DOI:
10.1039/C6RA08710H
(Paper)
RSC Adv., 2016,
6, 51183-51191
A facile access to novel heterocyclic analogues of chalcone from newly synthesized ketone containing isoxazole and a benzoxazinone ring†
Received
5th April 2016
, Accepted 17th May 2016
First published on 19th May 2016
Abstract
A series of novel heterocyclic analogs of chalcone containing isoxazole and a benzoxazinone ring as a prime motif was rationally designed and synthesized. There is nothing in the literature about such α,β-unsaturated ketones. These compounds were synthesized using a Claisen–Schmidt condensation reaction of the ketone precursor with different aromatic aldehydes using ethanol as solvent and piperidine as a base. Our procedure offers easy access to isoxazole and benzoxazinone based chalcone derivatives providing yields in the range of 81–93% within 16 min under mild conditions. Out of 21, 11 compounds were screened for their antibacterial activities. Among the screened compounds, compounds 5aj, 5ak and 5bj showed excellent antibacterial activities with MIC: 0.34, 0.59 and 0.74 mg ml−1 respectively as compared to standard drugs.
1. Introduction
Chalcones are important naturally occurring plant constituents and common structures and precursors in biologically active heterocycles, in particular, of benzothiazepines, pyrazolines, pyrimidines, isoxazolines, flavonoids and isoflavonoids. These display a wide range of pharmaceutical, extensive agricultural and synthetic applications such as, anti-inflammatory,1a antifungal,1b antibacterial,1c antiviral,1d antiprotozoal,1e anticancerous,1f anti-tubercular,1g hyperglycemic agents,1h agrochemicals,1i scavenging,1j and PET scan imaging probes.1k From a synthetic point of view, chalcone derivatives are gaining more importance due to their critical role in organic synthesis. Chalcone moieties containing nitrogen heterocycles have been reported as active compounds against herpes simplex virus-1 (HSV-1)2a and human immunodeficiency virus-1 (HIV-1).2b These compounds also exhibit antiproliferative activity towards leukemia cell lines.2c
Isoxazole derivatives exhibit a unique and interesting class of five-membered heterocycle because of their synthetic versatility and effective biological activities. Heterocyclic compounds with isoxazole moiety were found to be valuable intermediates for medicinal drugs. They are related with a broad spectrum of biological and pharmaceutical activities such as anti-inflammatory,3a anticancer,3b analgesic,3c antiviral,3d antimicrobial,3e anthelmintic,3f anticonvulsant,3g antidepressant,3h potent selective agonists of the human cloned dopamine D4 receptor,3i antagonist activity3j and antinociceptive activity.3k
The benzoxazinone moiety has been extensively studied due to prevalence of these moieties in the cores of natural products and pharmaceuticals. The benzoxazinones produced by a wide range of plants including commercially important cereal crops such as rye, maize and wheat.4 Benzoxazinones are well-known to show a broad range of biological properties including antifeedant,5a antimicrobial,5b antiphlogistic5c and insecticidal effects.5d These are known for their unique chemical motif with potential pharmaceutical activity and have been recognised as “privileged scaffold” in terms of drugs design.6
Chalcones on integration with different biologically active frameworks have always shown comparative more potent activities; like benzofuran chalcones have exhibited both antioxidant and antibacterial activity. Benzimidazole – “a heterocyclic scaffold” is a widely used medicinal component due to its broad spectrum of biological activities. For example, there are some recent reports on the efficient ultrasound-assisted or solvent-free Claisen–Schmidt synthesis of heterocycle-chalcone hybrids, such as quinoline chalcones,7 pyrazole chalcones8 and quinoline-appended ferrocenyl chalcones.9 Heterocyclic rings especially the benzoxazinone and isoxazole rings, represent an expedient choice for the synthesis of pharmaceutical compounds with different activities and noble safety profiles. As a consequence, the remarkable bioactivity along with the unique structural features displayed by these heteroaryl-based chalcones makes them a particularly attractive target for the synthetic efforts.
On account of these aspects and in order to expand the structure diversity for current medicinal chemistry needs, we would like to report here an efficient, and the detailed synthetic approach for the synthesis of novel isoxazole and benzoxazinone-based chalcone derivatives, namely 6-bromo-4-(3-(3-(4-substitutedphenyl)acryloyl)-4,5-dihydroisoxazol-5-yl)-4-methyl-1H-benzo[d][1,3]oxazin-2(4H)-one through Claisen–Schmidt condensation reaction of 4-((3-acetyl-4,5-dihydroisoxazol-5-yl)methyl)-6-bromo-4-methyl-1H-benzo[d][1,3]oxazin-2(4H)-one and various aromatic aldehydes with piperidine as base and ethanol as a solvent for 10–16 min under microwave irradiation providing 81–93% yield.
Many methods have been developed for the synthesis of chalcone derivatives, to the best of our knowledge, the synthesis of isoxazole and benzoxazinone-based chalcones under microwave irradiation has not been reported. To contribute to the development of environmentally benign organic chemistry, and in the context of our ongoing work on the ‘green’ synthesis of potential biologically active heterocycles,10 we have explored the above Claisen–Schmidt condensation reaction with the aim of providing a simple method for the synthesis of novel isoxazole and benzoxazinone-based chalcone derivatives.
2. Results and discussion
2.1. Chemistry
Chalcones 5aa–ak and 5ba–bj were synthesized via Claisen–Schmidt condensation reaction with substituted benzaldehyde and 5a or 5b, piperidine in ethanol, at 80 °C for 15 min under microwave irradiation (Scheme 1). After completion of the reaction, the mixture was filtered to collect the precipitates and purification by recrystallization affords the pure chalcones 5aa–ak and 5ba–bj in 81–93% yield. The compounds 5aa–ak and 5ba–bj were obtained from corresponding ketones (5a and 5b). The intermediate ketones were produced in a four-step synthesis (Scheme 1).
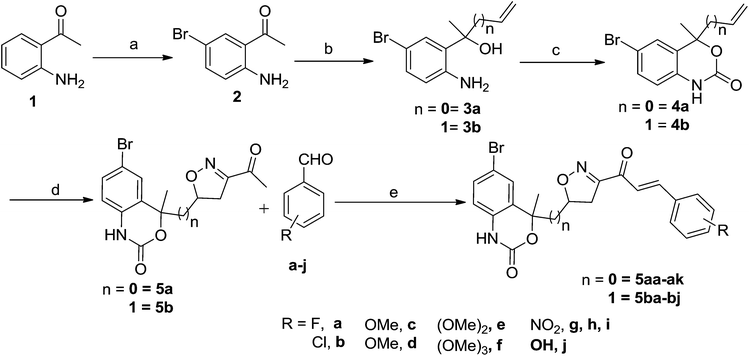 |
| Scheme 1 Synthesis of benzoxazinone–isoxazole endowed chalcone. Reagents and condition: (a) NBS, acetonitrile, 3 h (b) allyl or vinylmagnesium bromide, THF, −78 °C, inert, 5 h (c) carbonyldiimidazole, THF, 60 °C, 12 h (d) Ceric ammonium nitrate, acetone, 60 °C, overnight (e) ethanol, piperidine, MW 80 °C, 10–16 min. | |
In the first step, the commercially available starting material 2-aminoacetophenone (1) was transformed into 5-bromo-2-aminoacetophenone (2) by using N-bromosuccinimide in acetonitrile at 0 °C in high yields (92%).11 Then compound 2 was treated with vinyl or allylmagnesium bromide to furnish corresponding products 3a or 3b respectively via Grignard reaction in excellent yield (95%). Next step of the synthesis was the conversion of compound 3a or 3b to their corresponding products 4a or 4b respectively using carbonyldiimidazole in THF in 96% yield.12 They were then treated with ceric ammonium nitrate and acetone to obtain compounds 5a or 5b which were treated with various substituted aldehydes in ethanol/piperidine at 80 °C to obtain the final compounds 5aa–ak and 5ba–bj in good to excellent yields (81–93%).13 To find optimum protocol for the transformation various conditions for a prototypical reaction were explored as shown in Table 1.
Table 1 Optimization of base and solventa
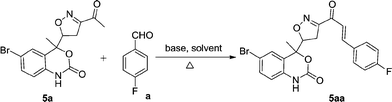
|
Entry |
Solvent |
Base |
Base equiv. |
Temp. (°C) |
Time (h) |
Yieldb (%) |
General condition: 4-(3-acetyl-4,5-dihydroisoxazol-5-yl)-6-bromo-4-methyl-1H-benzo[d][1,3]oxazin-2(4H)-one 5a (1 mmol), 4-fluro benzaldehyde a (1 mmol). Isolated yield. |
1 |
MeOH |
NaOH |
1 |
60 |
6 |
— |
2 |
EtOH |
KOH |
1 |
60 |
6 |
— |
3 |
t-BuOH |
t-BuOK |
1 |
80 |
5 |
— |
4 |
EtOH |
Pyridine |
2 |
Reflux |
6 |
45 |
5 |
t-BuOH |
Piperidine |
2 |
Reflux |
7 |
55 |
6 |
EtOH |
Piperidine |
1 |
Reflux |
8 |
67 |
7 |
EtOH |
Piperidine |
1.5 |
Reflux |
8 |
72 |
8 |
EtOH |
Piperidine |
2 |
Reflux |
6 |
85 |
9 |
EtOH |
Piperidine |
3 |
Reflux |
8 |
85 |
Initially, the reaction was carried out in methanol and ethanol in the presence of NaOH, KOH at 60 °C and in t-BuOK at 80 °C for 6 h (Table 1, entries 1, 2, 3). The reaction did not proceed, instead some decomposed products were obtained. Then different bases were examined and it was observed that pyridine promoted the reaction in low yields (45%, Table 1, entry 4). Among the bases screened, piperidine was found to be the most effective catalyst. The influence of solvent system was then evaluated. tert-Butanol furnished the desired product in low yield (55%, Table 1, entry 5).
To our delight, by using ethanol as solvent, moderate yield was achieved (67%, Table 1, entry 6). Regarding the influence of the solvent in this reaction, EtOH was achieved as optimal solvent (Table 1, entry 8). It can be easily rationalized that use of ethanol enhances the solubility of reagents and thus increased the product yield. It is important to note that when the amount of piperidine is increased from 1 to 1.5 eq., an increase in the yield was observed to 72% (Table 1, entry 7). Further increase in the amount of piperidine to 2 eq. the desired product 5aa was delivered in 85% yield (Table 1, entry 8). However, when 3 eq. of piperidine was taken in the reaction system, the product yield remained unchanged (85%, Table 1, entry 9).
With the optimal reaction condition regarding base and solvent in hands, we thought to explore different reaction conditions and temperature for the reaction (Table 2). The physical grinding of substrates with piperidine in a mortar and pestle for 1 h (monitored by TLC) without any solvent at room temperature did not result in appreciable yield of the product (Table 2, entry 1). The same reaction took around 6 h under conventional heating (80 °C) to afford 5aa in 85% yield as discussed above (Table 2, entry 2). However, increase or decrease in temperature of the reaction system to 70 or 90 °C, a decrease in the yield was observed (Table 2, entry 3, 4). On the other hand, when the same reaction is done under solvent free thermal heating condition, the desired product was furnished in moderate yield (67% Table 2, entry 5).
Table 2 Optimization of reaction temperature and conditionsa,b,c,d,e
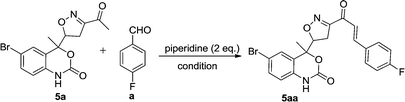
|
Entry |
Condition |
Temp. (°C) |
Time (min) |
Yielde (%) |
General condition: 4-(3-acetyl-4,5-dihydroisoxazol-5-yl)-6-bromo-4-methyl-1H-benzo[d][1,3]oxazin-2(4H)-one 5a (1 mmol), benzaldehyde a (1 mmol). Conventional heating. Anton Paar Monowave 300 reactor. Reaction carried out in neat condition. Isolated yield. |
1 |
Grinding |
Rt |
1 h |
—d |
2 |
CHb |
80 |
6 h |
85 |
3 |
CHb |
70 |
8 h |
71 |
4 |
CHb |
90 |
6 h |
80 |
5 |
CHb |
60 |
8 h |
67d |
6 |
MWc |
70 |
20 |
69 |
7 |
MWc |
80 |
15 |
90 |
8 |
MWc |
85 |
15 |
88 |
9 |
MWc |
90 |
15 |
84 |
10 |
MWc |
80 |
12 |
92 |
11 |
MWc |
80 |
10 |
79 |
12 |
MWc |
80 |
12 |
73d |
For comparison, the same reaction was subjected to microwave irradiation and effect of irradiation time and temperature on the efficiency and yield were briefly investigated. First, the effect of irradiation temperature was briefly examined. It was observed that yield of 5aa at 70 °C (69%) was abruptly increased to 90% by increasing the irradiation temperature up to 80 °C, but it became lower when the microwave temperature exceeded 80 °C (Table 2, entries 6, 7, 8, 9). Thus, 80 °C was chosen as the optimum irradiation temperature for 5aa. Then the effect of microwave irradiation time was studied, and 12 min was found to be the optimized reaction time for the formation of 5aa. So, we led to conclude that microwave irradiation at 80 °C for 12 minutes was the optimal condition for the reaction to get best yield of the product. Using microwave irradiation, an improvement in terms of yields and reaction time was achieved.
On the basis of these optimized conditions, various combinations of novel ketone 4a and 4b and substituted benzaldehydes were employed for the Claisen–Schmidt condensation reaction, allowing the reaction to be completed within 16 min in good yields of 81–93% and also high purities (Table 3).
Table 3 List of the synthesized benzoxazinone–isoxazole endowed chalcone derivativesa,b
Entry |
Ketone |
Aldehyde |
Product |
Time (min) |
Yieldc (%) |
General condition: 4-(3-acetyl-4,5-dihydroisoxazol-5-yl)-6-bromo-4-methyl-1H-benzo[d][1,3]oxazin-2(4H)-one 5a (1 mmol), benzaldehyde (a–k) (1 mmol), piperidine (2 mmol), ethanol. Anton Paar Monowave 300 reactor. Irradiation power: 850 W; ramp time: 1 min. 70 °C; holding temp: 80 °C. Isolated yield. |
1 |
5a |
 |
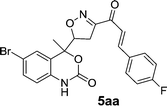 |
11 |
92 |
2 |
5a |
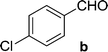 |
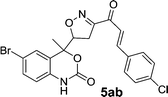 |
12 |
89 |
3 |
5a |
 |
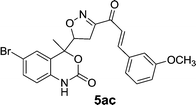 |
12 |
87 |
4 |
5a |
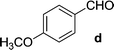 |
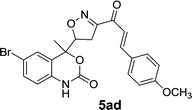 |
15 |
85 |
5 |
5a |
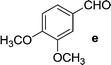 |
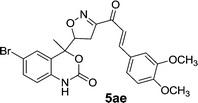 |
15 |
85 |
6 |
5a |
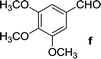 |
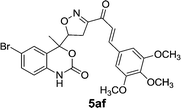 |
16 |
83 |
7 |
5a |
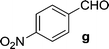 |
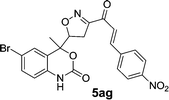 |
10 |
93 |
8 |
5a |
 |
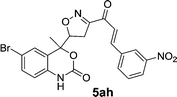 |
10 |
88 |
9 |
5a |
 |
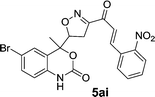 |
10 |
84 |
10 |
5a |
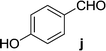 |
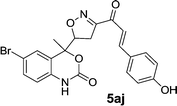 |
14 |
85 |
11 |
5a |
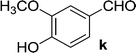 |
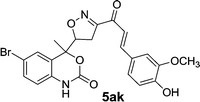 |
14 |
87 |
12 |
5b |
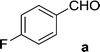 |
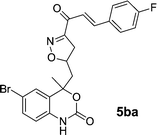 |
11 |
86 |
13 |
5b |
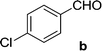 |
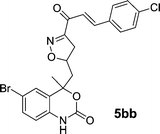 |
12 |
84 |
14 |
5b |
 |
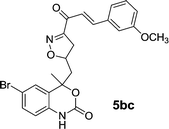 |
15 |
86 |
15 |
5b |
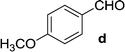 |
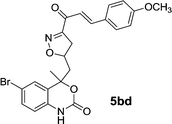 |
15 |
82 |
16 |
5b |
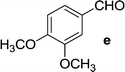 |
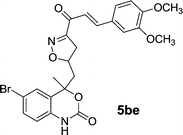 |
18 |
80 |
17 |
5b |
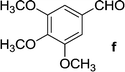 |
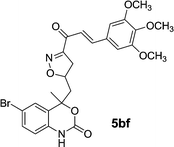 |
18 |
75 |
18 |
5b |
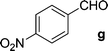 |
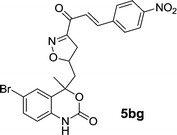 |
10 |
87 |
19 |
5b |
 |
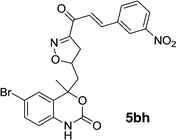 |
10 |
89 |
20 |
5b |
 |
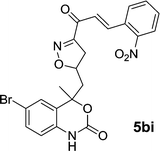 |
10 |
88 |
21 |
5b |
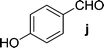 |
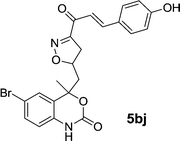 |
14 |
86 |
The purified products were characterized by FTIR, 1H NMR, 13C NMR, and HRMS. The IR spectra of compounds showed the characteristic band: the ν(C
O) peak observed at 1702 cm−1 shifts to a lower frequency of 1636–1655 cm−1 in chalcones. This is due to the conjugation of the π-electrons of the benzene moiety with those of the ethylene moiety in the enone linkage.
2.2. Biological activity
The newly synthesized compounds were evaluated for their in vitro antibacterial activity against S. aureus and B. subtilis as examples of Gram-positive bacteria and E. coli and S. flexneri as examples of Gram-negative bacteria. Out of these (Table 4) showing only 11 most potent antibacterial active compound.
Table 4 Minimum inhibitory concentration (MIC, mg mL−1) of some newly synthesized compounds against bacterial strains
Compound |
Gram-positive |
Gram-negative |
S. aureus |
B. subtilis |
E. coli |
S. flexneri |
5af |
1.78 |
3.10 |
1.45 |
2.23 |
5ag |
1.42 |
3.42 |
1.53 |
2.25 |
5ah |
6.2 |
6.45 |
7.8 |
6.5 |
5ai |
7.1 |
7.5 |
7. |
7.5 |
5aj |
0.34 |
3.12 |
0.34 |
0.95 |
5ak |
0.59 |
3.12 |
0.59 |
1.75 |
5bf |
2.12 |
3.75 |
2.25 |
2.15 |
5bg |
1.36 |
3.12 |
1.32 |
2.26 |
5bh |
6.8 |
7.1 |
7.2 |
7.5 |
5bi |
7.7 |
8.2 |
7.9 |
8.5 |
5bj |
0.74 |
3.10 |
0.72 |
1.55 |
Ampicillin |
0.78 |
0.39 |
1.56 |
0.78 |
Cefadroxil |
1.56 |
3.12 |
0.78 |
1.56 |
2.2.1. Antibacterial activity. As seen in the Table 4, compound 5aj (MIC: 0.34 mg ml−1) and compounds 5af, 5ag, 5ak, 5bg, 5bj (MIC: 1.78, 1.42, 0.59, 1.36, 0.74 mg ml−1) have shown better antibacterial activity than positive controls against S. aureus. In case of B. subtilis, all compounds have shown lower activity than ampicillin. Moreover, compounds 5af, 5aj, 5ak, 5bg, 5bj (MIC: 3.10–3.12 mg ml−1) were found to be equally potent as cefadroxil against B. subtilis. Compounds 5af, 5ag, 5aj, 5ak, 5bg, 5bj (MIC: 0.34–1.45 mg ml−1) have shown better activity than positive controls against E. coli while compounds 5af and 5ag (MIC: 1.45 and 1.53 mg ml−1) have shown approx. equal activity to the reference drugs. Compounds 5aj and 5bj have shown admirable antibacterial activity (MIC: 0.95 and 1.55 mg ml−1) against Shigella flexneri compared to ampicillin and cefadroxil. Thus, the hydroxy group on ring D carrying a compounds 5aj and 5bj have shown excellent (MIC: 0.34 mg ml−1) to good (MIC: 1.78 mg ml−1) activity against S. aureus. Against B. subtilis also, the methoxy group carrying compounds 5af and 5bf have shown (MIC: 3.10–3.75 mg ml−1) antibacterial activity to the standard drugs. Against E. coli, compounds 5af, 5ag, 5aj, 5ak, 5bg and 5bj have shown excellent activity compared to ampicillin and cefadroxil depending on the methoxy and hydroxyl group. Against Shigella flexneri, all compounds have shown low activity compared to the standard drugs without depending on functional groups on aromatic ring except 5aj and 5bj.
3. Conclusions
In conclusion, we have developed a convenient and simple method for the preparation of novel bioactive benzoxazinone–isoxazole-clubbed chalcone derivatives via the reaction of new ketone derivatives with substituted aldehydes in the presence of piperidine. The rapid conversion, excellent yield, and operational simplicity are great advantages of the proposed method. Furthermore, the reaction conditions employed include microwave irradiation as a source of energy, in short reaction time, contemplating the principles of green chemistry. Compounds 5aj, 5ak and 5bj exhibited the highest biological activity toward Gram-positive S. aureus and Gram-negative E. coli bacteria with MIC: 0.34, 0.59 and 0.74 mg ml−1 respectively as compare to standard drug.
4. Experimental
All the required chemicals were purchased from Merck and Aldrich Chemical Company. Pre-coated aluminium sheets (silica gel 60 F254, Merck) were used for thin-layer chromatography (TLC) and spots were visualized under UV light. IR spectra were recorded with KBr on Thermo Nicolet FT-IR spectrophotometer. 1H NMR and 13C NMR spectra were recorded respectively on Bruker Spectrospin DPX 500 MHz and Bruker Spectrospin DPX 125 MHz spectrometer using CDCl3 as a solvent and trimethylsilane (TMS) as an internal standard. Splitting patterns are designated as follows; s = singlet, d = doublet, m = multiplet, br = broad. Chemical shift (δ) values are given in ppm. High-resolution mass spectra (HRMS) were obtained on a Brüker micrOTOF™-Q II mass spectrometer (ESIMS).
4.1. Microwave irradiation experiment
All microwave experiments were carried out in a dedicated Anton Paar Monowave-300 reactor®, operating at a frequency of 2.455 GHz with continuous irradiation power of 0 to 850 W. The reactions were performed in a G-10 borosilicate glass vial sealed with Teflon septum and placed in a microwave cavity. Initially, microwave of required power was used and temperature was being ramped from room temperature to a desired temperature. Once this temperature was attained, the process vial was held at this temperature for required time. The reactions were continuously stirred. Temperature was measured by an IR sensor. After the experiments a cooling jet cooled the reaction vessel to ambient temperature.
4.2. General procedure for synthesis of compounds
4.2.1. Preparation of compound 5a and 5b. A mixture of 6-bromo-4-methyl-4-vinyl-1H-benzo[d][1,3]oxazin-2(4H)-one (4a) or 4-allyl-6-bromo-4-methyl-1H-benzo[d][1,3]oxazin-2(4H)-one (4b) (5 mmol) and ammonium cerium(IV) nitrate (2.74 g, 5 mmol) in acetone (20 ml) was stirred under reflux for 12 h. The reaction mixture was extracted with EtOAc (50 ml) and washed with aq. NaHCO3 solution (2 × 30 ml), saturated aq. NaCl (2 × 30 ml), and water (2 × 30 ml). The ethereal solution was dried over Na2SO4 and concentrated in a vacuum. The resulting solid was chromatographed on silica gel. Elution with hexane–EtOAc (8
:
2) gave 4-(3-acetyl-4,5-dihydroisoxazol-5-yl)-6-bromo-4-methyl-1H-benzo[d][1,3]oxazin-2(4H)-one (5a) and 4-((3-acetyl-4,5-dihydroisoxazol-5-yl)methyl)-6-bromo-4-methyl-1H-benzo[d][1,3]oxazin-2(4H)-one (5b) as white solid (70%).
4.2.2. Preparation of chalcones 5aa–ak and 5ba–bj. Dissolve 1 mmol of the substituted aldehyde and 1 mmol of the 5a or 5b in 10 ml of ethanol in a G-10 process vial capped with Teflon septum. 2 mmol of piperidine then added to the reaction vial using a micropipette. After a pre-stirring for one minute, the vial was subjected to microwave irradiation with the initial ramp time of 1 minute at 70 °C. The temperature was then raised to 80 °C with the holding time of 10–16 min. After completion of the reaction, cool the mixture in an ice-water bath until crystal formation is complete. Add 10 ml of ice-cold water to the flask and vacuum filter. Wash the crystals with water followed by ice-cold ethanol. Allow to air-dry. Recrystallize from 95% ethanol if necessary to afford the pure chalcones 5aa–ak and 5ba–bj in 81–93% yield.
4.3. Characterization data
4.3.1. (E)-6-Bromo-4-(3-(3-(4-fluorophenyl)acryloyl)-4,5-dihydroisoxazol-5-yl)-4-methyl-1H-benzo[d][1,3]oxazin-2(4H)-one (5aa). Yield: 0.42 g (92%) as yellow solid; mp 212–214 °C; 1H NMR (500 MHz, CDCl3, ppm): δ 1.78 (s, 3H), 2.87–2.94 (m, 1H), 3.0–3.06 (m, 1H), 3.89–3.97 (m, 1H), 6.78 (d, J = 14.0 Hz, 1H), 7.24 (s, 1H), 7.34 (d, J = 9.5 Hz, 1H), 7.40 (d, J = 9.5 Hz, 2H), 7.48 (d, J = 6.5 Hz, 2H), 7.56 (d, J = 8.5 Hz, 1H), 7.94 (d, J = 14.0 Hz, 1H), 9.67 (s, br, D2O exchangeable, 1H); 13C NMR (125 MHz, CDCl3, ppm): δ 22.1, 26.7, 84.9, 86.1, 117.0, 120.9, 126.9, 127.5, 129.1, 130.3, 130.9, 131.5, 132.4, 134.0, 138.0, 138.3, 138.8, 153.3, 189.3; FTIR (KBr, v = cm−1): 1638, 1716, 3331; HRMS (ESI+): m/z calcd. for C21H16BrFN2NaO4 [M + Na]+: 481.0175 found: 481.0171.
4.4. Biological activity
4.4.1. Antibacterial assay. Minimum inhibitory concentration (MIC) is the lowest concentration of an antimicrobial compound that inhibits the visible growth of a microorganism. MIC values of the compounds against bacterial isolates were determined on the basis of the micro-well dilution method following National Committee for Clinical Laboratory Standards (NCCLS) recommendations.14 In this method we made stock of chemically synthesized compounds at a concentration of 10 mg ml−1 in DMSO, which was further converted to working solution of concentration 1 mg ml−1. Using a micropipette, 100 μl of media was dispensed into all wells of a pre-sterilized micro titre plate (experiment was done in triplicate). Two fold serial dilutions (100, 50, 25, 0.78125 mg ml−1) were carried out from the well #1 to the well #10 and excess media (100 μl) was discarded from the last well (#10). A liquid broth culture of the test organism was grown to log phase in Luria Bertani broth (LB broth) for 24 h at 37 °C. The optical density of the liquid culture was determined at 600 nm and diluted in such a way that each well received 107 cfu ml−1 of bacterial culture. Appropriate positive and negative controls were also included in the study. The positive control contained only microbial cells whereas the negative control contained only standard drug solution (ampicillin and cefadroxil). All experimental procedures were performed under sterile conditions using bio-safety hood and microtitre plates were incubated at 37 °C for 24 h.
Acknowledgements
The authors wish to express their gratitude to DST New Delhi for providing HRMS, NMR facility and CSIR, New Delhi for awarding SRF to N. V. and S. K.
Notes and references
-
(a) C. Q. Meng, L. Ni, K. J. Worsencroft, Z. Ye, M. D. Weingarten, J. E. Simpson, J. W. Skudlarek, E. M. Marino, K.-L. Suen, C. Kunsch, A. Souder, R. B. Howard, C. L. Sundell, M. A. Wasserman and J. A. Sikorski, J. Med. Chem., 2007, 50, 1304 CrossRef CAS PubMed;
(b) J. S. Biradar, B. S. Sasidhar and R. Parveen, J. Med. Chem., 2010, 45, 4074 CrossRef CAS PubMed;
(c) M. V. Reddy, T. L. Hwang, Y. L. Leu, W. F. Chiou and T. S. Wu, Bioorg. Med. Chem., 2011, 15, 2751 CrossRef PubMed;
(d) S. R. Cherkupally, C. R. Dassari, Y. Vookanti and N. Adki, Org. Commun., 2010, 3, 57 CAS;
(e) A. Azam, F. Hayat, E. Mosely, S. Attar and R. Vanzyl, Eur. J. Med. Chem., 2011, 46, 1897 CrossRef PubMed;
(f) B. Srinivasan, T. E. Johnson, R. Lad and C. Xing, J. Med. Chem., 2009, 52, 7228 CrossRef CAS PubMed;
(g) L.-M. Linn, Y. Zhou, M. T. Flavin, L. M. Zhou, W. Nie and F.-C. Chen, Bioorg. Med. Chem., 2002, 10, 2795 CrossRef;
(h) M. Satyanarayana, P. Tiwari, B. K. Tripathi, A. K. Srivastava and R. Pratap, Bioorg. Med. Chem., 2004, 12, 883 CrossRef CAS PubMed;
(i) P.-L. Zhao, C.-L. Liu, W. Huang, Y.-Z. Wang, G.-F. Yang and J. Agric, Food Chem., 2007, 55, 5697 CrossRef CAS PubMed;
(j) N. Aoki, M. Muko, E. Ohta and S. Ohta, J. Nat. Prod., 2008, 71, 1308 CrossRef CAS PubMed;
(k) M. Ono, R. Watanabe, H. Kawashima, Y. Cheng, H. Kimura, H. Watanabe, M. Haratake, H. Saji and M. Nakayama, J. Med. Chem., 2009, 52, 6394 CrossRef CAS PubMed.
-
(a) A. Barbary, A. I. Khodair, E. B. Pederson and C. Nielsen, J. Med. Chem., 1994, 37, 73 CrossRef PubMed;
(b) H. I. Subbagh, S. M. Abu-Zaid, M. A. Mahran, F. A. Badaria and A. M. Al-Obaid, J. Med. Chem., 2000, 43, 2915 CrossRef PubMed;
(c) J. R. Dimmock, S. K. Raghavan, B. M. Logan and G. E. Bigam, Eur. J. Med. Chem., 1983, 18, 248 CAS.
-
(a) G. Amgad, P. N. Habeeb, R. Praveen and E. E. Knaus, J. Med. Chem., 2001, 44, 2921 CrossRef;
(b) C.-M. Sun, L.-G. Lin, H.-J. Yu, C.-Y. Cheng, Y.-C. Tsai, C.-W. Chu, Y.-H. Din, Y.-P. Chau and M.-J. Don, Bioorg. Med. Chem. Lett., 2007, 17, 1078 CrossRef CAS PubMed;
(c) G. Daidone, D. Raffa, B. Maggio, F. Plescia, V. M. C. Cutuli, N. G. Mangano and A. Caruso, Arch. Pharm., 1999, 50, 332 Search PubMed;
(d) D. Binder, F. Rovenszky and H. P. Ferber, US Pat., 4841065, 1985. Chem. Abstr., 1985, 103, 196028;
(e) A. H. Bhatt, H. H. Parekh and A. R. Parikh, Heterocycl. Commun., 1998, 4, 361 CrossRef CAS;
(f) J. F. Hansen and S. A. Stronge, J. Heterocycl.
Chem., 1977, 14, 1289 CAS;
(g) S. Balalaie, A. Sharifi and B. Ahangarian, Indian J. Heterocycl. Chem., 2000, 10, 149 CAS;
(h) M. Eda, T. Kuroda, S. Kaneko, Y. Aoki, M. Yamashita, C. Okumura, Y. Ikeda, T. Ohbora, M. Sakaue, N. Koyama and K. Aritomo, J. Med. Chem., 2015, 58, 4918 CrossRef CAS PubMed;
(i) M. Rowley, H. B. Broughton, I. Collins, R. Baker, F. Emms, R. Marwood, S. Patel, C. I. Ragan, S. B. Freedman and P. D. Leeson, J. Med. Chem., 1996, 39, 1943 CrossRef CAS PubMed;
(j) B. Frolund, A. T. Jorgensen, L. Tagmose, T. B. Stensbol, H. T. Vestergaard, C. Engblom, U. Kristiansen, C. Sanchez, P. Krogsgaard-Larsen and T. Liljefors, J. Med. Chem., 2002, 45, 2454 CrossRef CAS PubMed;
(k) M. P. Giovannoni, C. Vergelli, C. Ghelardini, N. Galeotti, A. Bartolini and V. Dal Piaz, J. Med. Chem., 2003, 46, 1055 CrossRef CAS PubMed.
- O. Wahlroos and A. I. Virtanen, Acta Chem. Scand., 1959, 13, 1906 CrossRef CAS.
-
(a) J. Ilas, P. S. Anderluh, M. S. Dolenc and D. Kikelj, Tetrahedron, 2005, 61, 7325 CrossRef CAS;
(b) F. A. Macıas, D. Marın, A. Oliveros-Bastidas and J. M. G. Molinillo, Nat. Prod. Rep., 2009, 26, 478 RSC;
(c) W. L. F. Armarego, Adv. Heterocycl. Chem., 1979, 24, 17 CrossRef;
(d) I. R. Ager, D. R. Harrison, P. D. Kennewel and J. B. Taylor, J. Med. Chem., 1977, 20, 379 CrossRef CAS PubMed;
(e) W. L. F. Armarego, Adv. Heterocycl. Chem., 1963, 1, 304 CrossRef;
(f) P. Wikder and A. Wilson, J. Am. Chem. Soc., 1955, 77, 5598 CrossRef.
- J. Poupaert, P. Carato, E. Colacino and S. Yous, Curr. Med. Chem., 2005, 12, 877 CrossRef CAS PubMed.
- V. Tiwari, P. Ali and J. Meshram, Green Chem. Lett. Rev., 2011, 4, 219 CrossRef CAS.
- P. Kumar, S. Kumar, K. Husain and A. Kumar, Chin. Chem. Lett., 2011, 22, 37 CrossRef CAS.
- Z. N. Siddiqui, T. N. M. Musthafa, A. Ahmad and A. U. Khan, Bioorg. Med. Chem. Lett., 2011, 21, 2860 CrossRef CAS PubMed.
-
(a) S. Kumar, N. Verma, I. Parveen and N. Ahmed, J. Heterocycl. Chem., 2015 DOI:10.1002/jhet.2517;
(b) S. Kumar and N. Ahmed, Green Chem., 2016, 18, 648 RSC;
(c) S. Kumar and N. Ahmed, RSC Adv., 2015, 5, 77075 RSC;
(d) S. Kumar, N. Verma and N. Ahmed, RSC Adv., 2015, 5, 85128 RSC;
(e) S. Kumar, N. Konduru, N. Verma and N. Ahmed, Synth. Commun., 2015, 45, 2555 CrossRef CAS;
(f) S. Kumar, N. Verma, N. Kumar, A. Patel, P. Roy, V. Pruthi and N. Ahmed, Synth. Commun., 2016, 46, 460 CrossRef CAS;
(g) S. Kumar, A. Patel and N. Ahmed, RSC Adv., 2015, 5, 93067 RSC;
(h) S. Kumar, N. Verma and N. Ahmed, J. Saudi Chem. Soc., 2016 DOI:10.1016/j.jscs.2016.01.005.
- Z. Huang, Y. Yang, Q. Xiao, Y. Zhang and J. Wang, Eur. J. Org. Chem., 2012, 33, 6586 Search PubMed.
-
(a) P. Zhang, P. Zhang, E. A. Terefenko, A. Fensome, J. Wrobel, R. Winneker, S. Lundeen, K. B. Marschke and Z. Zhang, J. Med. Chem., 2002, 45, 4379 CrossRef CAS PubMed;
(b) H.-B. Zhou, J. H. Lee, C. G. Mayne, K. E. Carlson and J. A. Katzenellenbogen, J. Med. Chem., 2010, 53, 3349–3360 CrossRef CAS PubMed.
- K. Itoh and C. A. Horiuchi, Tetrahedron, 2004, 60, 1671 CrossRef CAS.
- J. R. Zgoda and J. R. Porter, Pharm. Biol., 2001, 39, 221 CrossRef CAS.
Footnote |
† Electronic supplementary information (ESI) available. See DOI: 10.1039/c6ra08710h |
|
This journal is © The Royal Society of Chemistry 2016 |