DOI:
10.1039/C6RA07086H
(Paper)
RSC Adv., 2016,
6, 54346-54356
Purification, characterization and synthesis of antioxidant peptides from enzymatic hydrolysates of coconut (Cocos nucifera L.) cake protein isolates
Received
18th March 2016
, Accepted 27th May 2016
First published on 27th May 2016
Abstract
Papain was optimized to hydrolyze coconut cake protein isolates (CCPI) to produce peptides with high antioxidant activity. From the response surface methodology generated model, the optimum conditions were using papain at a concentration of 0.97 g/100 g CCPI, a hydrolysis time of 6.45 h, a temperature of 49.09 °C and a pH of 6.68. The coconut cake protein isolates hydrolysates were separated by G-25 gel chromatography and five fractions were obtained. The amino acid composition and antioxidant activity of the fractions were investigated. Fraction E with the highest antioxidant activity was further purified using gel filtration chromatography, ion exchange chromatography and RP-HPLC (reversed-phase high performance liquid chromatography). Finally, two peptides Pro-Gln-Phe-Tyr-Trp (865.02 Da) and Arg-Pro-Glu-Ile-Val (612.36 Da) were identified. Their IC50 (the concentration of peptide that is required to scavenge 50% of radical activity) on hydroxyl radical scavenging activities were 4.28 and 7.65 μg mL−1. Furthermore, these two peptides were chemically synthesized and the synthetic peptides showed good stability against simulated gastrointestinal protease digestion.
1. Introduction
Free radicals are accepted as important mediators of tissue injury in several neurodegenerative diseases and other pathological conditions.1,2 Oxidation is a major cause of food quality changes affecting the nutritional, texture and appearance and leads to undesirable off-flavors and potentially toxic reaction products.3 Hence, it is important to inhibit oxidation reactions and the formation of free radicals occurring in living bodies. Antioxidant peptides derived from food proteins have been considered as ideal natural antioxidants in food industry or medical treatment.4 Now, some antioxidant peptides have been identified from varieties of food proteins such as royal jelly protein, oil palm kernel protein and rice bran protein.5–7 It was reported that antioxidant peptides relied on their specific amino acid sequences and peptides with specific amino acid sequences could be obtained by enzymatic hydrolysis from protein source.3
Coconut cake, a byproduct of the coconut oil industry, contains about 10% to 16% of protein.8 Coconut cake protein (CCP) is a good source of edible protein for its abundant of essential amino acids and high emulsifying properties.9 Moreover, Mini and Rajamohan reported that CCP could prevent hyperlipidemia and lower serum cholesterol.10 Furthermore, Wang and Ng11 had isolated an antifungal peptide from coconut flesh, and three antimicrobial peptides (Cn-AMP1, Cn-AMP2 and Cn-AMP3) were identified from green coconut water.12,13 However, there is a little information regarding the antioxidant effect of peptides from CCP as well as their preparation, purification and bioactivity. Every year, about 200 million tons of coconut cake is produced in the world, meaning that a large amount of protein could be produced.14 If peptides with high antioxidant activity could be obtained from it, the usage of coconut cake will be expanded and remarkable economic benefits may be achieved.
Therefore, the objects of this paper are: (1) to optimum the enzymatic conditions to hydrolyze CCPI and (2) to separate, sequence, synthesize and characterize the most potential antioxidant peptides isolated from the coconut cake isolate hydrolysates (CCPIH).
2. Materials and methods
2.1 Materials and reagents
Coconut cake was obtained from the Coconut View Garden, Wenchang, China. Acid protease (5 × 104 U g−1), neutrase (1 × 105 U g−1), flavourzyme (5 × 104 U g−1), papain (5 × 104 U g−1) and alcalase (1 × 105 U g−1) were purchased from Shanghai Biotech. Co., Ltd. (China). Sephadex G-25, SP Sephadex C-25 and Sephadex G-15 were purchased from Pharmacia (Uppsala, Sweden). Acetonitrile (HPLC grade) was purchased from TianGen Co. Ltd. (American). Other chemicals and reagents were of analytical grade.
2.2 Preparation of coconut cake protein isolates (CCPI)
Followed the modified method of Angelia et al.,9 coconut cake was defatted three times with n-hexane (1
:
10, g mL−1) and dried, ground and passed through a sieve of 0.2 mm mesh. Thirty grams of defatted coconut cake powder were suspended in 300 mL of phosphate buffer (0.1 M, pH 8.0, containing 0.4 M NaCl). After being stirred at 4 °C for 1 h, the mixture was filtered and centrifuged at 10
000 × g for 30 min. The supernatant was collected and precipitated by adjusting to pH 4.5 and then centrifuged again. The precipitate was collected, dialyzed, lyophilized and stored at −20 °C.
2.3 Optimization of the enzymatic hydrolysis conditions
2.3.1 Choose of enzymes. Five g CCPI were suspended in 100 mL of phosphate buffer (0.1 M). Acid protease, neutrase, flavourzyme, alcalase, papain, mixture of flavourzyme and alcalase were separately used to hydrolyze CCPI at the suitable conditions recommended by their producers (Fig. 1). After hydrolysis, the mixture was incubated at 100 °C for 5 min and centrifuged at 12
000 × g for 15 min. The supernatant was collected and lyophilized. Degree of hydrolysis (DH) and scavenging activity on hydroxyl radical (·OH) were determined by the method of Adler-Nissen15 and the 2-deoxyribose oxidation method,16 respectively.
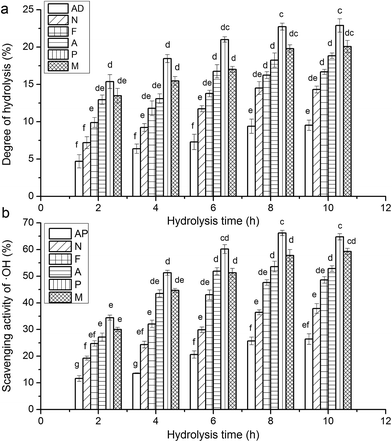 |
| Fig. 1 The degree of hydrolysis (a) and scavenging activity (b) of the hydrolysates from different enzymes treatments. AP was hydrolyzed with acid protease at pH2.0, E/S (enzyme to substrate) = 1%, 45 °C; N, hydrolyzed by neutrase at pH 7.0, E/S = 1%, 50 °C; F, hydrolyzed with flavourzyme at pH 6.5, E/S = 0.8%, 53 °C; A, hydrolyzed with alcalase at pH 8.5, E/S = 0.9%, 55 °C; P, hydrolyzed with papain at pH 7.0, E/S = 0.75%, 55 °C; M, hydrolyzed with alcalase at its optimal conditions followed by flavourzyme at its optimum conditions; c–g, different small letters on the bar meant significant difference (P < 0.05). | |
2.3.2 Experiment design. A central composite rotatable design (CCRD) with four variables including E/S (ratio of enzyme to substrate), time, pH and temperature, as well as utilizing five levels was used to investigate the optimal hydrolysis conditions of papain. The quadratic effects and central points were estimated with the DH as response. The response pattern and the optimum combination of variables of hydrolysis assay were determined. The independent variables Xi were coded as xi, which was defined as dimensionless, according to the following equation:where, xi was the coded value of an independent variable, Xi was the real value of an independent variable, X0 was the real value of an independent variable at the centre point and ΔXi was the step change value. The selected variables and levels were listed in Table 1.
Table 1 Experimental values and coded levels of the independent variables for central composite rotatable design (CCRD)
Independent variable |
Symbols |
Levels |
Coded |
Uncoded |
−2 |
−1 |
0 |
+1 |
+2 |
Temperature (°C) |
x1 |
X1 |
30 |
40 |
50 |
60 |
70 |
pH |
x2 |
X2 |
5 |
6 |
7 |
8 |
9 |
Enzyme to substrate (%, w/w) |
x3 |
X3 |
0.25 |
0.5 |
0.75 |
1.0 |
1.25 |
Time (h) |
x4 |
X4 |
2 |
4 |
6 |
8 |
10 |
2.3.3 Statistical analyses and verification of the model. The response obtained was subjected to multiple non-linear regression analysis to gain the coefficients. The DH of CCPI can be expressed as function of independent variables by the second-order polynomial as follows: |
Y = b0 + ∑bjxj + ∑bjjxj2 + ∑bjkxjxk
| (2) |
where, Y was the response (DH, %), b0 was the intercept, bj, bjj, bjk were linear, quadratic and interactive coefficients, respectively. The responses obtained were statistically evaluated and the model was built based on the variables with confidence levels more than 95%. All analyses were done by Design Expert software (version 7.0, Stat-Easy Inc., Minneapolis, USA). The optimum conditions of enzymatic hydrolysis with papain depended on temperature, pH, E/S and time were obtained from the CCRD generated model. Then CCPI was hydrolyzed by papain under the optimal conditions to verify the model, the hydrolysates (CCPIH) were collected, lyophilized and stored at −20 °C.
2.4 Separation of CCPIH by Sephadex G-25 gel chromatography
CCPIH prepared with papain was separated by a Sephadex G-25 gel filtration column (Φ1.6 cm × 100 cm) equilibrated with distilled water at 0.8 mL min−1. The column was eluted with distilled water and monitored at 254 nm. Fractions were collected, lyophilized and stored at −20 °C.
2.5 Amino acid composition and antioxidant activity of CCPIH fractions
2.5.1 Amino acid analysis. Amino acid composition of CCPIH fractions was determined according to the method of Wang et al.17 The obtained data was used for calculating Bigelow parameters, including NPS (the frequency of nonpolar side chains) and P (ratio of polar to nonpolar side chains). Thus, NPS was calculated by counting the Trp, Ile, Tyr, Phe, Pro, Leu and Val residues and expressing the sum as a fraction of the total number of residues.18
2.5.2 Scavenging activity of hydroxyl radical. Hydroxyl radical scavenging activity was assayed using the 2-deoxyribose oxidation method.16 IC50 was defined as the concentration of peptide that was required to scavenge 50% of radical activity. The activity was determined as follows: |
·OH scavenging activity (%) = [1 − (AS − AB)/AC] × 100
| (3) |
where AB is the absorbance of the blank (distilled water instead of samples), AC is the absorbance of control (without the addition of 2-deoxyribose oxidation) and AS is the absorbance of mixture contained samples.
2.5.3 Superoxide radical-scavenging activity. According to the method of Marklund and Marklund,19 0.1 mL of the fractions and 3 mL of pyrogallol solution (3 mM) were mixed. The absorbance of the mixture at 320 nm was recorded at 30 s intervals using a spectrophotometer. The antioxidant activity was determined as the percentage of inhibiting pyrogallol autoxidation, which was calculated from the absorbance at 320 nm in the presence or absence of samples.
2.5.4 ABTS radical scavenging ability. Two mL of ABTS (2,2′-azino-bis-(3-ethylbenzothiazoline-6-sulphonic acid)) radical solution and 20 μL of sample solutions (50–250 μg mL−1) were reacted at 30 °C for 6 min. Then the absorbance at 734 nm was recorded.20 The activity was calculated as follows: |
Scavenging activity (%) = [1 − (AS − AB)/AC] × 100
| (4) |
where AB is the absorbance of the blank, AC is the absorbance of control (distilled water instead of ABTS+ solution) and AS is the absorbance of mixture contained samples.
2.5.5 Metal chelating capacity. According to the method of Kong and Xiong,21 450 μL of sample solutions (50–250 μg mL−1), 45 μL of 2 mM FeCl2 and 1815 μL of distilled water were mixed. The mixture was reacted with 90 μL of 5 mM ferrozine for 30 min. Then the absorbance was read at 562 nm. Chelating activity was calculated as follows: |
Chelating activity (%) = [1 − (AS − AB)/AC] × 100
| (5) |
where AB is the absorbance of the blank, AC is the absorbance of control (without the addition of ferrozine) and AS is the absorbance of mixture contained samples.
2.5.6 Reducing power. Reducing power was determined by the method of Benzie and Strain.22 Concentrations of sample solutions were 50 to 250 μg mL−1. Increased absorbance at 700 nm of the reaction mixture indicated increased reducing power.
2.5.7 Inhibition of linoleic acid autoxidation. Following the modified method of Wu et al.,23 aliquot of sample solutions (100 μg mL−1) was added into 1 mL of linoleic acid (50 mM) in a tube sealed tightly and kept at 60 °C in the dark for 8 days. The degree of oxidation was evaluated by measuring the ferric thiocyanate values.24 Propyl gallate (PG, 100 μg mL−1) was used as comparison.
2.5.8 Protection on DNA damage. According to the modified method of Jeong et al.,25 5 μL of 2 mg mL−1 samples were mixed with 2 μL of 6 mM H2O2, 3 μL of 1.5 mM FeSO4 and 1 μL of plasmid pBR322 DNA, incubated at 37 °C for 1 h. Then electrophoresis was performed in 1% agarose gel. The remaining super-coiled (SC) form against oxidative DNA cleavage was quantified using a gel imaging system (Synene BOXF3, UK).
2.6 Purification of the antioxidant peptides
Following the method of Zhang et al.5 with some modifications, the CCPIH fraction from Sephadex G-25 chromatography showing the highest antioxidant activity was loaded onto a cationic exchange column (Φ2.6 cm × 60 cm) with Sephadex C-25 equilibrated with 20 mM sodium acetate buffer (pH 5.8), eluted with a linear gradient of NaCl (0–1.0 M) in the same buffer at a flow rate of 0.8 mL min−1 and monitored at 220 nm. The fraction with the highest antioxidant activity was concentrated and loaded on Sephadex G-15, eluted with distilled water at flow rate of 0.6 mL min−1 and monitored at 220 nm. The fraction exhibiting the highest antioxidant activity was separated by reversed-phase high performance liquid chromatography (RP-HPLC) on a Zorbax semi-preparative C18 column (Φ9.4 mm × 250 mm, Agilent Technologies, USA), using a linear gradient of acetonitrile containing 0.1% TFA (5–30%, in 30 min) at a flow rate of 2.5 mL min−1. The fraction showing high antioxidant activity was further isolated on a Zorbax analysis C18 column (Φ4.6 mm × 250 mm, Agilent Technologies, USA) with a linear gradient of acetonitrile containing 0.1% TFA (5–25%, in 20 min) at a flow rate of 1.0 mL min−1. The fractions with high antioxidant activity were rechromatographed to confirm their purity on the same analytical C18 column at a flow rate of 1.0 mL min−1 with a linear gradient of acetonitrile containing 0.1% TFA (5–25%, in 20 min).
2.7 Molecular mass and amino acid sequence of the purified peptides
Molecular mass and amino acid sequence of the purified peptides were determined with a Q-TOF mass spectrometer (Micromass, Altrincham, UK) coupled with electrospray ionization (ESI) source. Ionization was carried out in positive mode with a capillary voltage of 3500 V. Nitrogen was maintained at 40 psi for nebulization and 9 L min−1 at 350 °C for evaporation temperature. Data were collected in centroid mode from m/z 100 to 2000. Peaks Viewer 4.5 (Bioinformatics Solutions Inc., Waterloo, ON, Canada) was used in combination with manual de novo sequencing to process the MS/MS data and to perform peptide sequence.
2.8 Peptide synthesis
Antioxidant peptides identified from CCPIH were synthesized by the solid phase procedure with a Liberty microwave peptide synthesizer (Mathews, NC, USA). Briefly, the peptides were analyzed with a Kromasil 100-5 C18 column (4.6 × 250 mm; particle size 5 μm) eluted with mobile phase consisting of 0.1% TFA in water and 0.1% TFA in acetonitrile. The purity and molecular mass of the synthetic peptides were determined by Liquid Chromatography coupled to Mass Spectrometry (LC-MS/ESI).
2.9 Stability of the synthetic peptides
Following the method of Tavares et al.,26 peptides solutions were adjusted to pH 2.0 and mixture with pepsin (E/S = 1
:
35), incubated at 37 °C for 1 h. Afterwards, the reaction mixture was adjusted to pH 7.0, pancreatin was added (1 g/25 g peptide). After incubated at 37 °C for 2 h, the digestion was terminated after boiling for 10 min. The ·OH scavenging of the treated synthetic peptides and the untreated synthetic peptides were determined, respectively.
2.10 Statistical analysis
All the results were the means of triplicates. Data were subjected to analysis of variance and Duncan value with a confidence interval of 95% was calculated to compare means.
3. Results and discussion
3.1 Optimization of hydrolysis conditions for papain
As shown in Fig. 1, the hydrolysates with higher DH exhibited higher ·OH scavenging activity. The CCPIH with papain showed the highest DH and ·OH scavenging activity. It was pointed out that DH could influence the antioxidant activities of peptides through affecting the peptides' length as well as the terminal amino groups.27 Peptides with high antioxidant activity from loach protein and rice bran protein were also obtained using papain.7,28
The coded values of the CCRD test variables and experimental results of DH were shown in Table 2. Multiple regression analysis of the experimental data deduced the following regression equation for the DH (Y, %) of CCPI.
|
Y = 21.02 + 0.56x1 − 0.06x2 + 1.15x3 + 0.73x4 + 0.51x1x2 − 1.01x1x3 + x1x4 − 0.53x2x3 + 0.06x2x4 − 0.07x3x4 − 2.15x12 − 0.88x22 − 0.78x32 − 1.34x42
| (6) |
Table 2 Central composite rotatable design (CCRD) and response
Experiment |
Independent variables |
DH (%) |
X1 |
X2 |
X3 |
X4 |
1 |
−1 |
−1 |
−1 |
−1 |
12.00 |
2 |
1 |
1 |
1 |
−1 |
14.97 |
3 |
−2 |
0 |
0 |
0 |
10.16 |
4 |
1 |
−1 |
−1 |
1 |
16.16 |
5 |
1 |
1 |
−1 |
−1 |
15.08 |
6 |
−1 |
1 |
1 |
1 |
16.79 |
7 |
0 |
0 |
0 |
0 |
20.00 |
8 |
−1 |
1 |
−1 |
1 |
13.04 |
9 |
0 |
0 |
0 |
−2 |
14.00 |
10 |
0 |
0 |
0 |
2 |
18.98 |
11 |
−1 |
1 |
1 |
−1 |
15.74 |
12 |
0 |
2 |
0 |
0 |
17.50 |
13 |
1 |
−1 |
−1 |
−1 |
12.98 |
14 |
0 |
0 |
0 |
0 |
20.51 |
15 |
1 |
−1 |
1 |
1 |
15.39 |
16 |
−1 |
−1 |
−1 |
1 |
12.82 |
17 |
0 |
0 |
2 |
0 |
20.36 |
18 |
−1 |
−1 |
1 |
−1 |
19.64 |
19 |
0 |
−2 |
0 |
0 |
19.10 |
20 |
0 |
0 |
0 |
0 |
22.20 |
21 |
1 |
1 |
1 |
1 |
17.87 |
22 |
0 |
0 |
−2 |
0 |
17.06 |
23 |
2 |
0 |
0 |
0 |
16.31 |
24 |
0 |
0 |
0 |
0 |
22.61 |
25 |
0 |
0 |
0 |
0 |
20.70 |
26 |
−1 |
−1 |
1 |
1 |
18.65 |
27 |
1 |
1 |
−1 |
1 |
16.67 |
28 |
−1 |
1 |
−1 |
−1 |
14.30 |
29 |
0 |
0 |
0 |
0 |
20.11 |
30 |
1 |
−1 |
1 |
−1 |
15.11 |
Fig. 2 indicated a good fit of the plot of experimental values of DH versus those calculated from eqn (6). For a good fit model, R2 should be at least 0.80.29 Results of analysis of variance (ANOVA) for the CCRD shown in Table 3 demonstrated that it was a significant regression model with a reasonable R2 (0.8603) and insignificant lack of fit value (0.1365). The probability (P) value of the regression model significant was less than 0.001, meaning that the developed model could adequately represent the true relationship among the parameters chosen.
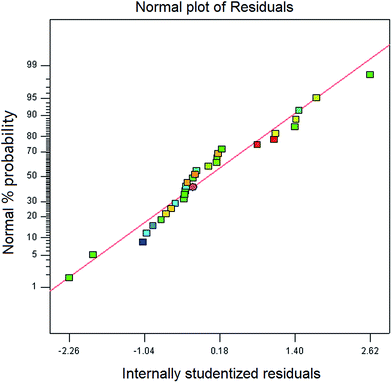 |
| Fig. 2 Correlation of calculated DH of CCPI with experimental DH of CCPI. | |
Table 3 Analysis of variance (ANOVA) for response surface quadratic model for the enzymatic hydrolysis of coconut cake protein isolateb
Source |
Coefficient estimate |
Sum of squares |
DF |
F value |
p-Value Prob > F |
Significant variables. Standard deviation, 1.64; R2, 0.86; mean, 16.89; adjusted R2, 0.73; C.V.%, 9.69; predicted R2, 0.29; press, 204.79; adequate precision, 8.42. |
Model |
|
247.29 |
14 |
6.60 |
0.0004a |
Intercept |
21.02 |
|
|
|
|
x1-Temperature (°C) |
0.56 |
7.64 |
1 |
2.85 |
0.1119 |
x2-pH |
−0.06 |
0.09 |
1 |
0.03 |
0.8583 |
x3-[E]/[S] (%) |
1.15 |
32.02 |
1 |
11.96 |
0.0035a |
x4-Time/h |
0.73 |
12.80 |
1 |
4.78 |
0.0450a |
x1x2 |
0.51 |
4.18 |
1 |
1.56 |
0.2304 |
x1x3 |
−1.01 |
16.43 |
1 |
6.14 |
0.0256a |
x1x4 |
0.52 |
4.32 |
1 |
1.61 |
0.2231 |
x2x3 |
−0.53 |
4.57 |
1 |
1.71 |
0.2110 |
x2x4 |
0.06 |
0.06 |
1 |
0.02 |
0.8850 |
x3x4 |
−0.07 |
0.07 |
1 |
0.03 |
0.8702 |
x12 |
−2.15 |
127.11 |
1 |
47.47 |
<0.0001a |
x22 |
−0.89 |
21.55 |
1 |
8.05 |
0.0125a |
x32 |
−0.78 |
16.81 |
1 |
6.28 |
0.0242a |
x42 |
−1.34 |
49.06 |
1 |
18.32 |
0.0007a |
Residual |
|
40.16 |
15 |
|
|
Lack of fit |
|
34.016 |
10 |
2.77 |
0.1365 |
Pure error |
|
6.15 |
5 |
|
|
Cor total |
|
287.46 |
29 |
|
|
Moreover, x3 (E/S), x4 (hydrolysis time), x1x3, x12, x22, x32 and x42 were significant model terms because their values of “Prob > F” were all less than 0.05 (Table 3). The response surfaces and contour plots of enzymatic hydrolysis conditions were shown in Fig. 3a and b. The E/S seemed to play a linear effect on the response whereas the effect of temperature or pH on DH was insignificant (P > 0.05). The optimum enzymatic hydrolysis conditions predicted by the models were temperature 49.09 °C, pH 6.68, time 6.45 h and E/S 0.97 (g/100 g CCPI). The DH of the verified experiments was 20.96% ± 0.52, comparable with the predicted value (21.61%). Therefore, the model was adequate.
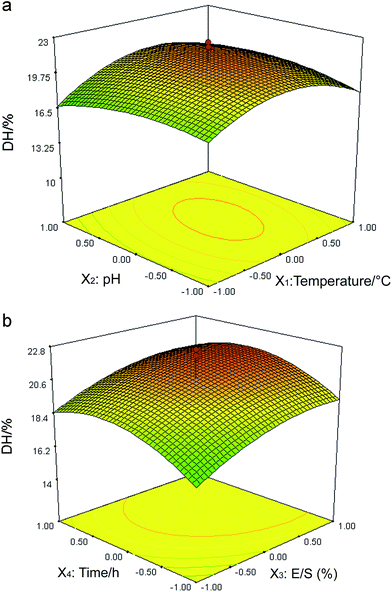 |
| Fig. 3 Surface plots for DH of CCPI. (a) Effect of temperature and pH on DH of CCPI when E/S was 5% and hydrolysis time 6 h. (b) Effect of E/S and hydrolysis time on DH of CCPI at temperature 50 °C and pH 7.0. | |
3.2 Gel chromatography of CCPIH and antioxidant activity of the fractions
3.2.1 G-25 gel chromatography of CCPIH. As shown in Fig. 4a, five fractions were collected separately after the Sephadex G-25 gel chromatography. Fraction E with the lowest molecular weight (Mw) showed the highest ·OH scavenging activity at all tested concentrations (50–250 μg mL−1, Fig. 4b and 5a). Since ·OH is the most reactive and highly damaging species in free radical pathology and it can attack almost every molecule in living cells.1 Hence, it was selected as the indicator for purification of antioxidant peptides in this paper.
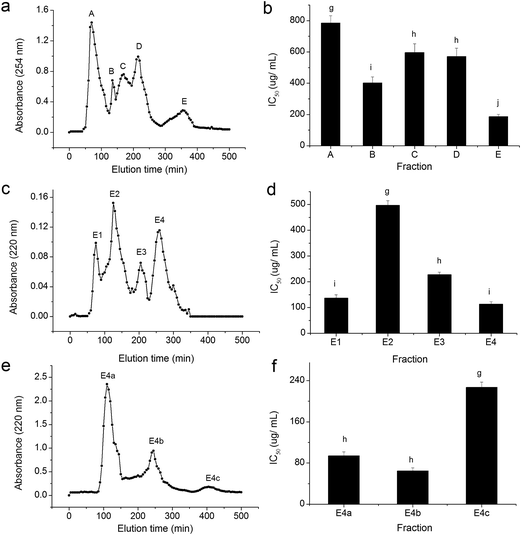 |
| Fig. 4 Sephadex G-25 gel chromatography profile of CCPIH (a) and IC50 of each fraction on hydroxyl radical (b); fraction E was further separated by SP Sephadex C-25 gel chromatography (c) and IC50 of each fraction on ·OH (d); fraction E4 was separated by Sephadex G-15 gel chromatography (e) and IC50 of fractions on ·OH (f); (g–j) different small letters on the bar meant significant difference (P < 0.05). | |
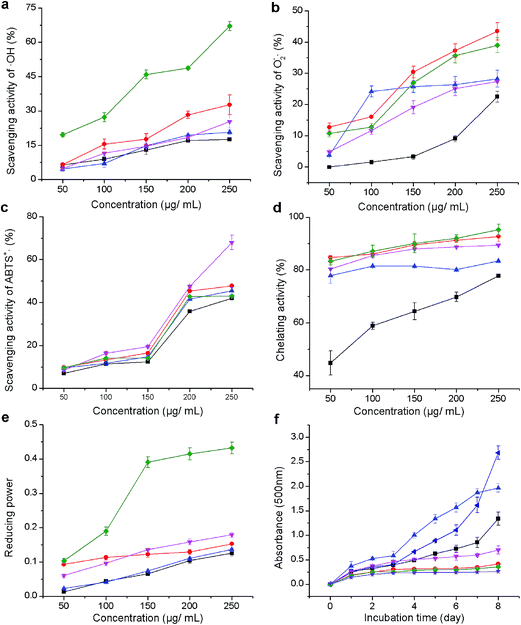 |
| Fig. 5 Antioxidant activity of fraction A–E including scavenging activity on hydroxyl radical (a), superoxide radical (b) and ABTS radical (c), chelating ability (d), reducing power (e) and inhibition of linoleic acid peroxidation (f). ■ − fraction A, ●-fraction B, ▲-fraction C, ▼-fraction D, ◆-fraction E, ◀-control, the autoxidation of linoleic acid, ★-PG (propyl gallate) was used as the comparison. | |
3.2.2 Amino acid composition analysis. Result in Table 4 showed that though CCPIH fractions showed different amino acid profiles, their content of arginine and glutamic acid was all high. Fraction D showed the highest NPS (the frequency of nonpolar side chains), indicating its high content of nonpolar amino acids. Both fraction E and B showed a higher NPS, a higher content of sulfur amino acid (methionine and cysteine) and aromatic amino acid (phenylalanine, tryptophan and tyrosine), which was probably the reason for their high scavenging activity on ·OH. For it was pointed out that hydrophobic amino acid residues could increase the presence of peptides at the water–lipid interface and facilitate the access to scavenging free radicals.30,31 Meanwhile the phenolic groups in aromatic amino acid could serve as hydrogen donors and inhibited the radical-mediated peroxidizing chain reaction.4,32
Table 4 Amino acid composition (g/100 g protein) and Bigelow parameters of coconut cake protein (CCPI) and its hydrolysate fractionsa
Amino acid |
Coconut cake protein hydrolysate fractions |
FAO/WHO, 1990 |
CCPI |
A |
B |
C |
D |
E |
Different letters (a–d) in the same row indicate a significant difference (P < 0.05). The total essential amino acid. Ratio of essential amino acid to total amino acids. The frequency of nonpolar side chains. Ratio of polar to nonpolar side chains. |
|
0–12 months |
2–5 years |
![[thin space (1/6-em)]](https://www.rsc.org/images/entities/char_2009.gif) |
Essential amino acid |
Threonine |
2.95 ± 0.12a |
2.44 ± 0.15b |
2.93 ± 0.14a |
1.23 ± 0.09c |
1.47 ± 0.11c |
2.43 ± 0.11b |
4.3 |
3.4 |
Valine |
4.43 ± 0.15b |
4.02 ± 0.04b |
5.47 ± 0.26a |
1.91 ± 0.11d |
3.47 ± 0.16c |
5.07 ± 0.21a |
5.5 |
3.5 |
Lysine |
4.70 ± 0.22a |
3.01 ± 0.08b |
3.06 ± 0.31b |
1.06 ± 0.18c |
0.70 ± 0.16d |
3.00 ± 0.14b |
6.6 |
5.8 |
Methionine |
1.53 ± 0.09c |
2.08 ± 0.11b |
2.39 ± 0.09b |
1.35 ± 0.12c |
2.01 ± 0.11c |
3.94 ± 0.26a |
|
|
Met ± Cys |
3.73 ± 0.11a |
2.08 ± 0.11b |
2.39 ± 0.09b |
1.35 ± 0.12d |
2.01 ± 0.11c |
3.94 ± 0.26a |
4.2 |
2.5 |
Isoleucine |
3.52 ± 0.19a |
2.20 ± 0.14b |
3.26 ± 0.17a |
1.11 ± 0.24d |
1.65 ± 0.14c |
3.66 ± 0.45a |
4.6 |
2.8 |
Leucine |
6.15 ± 0.30a |
3.52 ± 0.02c |
5.62 ± 0.08b |
2.00 ± 0.38d |
3.77 ± 0.08c |
5.26 ± 0.45b |
9.3 |
6.6 |
Phenylalanine |
4.45 ± 0.49a |
2.63 ± 0.01c |
2.95 ± 0.02b |
1.89 ± 0.16d |
2.81 ± 0.01b |
4.50 ± 0.28a |
|
|
Tyrosine |
2.80 ± 0.08b |
2.97 ± 0.22b |
3.62 ± 0.21a |
2.66 ± 0.21b |
3.30 ± 0.07a |
3.85 ± 0.15a |
|
|
Histidine |
2.02 ± 0.17a |
0.99 ± 0.15c |
1.31 ± 0.20b |
0.76 ± 0.09c |
1.41 ± 0.17b |
2.21 ± 0.17a |
2.6 |
1.9 |
Tryptophan |
1.02 ± 0.08a |
0.24 ± 0.03c |
0.31 ± 0.02c |
0.22 ± 0.02c |
0.22 ± 0.01c |
0.93 ± 0.08b |
1.1 |
0.5 |
TEAAb |
35.67 ± 0.23b |
26.58 ± 0.25c |
32.70 ± 0.10b |
14.74 ± 0.34d |
21.32 ± 0.28d |
39.35 ± 1.10a |
33.9 |
12.7 |
![[thin space (1/6-em)]](https://www.rsc.org/images/entities/char_2009.gif) |
Nonessential amino acid |
Arginine |
12.34 ± 1.24a |
5.94 ± 0.32d |
8.96 ± 1.18b |
3.63 ± 0.21b |
5.03 ± 1.45d |
6.09 ± 0.2c |
|
|
Aspartic acid |
8.17 ± 0.44a |
6.50 ± 0.22b |
7.41 ± 0.33b |
2.82 ± 0.18d |
3.00 ± 0.19c |
8.41 ± 0.60a |
|
|
Serine |
4.22 ± 0.25a |
2.61 ± 0.22c |
3.26 ± 0.19b |
1.55 ± 0.14d |
1.71 ± 0.10d |
4.26 ± 0.31a |
|
|
Glutamic acid |
22.40 ± 0.82a |
13.29 ± 0.35b |
15.36 ± 0.21b |
5.51 ± 0.13c |
6.08 ± 0.05c |
14.51 ± 0.27b |
|
|
Glycine |
4.95 ± 0.25a |
2.61 ± 0.15c |
3.51 ± 0.24b |
1.22 ± 0.19d |
1.75 ± 0.14d |
3.86 ± 0.15b |
|
|
Alanine |
8.04 ± 0.39a |
4.32 ± 0.17c |
5.88 ± 0.24b |
3.22 ± 0.16d |
4.21 ± 0.21c |
4.05 ± 0.14c |
|
|
Proline |
3.11 ± 0.35b |
2.70 ± 0.07c |
3.08 ± 0.28b |
0.77 ± 0.17d |
0.71 ± 0.20d |
5.41 ± 0.31a |
|
|
![[thin space (1/6-em)]](https://www.rsc.org/images/entities/char_2009.gif) |
Bigelow parameters |
E/Tc (%) |
36.40d |
42.97c |
42.16c |
45.10b |
49.48a |
48.85a |
|
|
NPSd |
0.27 ± 0.08d |
0.32 ± 0.01d |
0.34 ± 0.01c |
0.36 ± 0.01c |
0.41 ± 0.01a |
0.39 ± 0.01b |
|
|
Pe |
2.65 ± 0.15a |
2.07 ± 0.04a |
1.96 ± 0.20b |
1.80 ± 0.02b |
1.42 ± 0.08c |
1.54 ± 0.13c |
|
|
3.2.3 Antioxidant activity of CCPIH fractions. Fraction D showed the highest protective effect (76.25%) against DNA damage (Fig. 6), probably resulting from its high NPS (Table 4). Besides, fraction B and E also showed good protective ability, in accordance with their high radical scavenging ability (Fig. 5). Previous study also indicated that DNA damage could be inhibited by scavenging the radicals produced during the oxidation reactions or inhibiting the generation of radicals.33
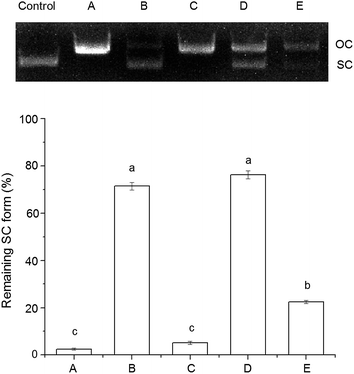 |
| Fig. 6 Protection of CCPIH fractions on DNA damage at concentration of 2 mg mL−1. A–E, CCPIH fraction A to E; SC, super-coiled form; OC, open-circular form; different small letters on the bar meant significant difference (P < 0.05). | |
As shown in Fig. 5b, all CCPIH fractions showed considerable superoxide radical scavenging activity and the activity increased with increasing concentration. The fraction E exhibited higher ability than fraction A and C, may be due to its relatively lower Mw and significantly higher NPS (P < 0.05, Table 4).27 Result in Fig. 5c showed that all the fractions exhibited high ABTS radical-scavenging activity at concentration of 200–250 μg mL−1, probably contributed to their relatively high content of aromatic amino acid (Table 4).32 Moreover, all fractions especially fraction E exhibited excellent chelating capacity even though at low concentration (Fig. 5d). This may be attributed to the abundance of Glu and Asp (Table 4), which could interact with metal ions through their charged groups and inactivate the prooxidant activity of metal ions.30 The highest reducing power was also found in fraction E (Fig. 5e), perhaps due to its high content of aromatic amino acid.4 Furthermore, fraction E also exhibited good inhibition ability against linoleic acid autoxidation (Fig. 5f), probably resulted from its high NPS (Table 4). It was reported that hydrophobic amino acids could enhance the lipophilicity of peptides and promote the synergy between each other to inhibit fat peroxidation.31 In general, the fraction E was selected to further purification and characterization for its high antioxidant activity.
3.3 Purification of the antioxidant peptides
Through the SP Sephadex C-25 gel chromatography, the fraction E was separated into four major fractions (E1, E2, E3 and E4, Fig. 4c–d). The fraction E4 was chose to the next separation for its highest ·OH scavenging activity with IC50 106.76 ± 2.08 μg mL−1 (Table 5). In the Sephadex G-15 gel chromatography of fraction E4, three main fractions were obtained and the activity of E4b (IC50 = 59.73 ± 2.64 μg mL−1) was much higher than that of E4a and E4c (P < 0.05, Fig. 4e–f).
Table 5 Purification of E4b3-p from coconut cake protein isolates hydrolysates, and their IC50 on hydroxyl radical scavenging activity
Fraction |
Step |
IC50 (μg mL−1) |
E |
Sephadex G-25 |
185.48 ± 1.50 |
E4 |
SP Sephadex C-25 |
106.76 ± 2.08 |
E4b |
Sephadex G-15 |
59.73 ± 2.64 |
E4b3 |
RP-HPLC semi-preparative column |
9.22 ± 0.31 |
E4b3-1 |
RP-HPLC analytical column |
4.28 ± 0.28 |
E4b3-2 |
RP-HPLC analytical column |
7.65 ± 0.15 |
The fraction E4b was further isolated by RP-HPLC on the semi-preparative C18 column and 13 major peaks named as E4b1–E4b13 were presented and collected (Fig. 7a). The E4b3 with the lowest IC50 (9.22 ± 0.31 μg mL−1) was further purified by RP-HPLC in an analytical C18 column (Φ4.6 mm × 250 mm). Two fractions named E4b3-1 and E4b3-2 with IC50 of 4.28 ± 0.28 and 7.65 ± 0.15 μg mL−1 were obtained (Fig. 7b, Table 5). Finally, fraction E4b3-1 and E4b3-2 were further separately purified on the same analytical C18 column.
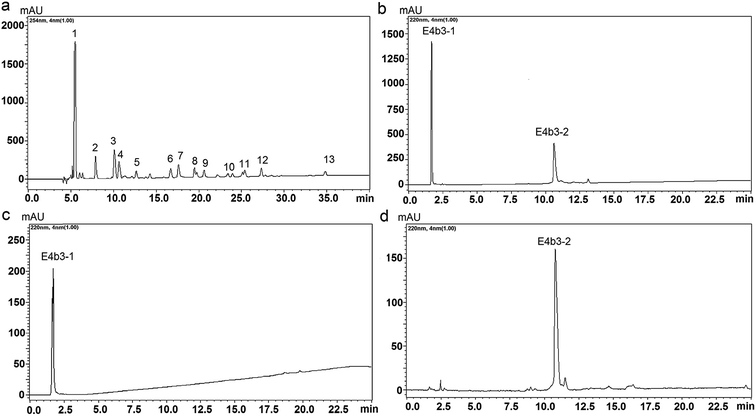 |
| Fig. 7 (a) Chromatography of E4b separated by semi-preparing RP-HPLC with the linear gradient of acetonitrile (5–30% in 30 min) containing 0.1% TFA at a flow rate of 2.5 mL min−1. Number 1–13 represented the elution peaks of E4b1–E4b13. (b) E4b3 was separated by analytical RP-HPLC at flow rate of 1 mL min−1 with the linear gradient of acetonitrile (5–25% in 20 min) containing 0.1% TFA. E4b3-1 (c) and E4b3-2 (d) were further purified and confirmed by RP-HPLC analytical column with the linear gradient of acetonitrile (5–25% in 20 min) containing 0.1% TFA. | |
3.4 Characterization of purified peptides
As shown in Fig. 8, the Mw of E4b3-1 and E4b3-2 were 865.02 Da with amino acid sequence Pro-Gln-Phe-Tyr-Trp and 612.36 Da with sequence Arg-Pro-Glu-Ile-Val. Obviously, E4b3-1 was rich in aromatic amino acid and hydrophobic amino acid residues. This may be the reason why E4b3-1 with higher Mw but exhibited lower IC50 value than E4b3-2. The size of E4b3-1 and E4b3-2 were lower than the peptides from rice residue protein, alfalfa leaf and conger ell (940.0, 1000 and 928 Da, respectively),34–36 but bigger than the peptides isolated from plum stones, loach, rice bran and walnut (530.3, 464.2 and 423.23 Da, respectively).7,31,37,38 Though Mw of peptide played an important role on its antioxidant activity,27 E4b3-1 exhibited a higher antioxidant activity (IC50 = 4.28 μg mL−1) than those smaller peptides from rice residue protein (43.55 μg mL−1),7 loach (26.4 μg mL−1)31 and walnut (310.0 μg mL−1).38 This was presumably due to its high content of hydrophobic amino acid and aromatic amino acid residues.4,32
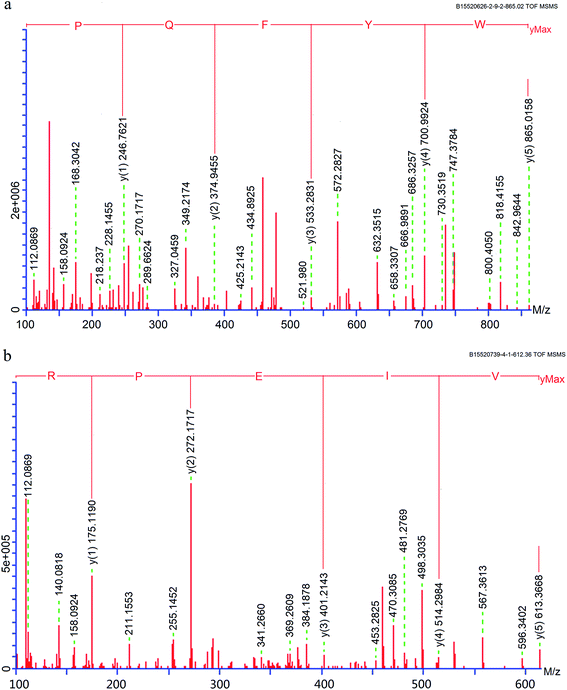 |
| Fig. 8 Identification of molecular mass and amino acid sequences of peptide E4b3-1 (a) and E4b3-2 (b) by Nano-LC-ESI-Q-TOF MS/MS. | |
Furthermore, the amino acid sequence of E4b3-1 and E4b3-2 was another important reason for their high activity. You et al.28 found that the peptides (Pro-Ser-Tyr-Val, Pro-His-His and Pro-Asn-Arg-Pro-Gln-Phe) with Pro at C-terminal could show strong ·OH scavenging ability. The peptides with Val residues either at C-terminal or N-terminal (e.g. Val-Lys-Leu, Val-Lys-Val and Val-Asp-Tyr-Pro) showed high antioxidant activity.30 Wattanasiritham et al.7 found that peptides rich in Gln residues showed high antioxidant activity. Furthermore, it has been demonstrated that peptides with Phe–Tyr or Tyr–Trp at N-terminal always exhibited excellent antioxidant ability.5,30 These were all in accordance with the result in this paper.
3.5 Stability of synthetic peptides
The purity of the synthetic peptides (E4b3-1 and E4b3-2) was all more than 95%. After the digestion with pepsin and pancreatin, the antioxidant activity of synthetic peptides was not significantly decreased (Fig. 9), meaning that both E4b3-1 and E4b3-2 retained their activity well in gastrointestinal digestion system.
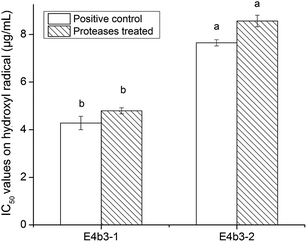 |
| Fig. 9 Stability of synthetic peptides E4b3-1 and E4b3-2 against the simulated gastrointestinal digestion. The synthetic peptides untreated by proteases were designed as positive control. Different letters on the bar meant significant difference (P < 0.05). | |
4. Conclusions
The optimal hydrolysis conditions of CCPI with papain were 49.09 °C, pH 6.68, concentration of CCPI 5% (m/v), E/S 0.97 (g/100 g) and time was 6.45 h. Two peptides E4b3-1 (Pro-Gln-Phe-Tyr-Trp, 865.02 Da, IC50 = 4.28 μg mL−1) and E4b3-2 (Arg-Pro-Glu-Ile-Val, 612.36 Da, IC50 = 7.65 μg mL−1) were purified, identified and synthesized. The synthetic peptides exhibited good stability in gastrointestinal digestion system. However, whether these peptides have activity in vivo need further works.
Acknowledgements
This work was supported by Chinese program of Science and Technology of 12th Five-year Plan (No. 2012BAD31B03).
References
- M. F. Beal, Mitochondria, free radicals and neurodegneration, Curr. Opin. Neurobiol., 1996, 6, 661–666 CrossRef CAS PubMed.
- E. R. Stadtman, Protein oxidation and aging, Free Radical Res., 2006, 40, 1250–1258 CrossRef CAS PubMed.
- S. Y. Dong, M. Y. Zeng, D. F. Wang, Z. Y. Liu, Y. H. Zhao and H. C. Yang, Antioxidant and biochemical properties of protein hydrolysates prepared from Silver carp (Hypophthalmichthys molitrix), Food Chem., 2008, 107, 1483–1493 CrossRef.
- B. H. Sarmadi and A. Ismail, Antioxidative peptides from food proteins: a review, Peptides, 2010, 31, 1949–1956 CrossRef CAS PubMed.
- Y. F. Zhang, X. Duan and Y. L. Zhuang, Purification and characterization of novel antioxidant peptides from enzymatic hydrolysates of tilapia (Oreochromis niloticus) skin gelatin, Peptides, 2012, 38, 13–21 CrossRef PubMed.
- Y. J. Zheng, Y. Li, Y. L. Zhang, R. G. Zhang, Q. A. Zhang, Y. F. Zhang and S. L. Zhao, Fractionation, Physicochemical Properties, Nutritional Value, Antioxidant Activity and ACE Inhibition of Palm Kernel Expeller Protein, RSC Adv., 2015, 5, 12613–12623 RSC.
- L. Wattanasiritham, C. Theerakulkait, S. Wickramasekara, C. S. Maier and J. F. Stevens, Isolation and identification of antioxidant peptides from enzymatically hydrolyzed rice bran protein, Food Chem., 2016, 192, 156–162 CrossRef CAS PubMed.
- K. Kwon, K. H. Park and K. C. Rhee, Fractionation and characterization of proteins from coconut (Cocos nucifera L.), J. Agric. Food Chem., 1996, 44(7), 1741–1745 CrossRef CAS.
- M. R. N. Angelia, R. N. Garcia, K. M. P. Caldo, K. Prak, S. Utsumi and E. M. Tecson-Mendoza, Physicochemical and functional characterization of cocosin, the coconut 11S globulin, Food Sci. Technol. Int., 2010, 16(3), 225–232 CrossRef CAS PubMed.
- S. Mini and T. Rajamohan, Influence of coconut kernel protein on lipid metabolism in alcohol fed rats, Indian J. Exp. Biol., 2004, 42, 53–57 CAS.
- H. X. Wang and T. B. Ng, An antifungal peptide from the coconut, Peptides, 2005, 26, 2392–2396 CrossRef CAS PubMed.
- S. M. Mandal, S. Dey, M. Mandal, S. Sarkar, S. Maria-Neto and O. L. Franco, Identification and structural insights of three novel antimicrobial peptides isolated from green coconut water, Peptides, 2009, 30, 633–637 CrossRef CAS PubMed.
- M. J. Santana, A. L. D. Olivera, L. H. K. Q. Júnior, S. M. Mandal, C. O. Matos, R. D. Dias, O. L. Franco and L. M. Liao, Structural insights into Cn-AMP1, a short disulfide-free multifunctional peptide from green coconut water, FEBS Lett., 2015, 589, 639–644 CrossRef CAS PubMed.
- S. Thaiphanit and P. Anprung, Physicochemical and emulsion properties of edible protein concentrate from coconut (Cocos nucifera L.) processing by-products and the influence of heat treatment, Food Hydrocolloids, 2016, 52, 756–765 CrossRef CAS.
- J. Adler-Nissen, Determination of the degree of hydrolysis of food protein hydrolysates by trinitrobenzenesulfonic acid, J. Agric. Food Chem., 1979, 27, 1256–1262 CrossRef CAS PubMed.
- S. Sakanaka and Y. Tachibana, Active oxygen scavenging activity of egg-yolk protein hydrolysates and their effects on lipid oxidation in beef and tuna homogenates, Food Chem., 2006, 95, 243–249 CrossRef CAS.
- X. S. Wang, C. H. Tang, X. Q. Yang and W. R. Gao, Characterization, amino acid composition and in vitro digestibility of hemp (Cannbis sativa L.) proteins, Food Chem., 2008, 107, 11–18 CrossRef CAS.
- C. C. Bigelow, On the coverage hydrophobicity of proteins and the relationship between it and protein structure, J. Theor. Biol., 1976, 16, 187–211 CrossRef.
- S. Marklund and G. Marklund, Involvement of the superoxide anion radical in the autoxidation of pyrogallal and a convenient assay for superoxide dismutase, Eur. J. Biochem., 1974, 47, 469–474 CrossRef CAS PubMed.
- J. T. J. Mariken, J. Arts, S. Dallinga, H. P. Voss, G. R. M. M. Haenen and A. Bast, A new approach to assess the total antioxidant capacity using the TEAC assay, Food Chem., 2004, 88, 567–570 CrossRef.
- B. H. Kong and Y. L. Xiong, Antioxidant activity of zein hydrolysates in a liposome system and the possible mode of action, J. Agric. Food Chem., 2006, 54, 6059–6068 CrossRef CAS PubMed.
- I. F. F. Benzie and J. J. Strain, The ferric reducing ability of plasma (FRAP) as a measure of “antioxidant power”: the FRAP assay, Anal. Biochem., 1996, 239, 70–76 CrossRef CAS PubMed.
- H. C. Wu, H. M. Chen and C. Y. Shiau, Free amino acids and peptides as related to antioxidant properties in protein hydrolysates of mackerel (Scomber austriasicus), Food Res. Int., 2003, 36, 949–957 CrossRef CAS.
- H. Mitsuda, K. Yasumoto and K. Iwami, Antioxidative action of indole compounds during the autoxidation of linoleic acid, J. Jpn. Soc. Nutr. Food Sci., 1966, 19, 210–214 CAS.
- J. B. Jeong, B. O. De Lumen and H. J. Jeong, Lunasin peptide purified from Solanum nigrum L. protects DNA from oxidative damage by suppressing the generation of hydroxyl radical via blocking Fenton reaction, Cancer Lett., 2010, 293, 58–64 CrossRef CAS PubMed.
- T. Tavares, M. D. Contreras, M. Amorim, M. Pintado, I. Recio and F. X. Malcata, Novel whey-derived peptides with inhibitory effect against angiotensin-converting enzyme: in vitro effect and stability to gastrointestinal enzymes, Peptides, 2011, 32, 1013–1019 CrossRef CAS PubMed.
- A. Alemán, B. Giménez, E. Pérez-Santin, M. C. Gómez-Guillén and P. Montero, Contribution of Leu and Hyp residues to antioxidant and ACE-inhibitory activities of peptide sequences isolated from squid gelatin hydrolysate, Food Chem., 2011, 125, 334–341 CrossRef.
- L. You, M. Zhao, J. M. Regenstein and J. Ren, Purification and identification of antioxidative peptides from loach (Misgurnus anguillicaudatus) protein hydrolysate by consecutive chromatography and electrospray ionization-mass spectrometry, Food Res. Int., 2010, 43, 1167–1173 CrossRef CAS.
- A. M. Joglekar and A. T. May, Product excellence through design of experiments, Cereal Foods World, 1987, 32, 857–868 Search PubMed.
- F. Shahidi and Y. Zhong, Novel antioxidants in food quality preservation and health promotion, Eur. J. Lipid Sci. Technol., 2010, 112, 930–940 CrossRef CAS.
- A. G. P. Samaranayaka and E. C. Y. Li-Chan, Food-derived peptidic antioxidants: a review of their production, assessment, and potential applications, J. Funct. Foods, 2011, 3, 229–254 CrossRef CAS.
- X. H. Kou, J. Gao, Z. H. Xue, Z. J. Zhang, H. Wang and X. Wang, Purification and identification of antioxidant peptides from chickpea (Cicerarietinum L.) albumin hydrolysates, LWT--Food Sci. Technol., 2013, 50, 591–598 CrossRef CAS.
- S. S. Leonard, D. Keil, T. Mehlman, S. Proper, X. Shi and G. K. Harris, Essiac tea: Scavenging of reactive oxygen species and effects on DNA damage, J. Ethnopharmacol., 2006, 103, 288–296 CrossRef CAS PubMed.
- Q. J. Yan, L. H. Huang, Q. Sun, Z. Q. Jiang and X. Wu, Isolation, identification and synthesis of four novel antioxidant peptides from rice residue protein hydrolyzed by multiple proteases, Food Chem., 2015, 179, 290–295 CrossRef CAS PubMed.
- Z. Xie, J. Huang, X. Xu and Z. Jin, Antioxidant activity of peptides isolated from alfalfa leaf protein hydrolysate, Food Chem., 2008, 111, 370–376 CrossRef CAS PubMed.
- S. Ranathunga, N. Rajapakse and S. K. Kim, Purification and characterization of antioxidative peptide derived from muscle of conger eel (Conger myriaster), Eur. Food Res. Technol., 2006, 222, 310–315 CrossRef CAS.
- E. González-García, P. Puchalska, M. L. Marina and M. C. García, Fractionation and identification of antioxidant and angiotensin-converting enzyme-inhibitory peptides obtained from plum (Prunus domestica L.) stones, J. Funct. Foods, 2015, 19, 376–384 CrossRef.
- N. Chen, H. Yang, S. Yi, J. Niu and S. Liu, Purification and identification of antioxidant peptides from walnut (Juglans regia L.) protein hydrolysates, Peptides, 2012, 38, 344–349 CrossRef CAS PubMed.
Footnote |
† The two authors contribute equally to this work and they are co-first author. |
|
This journal is © The Royal Society of Chemistry 2016 |