dramatic decrease in the number of pharmaceutical companies developing new antimicrobial drugs.1 Methicillin-resistant Staphylococcus aureus (MRSA) is still cause for concern due to the small number of compounds that can be used therapeutically for this organism and resistance associated with their use.2 A supplemental strategy to overcome resistance is to develop agents that can suppress bacterial resistance mechanisms, and such agents are known as resistance-modifying agents (RMAs).3 These compounds may work by synergism, which is defined as a phenomenon in which two or more different compounds are combined to enhance their individual activity,4 and this approach is now widely recognized and can be directly applied to the development of new pharmaceuticals.5
Whilst there are different kinds of mechanisms of drug-resistance, multidrug-resistance (MDR)-related efflux is a common and important one, and has been reported for many organisms, including bacteria, fungi and protozoa, and also as a mechanism of resistance in human tumor cells.6 Examples of efflux-related resistance mechanisms that have been reported for S. aureus include those conferred by QacA and NorA, which are MDR transporters.7 Fluoroquinolone resistance, facilitated by the NorA efflux protein,6 has been shown to be a particular risk factor for mortality associated with infections by S. aureus.8 Therefore, in our research, we have studied the effects of plant natural products on S. aureus strain SA1199B, which is fluoroquinolone resistant by means of increased NorA production as well as a critical amino acid substitution within the quinolone resistance-determining region of GrlA.9 We evaluated the in vitro synergism of extracts of Piper betle Linn. (Piperaceae) with the fluoroquinolone antimicrobial drug norfloxacin against S. aureus strains by the broth microdilution method.10
P. betle is widely distributed in Yunnan, Guangxi and the Guangdong province of China,11 and cultivated in India for its leaves, which are used for chewing.12 Experimentally, the extracts and herbal material of P. betle have been shown to possess many kinds of curative properties such as laxative, aphrodisiac, antimicrobial,13 antioxidant14 and to improve appetite.15 The plant is rich in phenolics,16 methyl piperbetol, piperol A and piperol B,17 and some benzocyclohexane oxide derivatives and neolignans.18 In this report, we studied the chemical constituents of P. betle, guided by an antibacterial synergistic assay, and a series of preliminary mechanistic experiments were carried out.
2. Materials and methods
2.1. General experimental instruments and materials
The silica gel (100–400 mesh) used in column chromatography was purchased from Qingdao Marine Chemical Plant, China. 1H and 13C NMR spectra were obtained on a Varian Mercury Plus 400 MHz spectrometer. EI-MS spectra were measured with an Agilent 5973N MSD mass spectrometer. IR spectra and UV spectra were recorded on an Avatar 360 ESP FTIR spectrophotometer and a HITACHI U-2900 spectropolarimeter, respectively. Fractions obtained from column chromatography were monitored by thin layer chromatography (TLC) (silica gel plate HGF254, Yantai Huangwu Chemical Plant, Yantai, China). Optical density was recorded on a Multiskan FC (Thermo Co. Ltd, USA), and fluorescence was recorded on a Tecan M 1000 plate reader (Tecan Co. Ltd., Switzerland).
2.2. Extraction and bioactivity-guided isolation
P. betle was collected in Mengla County, Yunnan Province of China, in December of 2002, and identified by Professor Xu You-Kai, Professor of Xishuangbanna Botanic Garden in Yunnan province, the Chinese Academic of Science. A voucher specimen (148888) was deposited at the Herbarium and used in identifying the plant by professor You-Kai, and in the same time, we also saved a plant sample in our lab in School of Pharmacy, Fudan University. 100 g air dried leaves of P. betle were extracted using 95% ethanol by maceration and 10.8 g resinous extract was obtained. The resulting resin was then extracted using chloroform by solid–liquid extraction and yielded a gum (3 g). The total chloroform extract, was dissolved in acetone and mixed with silica gel and then subjected to silica gel and eluted with a gradient of chloroform–acetone (99
:
1–7
:
3). Fractions A–H were obtained by TLC monitoring with similar eluents. All of the eight fractions were subjected to Middle Chromatogram Isolated (MCI) gel to remove chlorophyll. Under the guidance of the synergistic activity, some fractions were further separated selectively and six compounds were obtained. Compound 1, named (−)-acuminatin (8 mg) was isolated from fraction B by silica gel column chromatography with elution of petroleum ether–acetone (95
:
5). Compounds 2 and 3, named (−)-denudatin B (10 mg) and puberulin D (3 mg), were both obtained from fraction C. Compound 2 was isolated by forward silica gel column chromatography with elution of petroleum ether–ethyl acetate (9
:
1) while compound 3 was obtained by high-pressure liquid chromatography (HPLC) preparation with elution of methanol–water (8
:
2). Fraction E was further separated to obtain compound 4, named ferrudiol (12 mg), by silica gel column chromatography with elution of chloroform–acetone (95
:
5). Compounds 5 and 6, named ellipeiopsol B (20 mg) and zeylenol (35 mg), were isolated from fraction F by silica gel column chromatography and Sephadex LH-20 gel column chromatography. The structures of all the compounds were deduced from spectroscopic methods (NMR spectroscopy and mass spectrometry) and all the resulting data were in good agreement with the literature.
2.3. HPLC fingerprint
The chloroform extract and six compounds (1–6) were dissolved in methanol, respectively. Each solution (2 mg mL−1) was subjected on the HPLC (10 μL, Agilent 1200) using an autosampler at 25 °C. The specifications and models of using column are eclipse XDB-C18, 5 μm, 4.6 × 250 mm (Agilent). The eluent flow rate was set to 1.0 mL min−1 propelled by an Agilent 1200 series G1312B SL binary pump, with ultra-pure water and HPLC-grade methanol (solutions A and B) eluting using the gradient method: started with 50% B, changing to 70% B within 30 min, then changing to 90% B within 40 min, the total time was 75 min. UV detector was used and the signal of compounds was monitored at a wavelength of 254 nm.
2.4. Electronic circular dichroism (ECD) spectral calculations
The absolute configurations of the six compounds were predicted by a quantum chemical calculation using Gaussian 09.19 The geometries of all compounds were optimized at the B3LYP/6-31G level of density functional theory (DFT). The Electronic Circular Dichroism (ECD) spectra were then calculated using the time dependent DFT (TDDFT) method at the B3LYP/6-311++G** level in the solvent methanol.
2.5. Antibacterial minimum inhibitory concentration (MIC) assay
Five Staphylococcus aureus strains (SA1199B, XU212, RN4220, EMRSA-15 and EMRSA-16) were used in experiments, all of which had a drug-resistance phenotype. Strain SA1199B, which overexpresses the norA gene encoding the NorA MDR efflux pump, was the generous gift of Professor Glenn W. Kaatz.20 Strain XU212, which possesses the TetK tetracycline efflux protein, was provided by Dr Edet Udo.21 Strain RN4220, was provided by Dr Jon Cove.22 Strains EMRSA-15 and strain EMRSA-16, which are epidemic methicillin-resistant (MRSA) strains, were the kind gift of Dr Paul Stapleton.23
Minimum inhibitory concentration (MICs) values were determined following the guidelines of Clinical Laboratory Standards Institute.24 Mueller-Hinton broth (MHB; Oxoid), was used which was supplemented with 20 and 10 mg L−1 of Ca2+ and Mg2+ respectively. All bacterial suspensions were adjusted to 5 × 105 cfu mL−1 for the bioassay. 2% DMSO in broth served as the negative control and vancomycin (Sigma Chemical Co. Ltd) served as the positive control. All compounds and chloroform extract were dissolved in DMSO respectively and then diluted with MHB, added into 96-well microtiter plate, with a concentration of 512 mg L−1 in upmost row and each following row (B–H) contained half concentration of the previous one. Both controls and treatments were tested in duplicate. After incubation at 37 °C for 24 h, 20 μL of 3-[4,5-dimethylthiazol-2-yl]-2,5-diphenyltetrazolium bromide (MTT; Biosharp) solution (5 mg mL−1) was added into each well to determined MIC. MTT colorimetric assay was performed to determine the MIC values. The minimum concentrations for colour appearing from black to yellow (completely inhibited the visible bacterial growth) were recorded as the MIC values against test strains.
2.6. Synergy tests
The five drug-resistant S. aureus strains described above were used in broth microdilution antimicrobial checkerboard assays10,25 to examine for the presence of a synergistic interaction. The rows of a 96-well microtiter plate contained compounds isolated with a concentration of 128 mg L−1 in upmost row (A) and each following row (B–G) contained half concentration of the previous one. Row (H) contained no compounds but MHB. A similar procedure was carried out along columns (1–12) with concentration of specific antibiotics ranging from 256 to 0.5 mg L−1. All of the treatments were tested in duplicate wells. MTT colorimetric assay was performed to determine the MIC values, which were used to evaluate the effects of the combination of compounds and antibiotics by calculating the fractional inhibitory concentration index (FICI) according to the formula:
“Synergy” effects were defined when the FICI was less than or equal to 0.5. When the FICI was greater than 0.5 and less than or equal to 4.0 this was regarded as an “indifference” effect; whilst “antagonistic” effects were observed when the FICI was greater than 4.0.26 An isobologram was constructed to depict the results of the checkerboard assay and the FICI values.
2.7. EtBr efflux assay
Those compounds for which an adequate amount existed were chosen for an efflux assay as previously described.27 Test organisms were grown overnight in cation-adjusted MHB until the OD620 = 0.4 and then followed by the addition of carbonyl cyanide m-chlorophenylhydrazone (CCCP, Across Organic) and EtBr (Sigma) (final concentrations, 100 μM (20.5 mg L−1) and 25 μM (2.725 mg L−1), respectively). After incubation at room temperature for 20 min, 4 mL of the inocula was pelleted at 13
000g for 5 min and then re-suspended in 3 mL of fresh MHB containing CCCP or the tested compounds (final concentrations, all 100 μM) (35.6 mg L−1 for compound 2, 48.8 mg L−1 for compound 4 and 38.4 mg L−1 for compound 5 and 6). Fluorescence of the suspension was monitored continuously (excitation and emission wavelengths were 530 and 600 nm, respectively; slit width was 5 nm) every five min for one hour. All tested compounds and the control were measured in triplicate.
2.8. EtBr accumulation assay
Ferrudiol (4), ellipeiopsol B (5) and zeylenol (6) were subjected to the EtBr accumulation assay by the fluorometric method due to their adequate quantity. This assay was conducted according to a modification of reported methods.28 Organisms were grown overnight in cation-adjusted MHB until the OD620 reached 0.4. Then 3 mL of the inoculum was collected by centrifugation at 13
000g for 5 min and re-suspended using fresh MHB to adjust the OD620 to 0.4. Culture medium (50 μL) and an equal volume of CCCP and test compounds (final concentrations, all 100 μM) (20.5 mg L−1 for CCCP, 48.8 mg L−1 for compound 4 and 38.4 mg L−1 for compound 5 and 6) were added to a 96-well plate containing 100 μL of EtBr (final concentration, 12.5 μM) (1.36 mg L−1). The 96-well plate was filled with 200 μL of the suspension and monitored continuously by the fluorescence of the suspension (excitation and emission wavelengths were 530 and 600 nm, respectively; slit width was 5 nm) every five min for one hour. All tested compounds and the control were measured in triplicate.
2.9. Growth curves
Growth kinetics was determined according to Garvey29 with some modifications. The growth kinetics of strain SA1199B in the absence or presence of the benzocyclohexane oxide derivatives were determined by monitoring the optical density at 620 nm every 2 h at 37 °C for 12, 24, 36 and 48 h using a microwell reader (Multiskan FC, Thermo). The final concentration of compounds and norfloxacin both alone and in combination with each other were 128 mg L−1 and 4 mg L−1. All tested compounds and the control were measured in triplicate.
2.10. Cytotoxicity evaluation
To determine whether the benzocyclohexane oxide derivatives were toxic, a cytotoxicity assay using a human embryonic kidney cell line (HEK293T) with the method of the 3-(4,5-dimethylthiazol-2-yl)-2,5-diphenyltetrazolium bromide (MTT) assay was carried out and the cell viability was measured according to Lu with some modifications.30 Cells were plated into 96-well plates at a cell density of 5 × 103 cells per well and allowed to grow in a 5% CO2 incubator. After 24 h, the medium was removed and replaced by fresh medium containing the tested compounds that were dissolved in DMSO and diluted to various concentrations (final concentrations were 100, 30, 10, 3, 1 μM respectively) with complete medium (RPMI 1640 + 10% FBS) before the experiment, and the final concentration of DMSO was 0.1%. After 24 hours incubation, cultures were incubated in 200 μL of medium with 20 μL of 5 mg mL−1 MTT solution for 4 hour at 37 °C. The medium with MTT was removed, and 150 μL of DMSO was added to each well to dissolve the formazan. The absorbance at 490 nm was measured with a microwell reader (Multiskan FC, Thermo). The inhibitory percentage of each compound at various concentrations was calculated, and the IC50 value was determined. All tested compounds and the control were measured in triplicate.
3. Results
3.1. Extraction and isolation
Compounds 1–6 were identified as the known chemical constituents (−)-acuminatin (1),31 (−)-denudatin B (2),31 puberulin D (3),32 ferrudiol (4),33–35 ellipeiopsol B (5)34,35 and zeylenol (6)34,35 (Fig. 1) by comparison of their physical properties and spectral data (ESI Tables S1 and S2†) with the literature. The chemical systematic name of the compound 3 is (7S,8S,1′R,5′R,6′S)-Δ2′,8′-6′-acetoxy-3′-methoxy-3,4-methylenedioxy-4′-oxo-8.1′,7.5′-neolignan,32 which was given a trivial name as puberulin D in this manuscript. The absolute configuration of compounds 1–6 were determined by comparison of their circular dispersion (CD) spectra with those reported and with the quantum chemical calculated CD spectra (ESI Fig. S1†). The purities of all six compounds were ≥95% as determined by HPLC (Fig. S2†).
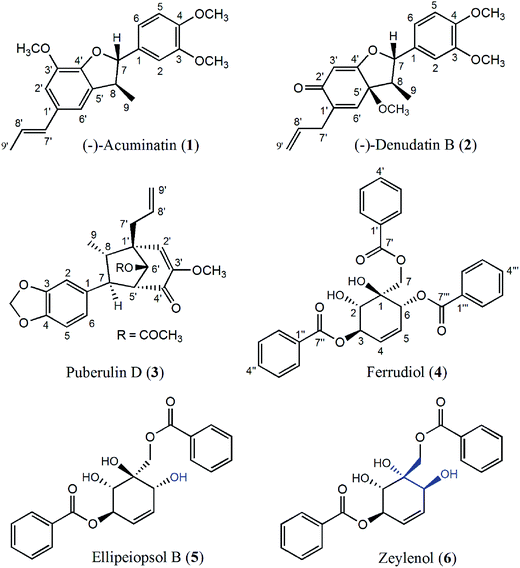 |
| Fig. 1 Chemical structures of compounds 1–6 isolated from Piper betle. | |
3.2. HPLC fingerprint
The HPLC fingerprint with well resolved peak separation for the chloroform extract of P. betle was obtained (Fig. 2). Compounds 1–6 were unambiguously recognized in the fingerprint by comparing with their retention time (Fig. 2). The retention time of (−)-acuminatin (1) was 41.473, which is the peak with retention time 41.418 in the fingerprint. We can were also able to identify the peaks for (−)-denudatin B (2), puberulin D (3), ferrudiol (4), ellipeiopsol B (5) and zeylenol (6) in the fingerprint with a retention time 31.431 min, 29.531 min, 39.988 min, 16.740 min and 11.281 min, respectively. The relative percentage contents of compounds 1–6 were 6.3%, 9.4%, 4.1%, 10.5%, 9.5% and 11.3% respectively. Total contents of the six compounds were more than fifty percent.
 |
| Fig. 2 The HPLC fingerprint of chloroform extract. | |
3.3. Antibacterial minimum inhibitory concentration (MIC) assay
The chloroform extract and compounds 1–6 were assayed for antibacterial activity against all test strains of S. aureus, and none had any significant antibacterial effect in that all MICs exceeded 512 mg L−1.
3.4. Synergy tests
The five drug-resistant S. aureus strains described above and antibiotics to which the strains were resistant were employed. The results showed that compounds 1–6 showed significant synergistic effects with norfloxacin against strain SA1199B, which overexpresses the norA gene encoding the NorA MDR efflux pump, but no synergetic effects were observed for the other four strains (XU212, RN4220, EMRSA-15 and EMRSA-16). (−)-Acuminatin (1), (−)-denudatin B (2), puberulin D (3), ferrudiol (4), ellipeiopsol B (5) and zeylenol (6) reduced the MIC of norfloxacin against SA1199B by eight-, four-, four-, two-, sixteen- and eight-fold respectively. This resulted in a concentration decrease of the antibiotic from 64 to 8 mg L−1, 128 to 32 mg L−1, 64 to 16 mg L−1, 64 to 32 mg L−1, 64 to 4 mg L−1 and 64 to 8 mg L−1, respectively (Table 1). The FICI of the combinations were 0.13, 0.25, 0.25, 0.52, 0.08, 0.27, respectively and we were able to construct an isobologram depicting the synergistic effects for the six compounds (ESI Fig. S3†).
Table 1 Minimum inhibitory concentrations (MIC) and fractional inhibition concentration indices (FICI) of six compounds from Piper betle for S. aureus SA1199Ba
Drug-resistant strain |
Agent |
MIC (mg L−1) |
FIC |
FICI |
Alone |
Combination |
(1) FICI ≤ 0.5 is defined as synergy; 0.5 < FICI ≤ 1 as additive; 0.5 < FICI ≤ 4 as indifferent; 4 < FICI as antagonistic. (2) All six compounds have no direct inhibitory effects on five strains and no synergetic effects combined with the other four strains, so data about those was not listed in above table. |
SA1199B |
(−)-Acuminatin (1) |
>512 |
2 |
<0.004 |
<0.13 |
Norfloxacin |
64 |
8 |
0.125 |
(−)-Denudatin B (2) |
>512 |
2 |
<0.004 |
<0.25 |
Norfloxacin |
128 |
32 |
0.25 |
Puberulin D (3) |
>512 |
2 |
<0.004 |
<0.25 |
Norfloxacin |
64 |
16 |
0.25 |
Ferrudiol (4) |
>512 |
8 |
<0.02 |
<0.52 |
Norfloxacin |
64 |
32 |
0.5 |
Ellipeiopsol B (5) |
>512 |
8 |
<0.02 |
<0.08 |
Norfloxacin |
64 |
4 |
0.06 |
Zeylenol (6) |
>512 |
8 |
<0.02 |
<0.27 |
Norfloxacin |
64 |
16 |
0.25 |
3.5. EtBr efflux assay
All compounds were subjected to an EtBr efflux assay by the fluorometric method without (−)-acuminatin (1) and puberulin D (3) due to their limited quantity. As shown in Fig. 3, ferrudiol (4), ellipeiopsol B (5) and zeylenol (6) had moderate efflux pump inhibitory effects against SA1199B (Fig. 3a, b and d), whereas (−)-denudatin B (2) demonstrated a strong efflux inhibitory effect against this strain (Fig. 3c). Within the first half hour, ferrudiol (4) showed a similar efflux pump inhibitory effect compared with the positive control CCCP, while a stronger effect than CCCP over the subsequent half hour was observed (Fig. 3a). Zeylenol (6) showed a similar activity compared with CCCP within one hour (Fig. 3b) and (−)-denudatin B (2) exhibited a stronger activity than CCCP within one hour (Fig. 3c). Ellipeiopsol B (5) showed a stronger activity than CCCP, but with a weaker effect than CCCP over the second half hour (Fig. 3d).
 |
| Fig. 3 EtBr efflux inhibitory effects of ferrudiol (4), zeylenol (6), (−)-denudatin B (2) and ellipeiopsol B (5) against S. aureus SA1199B. | |
3.6. EtBr accumulation assay
Ferrudiol (4), ellipeiopsol B (5) and zeylenol (6) exhibited EtBr accumulation effects with strain SA1199B (Fig. 4). They all increased the concentrations of EtBr compared with the vehicle control, especially ellipeiopsol B (5), showing stronger activity than the positive control CCCP (Fig. 4c). Zeylenol (6) had a similar activity compared with CCCP (Fig. 4b) and ferrudiol (4) showed a weaker activity compared to CCCP but with a significantly stronger effect than the control vehicle (Fig. 4a).
 |
| Fig. 4 EtBr accumulation effects of ferrudiol (4), zeylenol (6) and ellipeiopsol B (5) against S. aureus SA1199B. | |
3.7. Growth curves
Time-growth experiments of SA1199B in the absence or presence of norfloxacin and compounds alone and in combination with each other are shown in Fig. 5. The ordinate represents the increased several times of OD value. The results suggested that the increase of strain growth in a mixture of norfloxacin and compounds was less than strain growth with norfloxacin or compounds alone. All three of the tested compounds ferrudiol (4), ellipeiopsol B (5) and zeylenol (6) alone showed weak growth inhibitory activity within the first 30 h, while no activity within the last 18 h was observed. Interestingly, SA1199B with norfloxacin and the test compounds exhibited a lower OD value increase than with SA1199B and norfloxacin alone within 48 h (Fig. 5).
 |
| Fig. 5 Time-growth of SA1199B in the absence or presence of norfloxacin and benzocyclohexane oxide derivatives. | |
3.8. Cytotoxicity evaluation
In terms of the cytotoxic activity of the benzocyclohexane oxide derivatives, ferrudiol (4), ellipeiopsol B (5) and zeylenol (6) showed no toxicity with IC50 values within the concentration of 100 μM for HEK293T cells, a human embryonic kidney cell line. As shown in the ESI Fig. S5,† within the 1–100 μM L−1 (<48.8 mg L−1 for compound 4, <38.4 mg L−1 for compound 5 and 6) concentration range of all three compounds the viability of HEK293T was approximately 100%. The compounds on their own had no cytotoxicity and did not increase the toxicity of norfloxacin compared with norfloxacin alone within the antibacterial effect concentration (ESI Fig. S6†).
4. Discussion
In the primary experiment, the chloroform extract of Piper betle showed no direct bacterial growth inhibition but had a marginal synergistic effect together with the antibiotics. Under the guidance of a synergistic bacterial assay employing a checkerboard methodology in combination with chromatography, three neolignans [(−)-acuminatin (1), (−)-denudatin B (2) and puberulin D (3)] and three benzocyclohexane oxide derivatives [ferrudiol (4), ellipeiopsol B (5) and zeylenol (6)] were isolated from the extract. These compounds were isolated from other Piper species such as P. cubeba, P. puberulum and so on previously. All six compounds showed no direct bacterial inhibition but had significant synergistic activity with norfloxacin against strain SA1199B. The MIC values of the antibiotic combined with the compounds was several times less than that of the antibiotic alone (Table 1). The six compounds were known compounds, but there are no reports on their synergistic activities on drug-resistant strains. Considering the tested strain SA1199B overexpressing the NorA efflux pump, we postulated that the synergistic effects were as a result of NorA inhibition.
All tested compounds showed a similar inhibitory activity on strain SA1199B compared with the positive control (CCCP) in the EtBr efflux and accumulation assays (Fig. 3 and 4). These results indicated that efflux pump inhibition may contribute to the synergistic effects of these compounds against SA1199B.
In order to further confirm the synergistic effects, growth kinetics in the presence and absence of compounds were determined. The synergistic effects were obvious since the OD value increase of SA1199B with norfloxacin and compounds was lower than that with norfloxacin alone (Fig. 5), while the OD value of SA1199B with compounds alone was almost the same as SA1199B grown in isolation. The exact synergistic effects on the growth kinetics of the strain need further confirmation at the CFU level.
ESI Fig. S5 and S6† suggested that the synergistic activity of the compounds showed no inhibition on bacteria and no toxicity on mammalian cells within synergistic effect concentration, which was encouraging and warrants further investigation of the characteristics of these compounds as promising drug hits to overcome resistance in Staphylococcus aureus.
5. Conclusion
The conclusion can be drawn that these compounds may have specific inhibitory effects against the NorA efflux pump of strain SA1199B, which contributes to their synergistic effects with antibiotics against this strain. Synergy may be a promising strategy to resolve problems caused by drug-resistant strains because the compounds with synergistic effects will not cause a reduction of bacterial growth, reducing the likelihood of the formation of new drug-resistant mutants. Some of the classical antibiotics could be re-introduced into a clinical role in the therapy of infections caused by selected drug-resistant bacteria, resulting in the use of lower doses and potentially an improved safety profile. Furthermore, these compounds had no cytotoxic effects on the human embryonic kidney cell line (HEK293T) within the antibacterial effect concentration. This approach may have utility against multi-drug resistant Staphylococcus aureus.
Acknowledgements
This work was supported by the NSFC grant (21172041) of China.
References
- H. W. Boucher and G. H. Talbot, Clin. Infect. Dis., 2009, 48, 1–12 CrossRef PubMed.
- H. Grundmann, M. Aires-de-Sousa, J. Boyce and E. Tiemersma, Lancet, 2006, 368, 874–885 CrossRef.
- A. C. Abreu, A. J. McBain and M. Simose, Nat. Prod. Rep., 2012, 29, 1007–1021 RSC.
- E. M. Williamson, Phytomedicine, 2001, 8, 401–409 CrossRef CAS PubMed.
- H. Wagner and G. Ulrich-Merzenich, Phytomedicine, 2009, 16, 97–110 CrossRef CAS PubMed.
- S. Gibbons, M. Oluwatuyi and G. W. Kaatz, J. Antimicrob. Chemother., 2003, 51, 13–17 CrossRef CAS PubMed.
- T. G. Littlejohn, I. T. Paulsen, M. T. Gillespie, J. M. Tennent, M. Midgley, I. G. Jones, A. S. Purewal and R. A. Skurrsy, FEMS Microbiol. Lett., 1992, 74, 259–265 CrossRef CAS PubMed.
- M. Helms, S. Ethelberg, K. Mølbak and the DT104 Study Group, Emerging Infect. Dis., 2005, 11, 859–867 CrossRef PubMed.
- G. W. Kaatz and S. M. Seo, Antimicrob. Agents Chemother., 1997, 41, 2733–2737 CAS.
- S. Hemaiswarya, A. Kruthiventi and M. Doble, Phytomedicine, 2008, 15, 639–652 CrossRef CAS PubMed.
- Editorial Committee of Chinese Bencao of the State Administration of Traditional Chinese Medicine of the People's Republic of China., Zhong Hua Ben Cao, Shanghai Science and Technology Press, Shanghai, 1999.
- K. Ghosh and T. K. Bhattacharya, Molecules, 2005, 10, 798–802 CrossRef CAS PubMed.
- S. N. Tewari and M. Nayak, Trop. Agric., 1991, 68, 373–375 Search PubMed.
- L. Arambewela, M. Arawwawala and D. Rajapaksa, Int. J. Food Sci. Technol., 2006, 41, 10–14 CrossRef CAS.
- S. M. Prabu, M. Muthumani and K. Shagirtha, Saudi J. Biol. Sci., 2012, 19, 229–239 CrossRef CAS PubMed.
- C. K. Wang and M. J. Wu, J. Chin. Agric. Chem. Soc., 1996, 34, 638–647 CAS.
- H. W. Zeng, Y. Y. Jiang, D. G. Cai, J. Bian, K. Long and Z. L. Chen, Planta Med., 1997, 63, 296–298 CrossRef CAS PubMed.
- V. S. Parmar, S. C. Jain, K. S. Bisht, R. Jain, P. Taneja, A. Jha, O. D. Tyagi, A. K. Prasad, J. Wengel, C. E. Olsen and P. M. Boll, Phytochemistry, 1996, 46, 591–673 Search PubMed.
- M. J. Frisch, Gaussian09, (Revision B.01), Wallingford CT, 2009 Search PubMed.
- G. W. Kaatz, S. M. Seo and C. A. Ruble, Antimicrob. Agents Chemother., 1993, 37, 1086–1094 CrossRef CAS PubMed.
- E. E. Udo and S. Gibbons, Phytother. Res., 2000, 14, 139–140 CrossRef.
- J. I. Ross, A. M. Farrell, E. A. Eady, J. H. Cove and W. J. Cunliffe, J. Antimicrob. Chemother., 1989, 24, 851–862 CrossRef CAS PubMed.
- J. F. Richardson and S. Reith, J. Hosp. Infect., 1993, 25, 45–52 CrossRef CAS PubMed.
- Clinical and Laboratory Standards Institute, CLSI, M100-S25, Wayne, USA., PA, 2015.
- F. Iten, R. Saller, G. Abel and J. Reichling, Planta Med., 2009, 75, 1231–1236 CrossRef CAS PubMed.
- A. J. Hiyas, A. S. C. Arlene, A. E. Keivan, T. B. Johnna, T. M. Kathryn, N. G. Tyler, J. R. Scott, E. C. Robert, H. O. Nicholas and B. C. Nadja, J. Nat. Prod., 2011, 74, 1621–1629 CrossRef PubMed.
- D. Lechner, S. Gibbons and F. Bucar, J. Antimicrob. Chemother., 2008, 62, 345–348 CrossRef CAS PubMed.
- L. J. V. Piddock, M. I. Garvey, M. M. Rahman and S. Gibbons, J. Antimicrob. Chemother., 2010, 65, 1215–1223 CrossRef CAS PubMed.
- M. I. Garvey, M. M. Rahman, S. Gibbons and L. J. V. Piddock, Int. J. Antimicrob. Agents, 2011, 37, 145–151 CrossRef CAS PubMed.
- Y. L. Lu, Y. K. Li, M. X. Li, D. Chen and T. Wu, Synth. React. Inorg., Met.-Org., Nano-Met. Chem., 2014, 44, 859–863 CrossRef CAS.
- Y. Ma, G. Q. Han and Y. Y. Wang, Acta Pharmacol. Sin., 1993, 28, 370–373 CAS.
- J. M. David, M. Yoshida and O. R. Gottlieb, Phytochemistry, 1993, 36, 491–499 CrossRef.
- L. Wirasathien, T. Pengsuparp, M. Moriyasu, K. Kawanishi and R. Suttisri, Acta Pharmacol. Sin., 2006, 29, 497–502 CAS.
- G. R. Schulte and B. Ganem, Tetrahedron Lett., 1982, 23, 4299–4302 CrossRef CAS.
- A. Kijjoa, J. Bessa, M. M. M. Pinto, C. Anatachoke, A. M. S. Silva, G. Eaton and W. Herz, Phytochemistry, 2002, 59, 543–554 CrossRef CAS PubMed.
Footnote |
† Electronic supplementary information (ESI) available. See DOI: 10.1039/c6ra10199b |
|
This journal is © The Royal Society of Chemistry 2016 |
Click here to see how this site uses Cookies. View our privacy policy here.