DOI:
10.1039/C6RA06881B
(Paper)
RSC Adv., 2016,
6, 42863-42868
Access to steroidal pyridazines via modified thiohydrazides†
Received
15th March 2016
, Accepted 20th April 2016
First published on 25th April 2016
Abstract
An approach to steroids annulated with pyridazines via cascade imination/electrocyclization of chlorovinyl aldehydes with oxamic acid thiohydrazides is disclosed. A mechanistic rationalization was performed using real-time 1H NMR spectroscopy and computational studies. A series of 18-nor-5α-androsta-2,13-diene[3,2-d]pyridazines, androsta-2-ene[3,2-d]pyridazines and Δ1,3,5(10)-estratrieno[16,17-d]pyridazines were synthesized from native hormones. These compounds were screened for cytotoxicity against the human estrogen-responsive breast cancer cell line MCF-7 and the estrogen-independent breast cancer cell line MDA-MB-231. The structure–activity relationship analysis revealed that the annulation of the pyridazine moiety to the A-ring of the 17β-hydroxy-5α-androsta-2-ene core provides high antiproliferative activity. Compounds 7a and 10b exhibited higher antiproliferative potency than the drug cisplatin. 5α-Androsta-2-ene[3,2-d]pyridazine 10c showed good selectivity against the MCF-7 breast cancer cells.
Introduction
The development of methods for rapid access to N-heterocycles from simple and readily available starting compounds via the formation of several bonds as a one-pot reaction has become one of the main challenges in organic synthesis. In this regard, transformations of hydrazones have emerged as a powerful tool for the atom-economical chemoselective construction of (hetero)cyclic systems with complexity and diversity.1 The synthetic utility of hydrazones extends far beyond the preparation of indoles via the classical Fischer reaction.2 Hydrazones have found applications in the total synthesis of natural products as practical chiral auxiliaries3 and have been reported as useful ligands4 and catalysts for asymmetric reactions.5 The unique reactivity of N-tosylhydrazones6 as precursors of unstabilized diazo compounds enabled the synthesis of isoindolines,7 1H-indazoles,8 azetidin-2-ones,9 pyrroles,10 pyrrolidines,11 etc.;12 N,N′-dialkylhydrazones1b proved to be excellent Michael acceptors,13 good azomethine nitrogen atom nucleophiles14 and radical acceptors in cyclizations.15 Besides, α,β-unsaturated hydrazones bearing the 1-azadiene unit are employed in hetero-Diels–Alder reactions.16 Examples of the use of donor–acceptor substituted hydrazones as acyl anion equivalents that undergo heterocyclization with α,β-unsaturated aldehydes or keto esters were reported.13c,d,17 Modified hydrazones with C
O, C
S, C
N and P
O functional groups are applied in the synthesis of diverse five- and six-membered O,N,S-heterocycles,18 including such widely used pharmacophores as 1,3,4-thiadiazoles19 and pyrazoles.20 All this clearly attests to the power of hydrazone chemistry to reach into new areas of (hetero)cyclic chemical space, aromatic and saturated alike.
Recently, we have reported a new type of reactivity of α,β-unsaturated hydrazones of thiohydrazides as 2,3-diazahexatriene synthons (Scheme 1).21 We elaborated a two-step procedure for the synthesis of highly substituted pyridazines employing (1) the Vilsmeier–Haack reaction of enolizable ketones giving chlorovinyl aldehydes and (2) imination of the former with oxamic acid thiohydrazides followed by cascade electrocyclization/aromatization of the resulting 2,3-diazahexatrienes. The aim of this work was to develop an efficient method for transformations of complex natural products in order to modulate the biological activity of substrates.
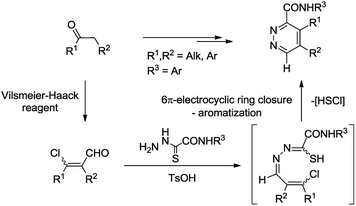 |
| Scheme 1 Transformation of ketones into pyridazines via α,β-unsaturated hydrazones of thiohydrazides. | |
In this respect, we sought to steroids comprising one of the largest and most diverse classes of natural products. Native hormones are versatile modulators that regulate most of the key functions in the mammalian body. Their A- and D-ring modified heterocyclic derivatives, over the years, have been considered to be a privileged scaffold for the drug discovery, including the design of anticancer agents.22 Synthetic azasteroids encompass a wide range of compounds with antiproliferative activity, e.g., reductase inhibitors such as finasteride,23 high-affinity agonist ligands for the glucocorticoid receptor, e.g., cortivazol,24 GnRH agonists such as danazol,25 aromatase inhibitors such as formestane and exemestane26 (Fig. 1). Herein, we report the synthesis of unique derivatives of the androstene and estrane series containing pyridazine motifs annulated to the A- and D-rings of the steroid core and the evaluation of their inhibitory activity against breast cancer cells.
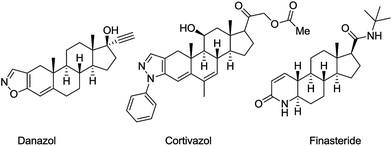 |
| Fig. 1 Synthetic heterosteroid drugs. | |
Results and discussion
Chemistry
Based on our previous work, we initially prepared 3-chloro-2-formylandrostane 2 from 3-keto-17α-methyl-17β-hydroxyandrostane (1) by the treatment with the Vilsmeier–Haack reagent under standard conditions (Scheme 2). The chlorovinylation was accompanied by the Wagner–Meerwein rearrangement resulted in the elimination of 17-OH group and migration of 18-Me group to C-17 position. Meanwhile, the subsequent reactions of 3-chloro-2-formyl-17,17-dimethyl-18-norandrostane-2,13-diene (2) with thiohydrazides 3a–c containing both electron-donating and withdrawing substituents on the benzoyl group proceeded smoothly. The reflux for 2 h in ethanol in the presence of a catalytic amount of TsOH afforded A-ring modified 18-nor-5α-androsta-2,13-diene[3,2-d]pyridazines 4a–c in 51–82% yields.
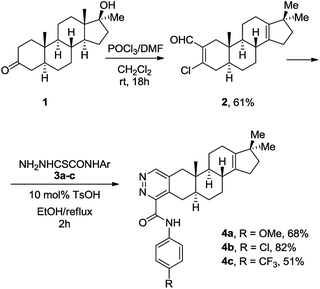 |
| Scheme 2 Synthesis of 18-nor-5α-androsta-2,13-diene[3,2-d]pyridazines 4. | |
In order to avoid side rearrangements and ensure an efficient synthesis process, we decided to use 3-keto-17β-hydroxyandrostane 5. The reaction of ketone 5 with the Vilsmeier–Haack reagent during prolonged storage at rt produced 3-chloro-2-formylandrostane 6 in 61% yield (Scheme 3). In this case, the chlorovinylation was followed by esterification of 17-OH group. Fortunately, the desired heterocyclization of 6 with oxamic acid thiohydrazides 3a, 3b was accompanied by the release of 17-OH group, giving appropriate 17β-hydroxy-5α-androsta-2-ene[3,2-d]pyridazines 7a, 7b. The reactions were completed in 3 h in ethanol under reflux with TsOH (10 mol%) resulting in the formation of 7a, 7b in 73% and 67% yields.
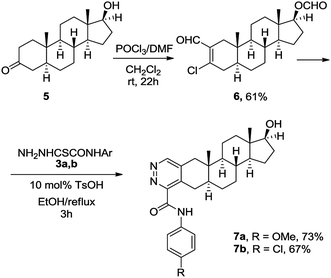 |
| Scheme 3 Synthesis of 5α-androsta-2-ene[3,2-d]pyridazines 7. | |
Then we made an attempt to generate D-ring modified androstene derivatives from 17-chloro-16-formyl steroid 9 derived from 3β-acetoxyandrostene 8 (Table 1). Having so far observed a seemingly general transformation pattern, we found that the reaction of chlorovinyl aldehyde 9 with thiohydrazides 3 affords androst-5-ene[16,17-d]pyridazines 10a–f in high yields. Acid thiohydrazides 3a–f containing electron-donating and-withdrawing groups were tested. The reaction showed high chemoselectivity and functional group tolerance. It is remarkable that the acetyl protecting group could be preserved or removed depending on pH of the reaction mixture in the heterocyclization step. Once TsOH was washed out after the intermediate formation of hydrazone, 3β-acetoxy-androst-5-ene[16,17-d]pyridazines 10a–c were isolated as the only products (Table 1, procedure A). Meanwhile, the reaction was accompanied by the deprotection of the 3-hydroxy group producing 10a′,10b′,10e′,10f′ under reflux in the presence of TsOH (Table 1, procedure B).
Table 1 Synthesis of androsteno[16,17-d]pyridazines 10ab
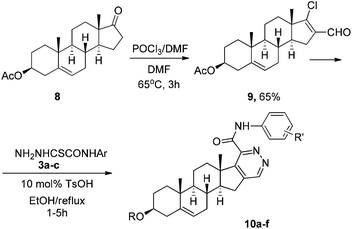
|
Reaction conditions. Procedure A: androsta-5,16-diene 9 (0.1 mmol), oxamic acid thiohydrazide 3 (0.1 mmol), TsOH·H2O (10 mol%) and ethanol (30 mL) were stored at rt for 30 min. TsOH was washed out and the reaction mixture was refluxed for 1 h. Procedure B: androsta-5,16-diene 9 (0.1 mmol), oxamic acid thiohydrazide 3 (0.1 mmol), TsOH·H2O (10 mol%) and ethanol (30 mL) were refluxed for 3–5 h. Isolated yield. |
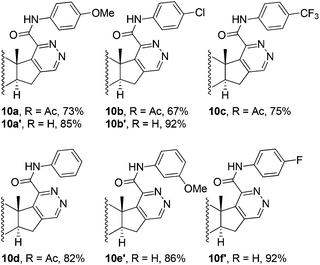 |
Since 3-oxo-Δ4-azasteroids are well-known as 5-α-reductase inhibitors,27 the Oppenauer oxidation was used for compounds 10a′ and 10f′ producing Δ4-3-oxo[17,16-d]pyridazines 11a, 11f in good yields (Scheme 4).
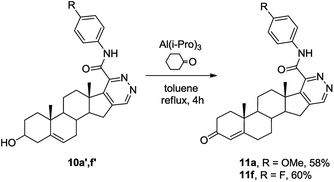 |
| Scheme 4 Oppenauer oxidation of compounds 10. | |
We next examined the scope of the method employing 17-chloro-16-formyl-Δ1,3,5(10),16-estratetraenol 13 – chlorovinylation product of estrone (12). The reaction shown in Scheme 5 resulted in the formation of the Δ1,3,5(10)-estratrieno[16,17-d]pyridazines 14a, 14b, 14g in 79–91% yields. The reactions were accomplished under standard conditions by heating under reflux for 2 h in ethanol in the presence of 10 mol% of TsOH.
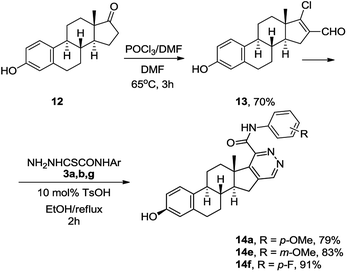 |
| Scheme 5 Synthesis of pyridazines 14. | |
It should be noted that the structural assignments for all compounds 4, 7, 10 and 14 were confirmed by 2D NMR (1H–1H COSY, 1H–1H TOCSY, 1H–1H ROESY, 13C–1H HMBC, and 13C–1H HSQC) techniques and HRMS.
The possible mechanism for the formation of steroidal pyridazines by the reaction of chlorovinyl aldehydes with oxamic acid thiohydrazides is shown in Scheme 6 illustrating an example of product 10a. Relying on the literature,28 the mechanism involves disrotatory 6π-electrocyclization of highly reactive Z-15-thiol isomer into dihydropyridine 16 followed by aromatization with elimination of hydrochloric acid and elemental sulfur.29 The evaluated activation barriers for electrocyclization step are in the range of 2.5–5.7 kcal mol−1 for the products obtained (ΔHr = 3.3–7.3 kcal mol−1, PM6, see ESI†).
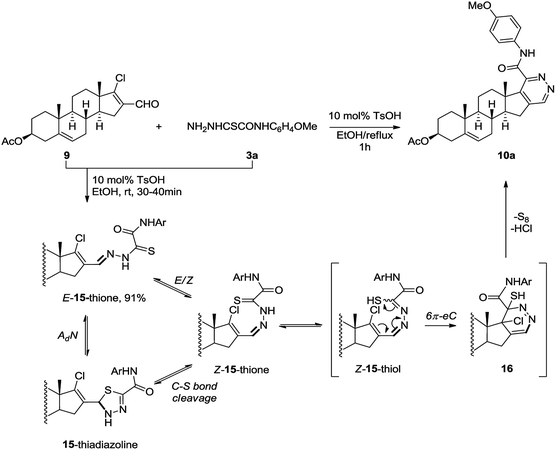 |
| Scheme 6 Proposed mechanistic pathway. | |
In order to elucidate the pattern of the critical build up of Z-15-thiol, we isolated intermediate hydrazone 15 in 91% yield after short-time storage of a mixture of aldehyde 9 and thiohydrazide 3a at rt in the presence of TsOH (10 mol%). In relatively non-polar CDCl3, as well in the solid state,30 hydrazone 15 was found to exist as the most stable E-15-thione, resulting in that no isomerization or formation of product 10a occur even after 100 h of storage at rt (for detailed spectra see ESI†). However, the solution-state structure of compound 15 in polar DMSO-d6 immediately after dissolution corresponded to a mixture of E-15-thione and 15-thiadiazoline isomers in a 1.3
:
1.0 ratio. The minor isomer Z-15-thione was additionally detected (in DMSO-d6) using real-time monitoring of the heterocyclization step at rt by 1H NMR spectroscopy in an NMR tube. The observed solvent effect was attributed to the nucleophilic attack of the S-nucleophile at the C
N bond of E-15-thione giving 15-thiadiazoline, which is enhanced in polar solvents. In that context, the pathway of the formation of Z-15-thione isomer through 15-thiadiazoline via the C–S bond cleavage, at least at rt in the absence of an acidic catalyst, seems to play a significant role. The subsequent thione–thiol tautomerism results in the conversion of Z-15-thione into Z-15-thiol,31 which was not detected by NMR spectroscopy as a short-lived isomer involved in the fast isomerization and cyclization. This suggestion is supported by the simultaneous occurrence of both Z-15-thione and product 10a in the reaction mixture.
Antitumor evaluation
Cytotoxic effects against breast cancer cells. The antiproliferative activity of some synthesized compounds was evaluated against the human estrogen-responsive breast cancer cell line MCF-7, as well as against the estrogen-independent breast cancer cell line MDA-MB-231, using the MTT assay. Cisplatin, a chemotherapy drug, was used as the reference compound. The corresponding inhibitory concentrations IC50 (IC50 is the half maximal inhibitory concentration) are enlisted in Table 2.
Table 2 Antiproliferative data for the synthesized steroidal pyridazines and the reference drug (cisplatin) against the human breast cancer cells (MCF-7 and MDA-MB-231)
Entry |
Comp. |
R1 |
R2 |
IC50, μM |
MCF-7 |
MDA-MB-231 |
1 |
4a |
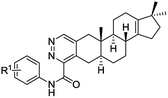 |
p-OMe |
— |
12.4 ± 1.1 |
NA |
2 |
4b |
p-Cl |
— |
15.8 ± 1.2 |
20.3 ± 1.3 |
3 |
4c |
p-CF3 |
— |
9.5 ± 0.7 |
20.6 ± 1.0 |
4 |
7a |
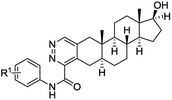 |
p-OMe |
— |
3.9 ± 0.7 |
7.7 ± 0.9 |
5 |
7b |
p-Cl |
— |
7.6 ± 0.9 |
12.8 ± 0.7 |
6 |
10a |
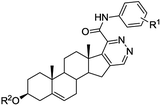 |
p-OMe |
Ac |
NA |
12.5 ± 0.7 |
7 |
10b |
p-Cl |
Ac |
5.1 ± 0.7 |
6.9 ± 0.8 |
8 |
10c |
p-CF3 |
Ac |
4.9 ± 0.8 |
NA |
9 |
10d |
H |
Ac |
6.5 ± 0.9 |
7.9 ± 0.7 |
10 |
10a′ |
p-OMe |
H |
NA |
NA |
11 |
10e′ |
m-OMe |
H |
NA |
NA |
12 |
10f′ |
p-F |
H |
NA |
NA |
13 |
11a |
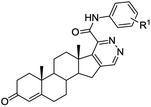 |
p-OMe |
— |
NA |
NA |
14 |
11f |
p-F |
— |
NA |
NA |
15 |
14e |
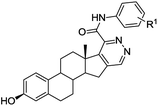 |
m-OMe |
— |
16.5 ± 0.9 |
NA |
16 |
|
Cisplatin |
|
|
6.2 ± 0.9 |
13.1 ± 1.1 |
For a SAR evaluation, the activity of the series of the newly synthesized compounds 4, 7, 10, 11 and 14 were evaluated against MCF-7 and MDA-MB-231 in order to investigate the effects of the pyridazine annulation to A/D-rings of the steroidal core and the halogen and methoxy substituents on the benzoyl moiety. The IC50 values of these compounds were determined as a measure of their relative cytotoxicity. Initially, A-ring modified androstane[3,2-d]pyridazines 4, 7 showed higher cytotoxic activity against both the MCF-7 and MDA-MB-231 cell lines as compared to D-ring annulated androstene[16,17-d]pyridazines and Δ1,3,5(10)-estratrieno[16,17-d]pyridazines 10, 11 and 14.
The estrogen-responsive breast cancer cell line MCF-7 was more sensitive to series of compounds 4 and 7. Among these series, 19-norandrostene 4b with R1 = Cl showed weak activity compared to the reference drug (Table 2, entry 2). However, after the replacement of chlorine with the methoxy substituent on the benzoyl moiety and of the 17,17′-dimethyl substituent with the hydroxyl group on the 18-methyl-17β-hydroxy moiety resulting in the formation of compound 7a, the latter showed the highest antiproliferative potency with IC50 of 3.9 ± 0.7 μM against the MCF-7 cell line, the IC50 value of compound 7a being 1.6 times smaller compared to cisplatin. Compounds 10b and 10c of the androstene[16,17-d]pyridazine series bearing a Cl and CF3 substituent on the benzoyl group exhibited higher antiproliferative activity (IC50 are 5.1 ± 0.7 μM and 4.9 ± 0.8 μM, respectively; see Table 2, entries 7 and 8) compared to the reference compound. Compound 10d containing the unsubstituted benzoyl moiety showed antiproliferative potency comparable to the drug cisplatin with the IC50 value of 6.5 ± 0.9 μM (Table 2, entry 8). It is remarkable that the structurally similar compounds 10a′, 10e′, 10f′ possessing a free hydroxyl group (Table 2, entries 10–12), as well as Δ4-3-oxo[17,16-d]pyridazines 11a, 11f (Table 2, entries 13, 14), were completely ineffective. Compound 14e from the Δ1,3,5(10)-estratrieno[16,17-d]pyridazine series was found to be weakly active towards MCF-7 cells with IC50 16.5 ± 0.9 μM (Table 2, entry 15).
The hormone-independent MDA-MB-231 cells were less sensitive to series of the newly synthesized compounds. Among the synthesized compounds, only seven chemicals showed toxicity against the MDA-MB-231 cells, whereas the other eight were not active. Chlorine-substituted androstene[16,17-d]pyridazine 10b proved to be the most active compound with IC50 (6.9 ± 0.8 μM, Table 2, entry 7) lower than that of cisplatin. However, A-ring modified androstan[3,2-d]pyridazine 7a with the methoxy substituent also exhibited higher antiproliferative activity compared to cisplatin (IC50 is 7.7 ± 0.9 μM, Table 2, entry 4).
It is noteworthy that compound 10a inhibited MDA-MB-231 cell growth, but was not active in MCF-7. On the contrary, compound 10c exhibited high activity in hormone-responsive MCF-7 and showed no effects in MDA-MB-231. Δ1,3,5(10)-Estratrieno[16,17-d]pyridazine 14e and 19-norandrostene 4a showing activities against MCF-7 did not exhibit any cytotoxic activity against the MDA-MB-231 cells.
Conclusions
To summarize, new types of heterosteroids, steroidal pyridazines, were prepared by the 6π-electrocyclization of highly reactive thiohydrazones of oxamic acid thiohydrazides. Native hormones 1, 5, 8 and 11 were transformed into the corresponding A- and D-annulated products in good/high yields (51–92%) using a two-step sequence involving the Vilsmeier–Haack reaction and imination with oxamic acid thiohydrazides. The mechanistic rationalization of the thiohydrazone isomerization equilibrium revealed the thiadiazoline form as an important intermediate. The evaluation of cytotoxic activity of the newly synthesized compounds against the breast cancer cell lines MCF-7 and MDA-MB-231 showed that compounds 7a and 10b, 10c, 10d displayed higher cytotoxicity than the reference drug. The IC50 values of compound 7a being 3.9 and 7.7 μM, which is about 1.6 times lower than that of the control cisplatin. Furthermore, the analysis of the structure–activity relationship revealed that the A-ring annulation is favorable for high cytotoxicity. Finally, this work provides evidence that steroidal pyridazines are promising lead compounds for developing novel and highly effective anticancer drugs.
Notes and references
-
(a) S. Kobayashi, Y. Mori, J. S. Fossey and M. M. Salter, Chem. Rev., 2011, 111, 2626–2704 CrossRef CAS PubMed;
(b) R. Lazny and A. Nodzewska, Chem. Rev., 2010, 110, 1386–1434 CrossRef CAS PubMed;
(c) P. Majumdar, A. Pati, M. Patra, R. K. Behera and A. K. Behera, Chem. Rev., 2014, 114, 2942–2977 CrossRef CAS PubMed.
-
(a) S. Gore, S. Baskaran and B. König, Org. Lett., 2012, 14, 4568–4571 CrossRef CAS PubMed;
(b) S. Wagaw, B. H. Yang and S. L. Buchwald, J. Am. Chem. Soc., 1998, 120, 6621–6622 CrossRef CAS.
- B. Du, C. Yuan, T. Yu, L. Yang, Y. Yang, B. Liu and S. Qin, Chem.–Eur. J., 2014, 20, 2613–2622 CrossRef CAS PubMed.
-
(a) A. Job, C. F. Janeck, W. Bettray, R. Peters and D. Enders, Tetrahedron, 2002, 58, 2253–2329 CrossRef CAS;
(b) K. Samment, C. Gastl, A. Baro, S. Laschat, P. Fischer and I. Fetting, Adv. Synth. Catal., 2010, 352, 2281–2290 CrossRef.
- A.-F. Li, H. He, Y.-B. Ruan, Z.-C. Wen, J.-S. Zhao, Q.-J. Jiang and Y.-B. Jiang, Org. Biomol. Chem., 2009, 7, 193–200 CAS.
-
(a) D.-H. Zhang, Z. Zhang and M. Shi, Chem. Commun., 2012, 48, 10271–10279 RSC;
(b) L. Hao, J.-J. Hong, J. Zhu and Z.-P. Zhan, Chem.–Eur. J., 2013, 19, 5715–5720 CrossRef CAS PubMed;
(c) Q. Sha, H. Liu and Y. Wei, Eur. J. Org. Chem., 2014, 7707–7715 CrossRef CAS.
-
(a) P.-X. Zhou, J.-Y. Luo, L.-B. Zhao, Y.-Y. Yea and Y.-M. Liang, Chem. Commun., 2013, 49, 3254–3256 RSC;
(b) P. Li, C. Wu, J. Zhao, D. C. Rogness and F. Shi, J. Org. Chem., 2012, 77, 3149–3158 CrossRef CAS PubMed.
- Z. Liu, L. Wang, H. Tan, S. Zhou, T. Fu, Y. Xia, Y. Zhang and J. Wang, Chem. Commun., 2014, 50, 5061–5063 RSC.
- Z. Zhang, Y. Liu, L. Ling, Y. Li, Y. Dong, M. Gong, X. Zhao, Y. Zhang and J. Wang, J. Am. Chem. Soc., 2011, 133, 4330–4341 CrossRef CAS PubMed.
- R. J. Billedeau, K. R. Klein, D. Kaplan and Y. Lou, Org. Lett., 2013, 15, 1421–1423 CrossRef CAS PubMed.
- A. Khanna, C. Maung, K. R. Johnson, T. T. Luong and D. L. Van Vranken, Org. Lett., 2012, 14, 3233–3235 CrossRef CAS PubMed.
- B. Chen, M. E. Scott, B. A. Adams, D. A. Hrovat, W. T. Borden and M. Lautens, Org. Lett., 2014, 16, 3930–3933 CrossRef CAS PubMed.
-
(a) D. Perdicchia and K. A. Jørgensen, J. Org. Chem., 2007, 72, 3565–3568 CrossRef CAS PubMed;
(b) S. Gogoi, C.-G. Zhao and D. Ding, Org. Lett., 2009, 11, 2249–2252 CrossRef CAS PubMed;
(c) A. Crespo-Pena, D. Monge, E. Martin-Zamora, E. Alvarez, R. Fernandez and J. M. Lassaletta, J. Am. Chem. Soc., 2012, 134, 12912–12915 CrossRef CAS PubMed;
(d) M. Fernandez, U. Uria, J. L. Vicario, E. Reyes and L. Carrillo, J. Am. Chem. Soc., 2012, 134, 11872–11875 CrossRef CAS PubMed.
- W. Wu, X. Yuan, J. Hu, X. Wu, Y. Wei, Z. Liu, J. Lu and J. Ye, Org. Lett., 2013, 15, 4524–4527 CrossRef CAS PubMed.
- S. Mao, Y.-R. Gao, X.-Q. Zhu, D.-D. Guo and Y.-Q. Wang, Org. Lett., 2015, 17, 1692–1695 CrossRef CAS PubMed.
- V. Sridharan, P. Ribelles, V. Estvez, M. Villacampa, M. T. Ramos, P. T. Perumal and J. C. Menndez, Chem.–Eur. J., 2012, 18, 5056–5063 CrossRef CAS PubMed.
- X. Xu, P. Y. Zavalij, W. Hu and M. P. Doyle, J. Org. Chem., 2013, 78, 1583–1588 CrossRef CAS PubMed.
-
(a) P. Mahalingam, K. Takrouri, T. Chen, R. Sahoo, E. Papadopoulos, L. Chen, G. Wagner, B. H. Aktas, J. A. Halperin and M. Chorev, J. Med. Chem., 2014, 57, 5094–5111 CrossRef CAS PubMed;
(b) V. N. Yarovenko, A. S. Nikitina, I. V. Zavarzin, M. M. Krayushkin and L. V. Kovalenko, Synthesis, 2006, 8, 1246–1248 CrossRef;
(c) I. V. Zavarzin, V. V. Chertkova, I. S. Levina and E. I. Chernoburova, Russ. Chem. Rev., 2011, 80, 661–682 CrossRef CAS;
(d) V. N. Yarovenko, A. A. Es'kov, I. V. Zavarzin, E. I. Chernoburova, A. Yu Martynkin and M. M. Krayuskin, Phosphorus, Sulfur Silicon Relat. Elem., 2003, 178, 1283–1288 CrossRef CAS;
(e) A. S. Shawali, M. A. Abdallah, I. M. Abbas and G. M. Eid, J. Chin. Chem. Soc., 2004, 51, 351–357 CrossRef CAS;
(f) H. Behbehani, H. M. Ibrahim and K. M. Dawood, RSC Adv., 2015, 5, 25642–25649 RSC.
-
(a) Y. Hu, C.-Y. Li, X.-M. Wang, Y.-H. Yang and H.-L. Zhu, Chem. Rev., 2014, 114, 5572–5610 CrossRef CAS PubMed;
(b) W. Thiel and R. Mayer, J. Prakt. Chem., 1989, 331, 649–658 CrossRef CAS;
(c) W. Thiel and R. Mayer, J. Prakt. Chem., 1990, 332, 55–64 CrossRef CAS;
(d) V. N. Yarovenko, A. V. Shirokov, I. V. Zavarzin, O. N. Krupinova, A. V. Ignatenko and M. M. Krayushkin, Synthesis, 2004, 1, 17–19 CrossRef.
- Z. Ding, Q. Tan, M. Gao and B. Xu, Org. Biomol. Chem., 2015, 13, 4642–4646 CAS.
- A. V. Komkov, A. S. Komendantova, L. G. Menchikov, E. I. Chernoburova, Y. A. Volkova and I. V. Zavarzin, Org. Lett., 2015, 17, 3734–3737 CrossRef CAS PubMed.
-
(a) A. Gupta, B. S. Kumar and A. S. Negi, J. Steroid Biochem. Mol. Biol., 2013, 137, 242–270 CrossRef CAS PubMed;
(b) A. Talbot, R. Maltais, L. C. Kenmogne, J. Roy and D. Poirier, Steroids, 2016, 107, 55–64 CrossRef CAS PubMed;
(c) B. Yu, X.-N. Sun, X.-J. Shi, P.-P. Qi, Y.-C. Zheng, D.-Q. Yu and H.-M. Liu, Steroids, 2015, 102, 92–100 CrossRef CAS PubMed.
- J. Szychowski, J.-F. Truchon and Y. L. Bennani, J. Med. Chem., 2014, 57, 9292–9308 CrossRef CAS PubMed.
-
(a) C. M. Yates, P. J. Brown, E. L. Stewart, C. Patten, R. J. H. Austin, J. A. Holt, J. M. Maglich, D. C. Angell, R. Z. Sasse, S. J. Taylor, I. J. Uings and R. P. Trump, J. Med. Chem., 2010, 53, 4531–4544 CrossRef CAS PubMed;
(b) R. M. Hoyte, J.-X. Zhang, R. Lerum, A. Oluyemi, P. Persaud, C. O'Connor, D. C. Labaree and R. B. Hochberg, J. Med. Chem., 2002, 45, 5397–5405 CrossRef CAS PubMed.
- D. Kovács, Z. Kádár, G. Mótyán, G. Schneider, J. Wölfling, I. Zupkó and É. Frank, Steroids, 2012, 77, 1075–1085 CrossRef PubMed.
- P. Carlini, A. Frassoldati, S. D. Marco, A. Casali, E. M. Ruggeri, M. Nardi, P. Papaldo, A. Fabi, F. Paoloni and F. Cognetti, Ann. Oncol., 2001, 12, 1539–1543 CrossRef CAS PubMed.
- S. Aggarwal, S. Thareja, A. Verma, T. R. Bhardwaj and M. Kumar, Steroids, 2010, 75, 109–153 CrossRef CAS PubMed.
-
(a) B. M. Trost and A. C. Gutierrez, Org. Lett., 2007, 9, 1473–1476 CrossRef CAS PubMed;
(b) E. M. Beccalli, F. Clerici and M. L. Gelmi, Tetrahedron, 2000, 56, 4817–4821 CrossRef CAS;
(c) T. Kleine, R. Frohlich, B. Wibbeling and E. Wurthwein, J. Org. Chem., 2011, 76, 4591–4599 CrossRef CAS PubMed;
(d) B. M. Trost and B. Biannic, Org. Lett., 2015, 17, 1433–1436 CrossRef CAS PubMed;
(e) D. A. Colby, R. G. Bergman and J. A. Ellman, J. Am. Chem. Soc., 2008, 130, 3645–3651 CrossRef CAS PubMed;
(f) C. Landreau, D. Deniaud, A. Reliquet, F. Reliquet and J. Meslin, J. Heterocycl. Chem., 2001, 38, 93–98 CrossRef CAS.
- The last step was confirmed experimentally, since sulfur was isolated in equimolar amounts in all reactions.
- V. N. Yarovenko, A. V. Shirokov, O. N. Krupinova, I. V. Zavarzin and M. M. Krayushkin, Russ. J. Org. Chem., 2003, 39, 1133–1139 CrossRef CAS.
- B. G. Milevsky, N. P. Solov'eva, T. A. Chibisova, V. N. Yarovenko, E. S. Zayakin, V. V. Chernyshev, M. M. Krayushkin and V. F. Traven, Russ. Chem. Bull., 2012, 61, 2311–2321 CrossRef CAS.
Footnote |
† Electronic supplementary information (ESI) available: Experimental details and characterization data for new compounds. For ESI see DOI: 10.1039/c6ra06881b |
|
This journal is © The Royal Society of Chemistry 2016 |