DOI:
10.1039/C6RA02908F
(Paper)
RSC Adv., 2016,
6, 24285-24289
p-Sulfonic acid calix[4]arene-functionalized alkyl-bridged organosilica in esterification reactions
Received
31st January 2016
, Accepted 25th February 2016
First published on 26th February 2016
Abstract
Two new p-sulfonic acid calix[4]arene- and p-sulfonic acid calix[6]arene-functionalized organosilica have been synthesized using a sol–gel method and applied as heterogeneous catalysts in esterification reactions. The catalytic performance was evaluated using the esterification of carboxylic acids with ethanol, and good catalytic activity (i.e., 55–88%) was observed under the optimum reaction conditions. This study reports the first promising example of the successful employment of calix[n]arenes as a heterogeneous catalyst for catalytic esterification. The catalyst was easily separated by filtration and reused five times without any significant loss of activity.
Introduction
Esters are among the most important functional groups in organic synthesis due to their usefulness.1 Esters have attracted more attention due to their wide applications in polymers, plastic derivatives, perfumes, flavours, chemicals, pharmaceutical and agrochemicals intermediates, and biodiesel.2
However, to develop an environmentally benign process and simplify the existing processes associated with homogeneous acid catalysts, substantial efforts have been focused on exploring heterogeneous solid acid catalysts, which offer significant advantages, such as easy recovery, little corrosion, low toxicity and environmental safety.3 Recently, the combination of the chemical properties of organo-sulfonic acid catalysts and the physical properties of different nanomaterials has been a subject of great interest in heterogeneous catalysis.3a,4
In last two decades, research interest in calix[n]arene chemistry has increased dramatically due to their applications in several fields of chemistry related to supramolecular chemistry including molecular recognition,5 self-assembling systems,6 mechanically interlocked molecules,7 and nanoporous materials.8 The application of calix[n]arenes as catalysts in organic transformations has become very popular.9 In addition, we have recently reported excellent results for the esterification of carboxylic acids using p-sulfonic acid calix[4]arene (CX4SO3H) or p-sulfonic acid calix[6]arene (CX6SO3H) as a homogeneous catalyst (Fig. 1).10 In this study, we report novel calix[n]arenes catalysts with Brønsted acid and heterogeneous properties as well as results from esterification reactions to synthesize carboxylic esters. To the best of our knowledge, this is the first application of p-sulfonic acid calix[n]arenes as heterogeneous catalysts in these esterification reactions.
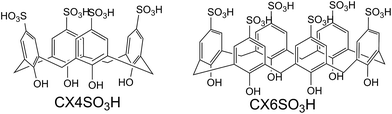 |
| Fig. 1 Molecular structures of the two catalysts: p-sulfonic acid calix[4]arene (CX4SO3H) and p-sulfonic acid calix[6]arene (CX6SO3H). | |
Results and discussion
To develop catalytic methodologies with easy recovery, little corrosion, low toxicity and environmental safety for the esterification of carboxylic acids, we designed an effective system using calix[n]arenes as a heterogeneous catalyst (Scheme 1).
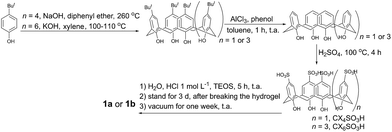 |
| Scheme 1 Synthesis of catalysts 1a and 1b. | |
p-Sulfonic acid calix[4]arene (CX4SO3H) and p-sulfonic acid calix[6]arene (CX6SO3H) were synthesized in our laboratory (Scheme 1) according to previously reported protocols.11 Catalysts 1a and 1b were synthesized using a sol–gel technique. A mixture of CX4SO3H or CX6SO3H, water and a hydrochloric acid solution was added to tetraethyl orthosilicate. The mixture was stirred for 5 h at room temperature. Then, the mixture was allowed to stand for 3 days, and after breaking the formed hydrogel with a Teflon stick, the sample was dried in a vacuum for one week at room temperature (Scheme 1 and Fig. 2). Catalysts 1a and 1b were characterized based on acidity and XRD.
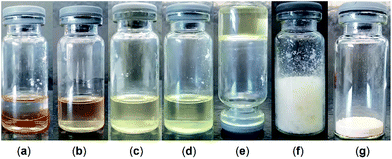 |
| Fig. 2 Formation of the 1a-based hydrogel induced by TEOS. (a) 0 h, (b) 1 h, (c) 24 h, (d) 72 h, (e) 72 h, (f) after breaking the formed hydrogel, and (g) hydrogel after washing and drying in a vacuum. | |
The ion exchange capacities of 1a were determined by acid–base titration and potentiometric titration. The acid capacity of the sample (catalyst 15%) was determined by titration with 5 × 10−3 M NaOH (aq).12 The result in terms of mmol H+ g−1 for the catalyst was 0.32 mmol H+ g−1 catalyst. The strength of the acid sites can be classified according to the following scale: E > 100 mV (very strong sites); 0 < E < 100 mV (strong sites); −100 < E < 0 mV (weak sites) and E < −100 mV (very weak sites).13 The acid strength for 15% catalyst was 205 mV, indicating the presence of very strong sites.
Typical XRD patterns of amorphous materials were observed for the supported 1a samples in Fig. 3. The XRD pattern peaks were obtained between 5° and 50°. The absence of crystalline 1a peaks in the XRD pattern of the supported samples cannot be ruled out because they may be less than 40 Å in size, which is beyond the detection limit of the XRD technique. The broad X-ray diffraction patterns may indicate long-range disordered nature of the silica.
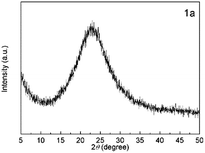 |
| Fig. 3 XRD patterns of catalyst 1a. | |
For the initial investigation of the use of 1a and 1b as catalysts for esterification, palmitic acid and ethanol were employed as substrates under the optimal reaction conditions (i.e., catalyst loading, time, and reuse of the catalyst). The results from the optimization of the catalyst concentration (2.0 mol%, 2.5 mol% and 5 mol%) are listed in Table 1. The evaluation of three concentrations of catalyst 1a indicated a slight increase in the conversion of palmitic acid to ethyl palmitate (Table 1, entries 1–3). For catalyst 1b, an increase in the catalyst concentration had a significant effect on the yield of ethyl palmitate (Table 1, entries 4–6). The differences observed between the catalytic activities of 1a and 1b may be due to the presence of water, which is a by-product of the esterification reaction.
Table 1 Effect of the amount of catalyst 1a or 1b and time on the conversion of ethyl palmitatea

|
Entry |
Catalyst |
Catalyst (mol%) |
Time (h) |
Yieldb (%) |
Reaction conditions: palmitic acid (100 mg) and ethanol (10 mL) was performed at 80 °C. Analysed by GC-MS and 1H NMR. |
1 |
1a |
2 |
4 |
88 |
2 |
1a |
2.5 |
4 |
91 |
3 |
1a |
5 |
4 |
91 |
4 |
1b |
2 |
4 |
67 |
5 |
1b |
2.5 |
4 |
78 |
6 |
1b |
5 |
4 |
87 |
7 |
1a |
2 |
6 |
90 |
8 |
1a |
2 |
8 |
93 |
As described by Fernandes et al. in 2014, p-sulfonic acid calix[n]arenes catalytic activity is affected by increasing the amount of water, and this effect was more pronounced for p-sulfonic acid calix[6]arene.10a Therefore, catalyst 1a exhibited the best conversion, and the concentration used for subsequent studies was 2 mol%. The effect of time on the conversion of palmitic acid to ethyl palmitate was also investigated (Table 1). The conversion of ethyl palmitate increased slightly from 88% to 90% and 93% with prolonged reaction times of 4 h, 6 h and 8 h (Table 1, entries 1, 7 and 8). Based on the conversion and selectivity results, a reasonable reaction time is 4 h.
The catalytic activities of the heterogeneous catalysts (1a and 1b) were compared to those of the CX4SO3H and CX6SO3H homogeneous catalysts (Table 2). The CX4SO3H and CX6SO3H homogeneous catalysts exhibited catalytic activities that were similar to that of 1a and higher than that of catalyst 1b (Table 2, entries 1–4). Similarly, both strong Brønsted acid heterogeneous Amberlyst 15 and H3PW12O40 exhibited a lower activity than the 1a catalyst, and the ethyl palmitate yield did not exceed 80% (Table 2, entries 5 and 6). The ethyl palmitate yield in the catalyst-free or matrix (TEOS) reactions was lower than 5% (Table 1, entries 7 and 8).
Table 2 Effect of different catalysts on the conversion of palmitic acid to ethyl palmitatea

|
Entry |
Catalyst |
Catalyst (mol%) |
Yieldc (%) |
Reaction conditions: palmitic acid (100 mg); ethanol (10 mL) was performed at 80 °C and time (4 h). Active phase (CX4SO3H or CX6SO3H). Analysed by GC-MS and 1H NMR. |
1 |
1a |
2b |
88 |
2 |
1b |
2b |
67 |
3 |
CX4SO3H |
2 |
91 |
4 |
CX6SO3H |
2 |
89 |
5 |
Amberlyst 15 |
2 |
79 |
6 |
H3PW12O40 |
2 |
67 |
7 |
TEOS |
2 |
<5 |
8 |
— |
2 |
<5 |
To determine if catalyst leaching occurred, an experiment using the optimal reaction conditions was performed for only 1 h. Then, the mixture was cooled, catalyst 1a was removed by filtration, and the conversion of palmitic acid was confirmed. The mixture was heated again at 80 °C for 3 h but no significant reaction progress was observed, demonstrating that leaching did not occur.
Once the optimal conditions for the formation of carboxylic esters using 1a as the catalyst were determined, the scope of this protocol was further investigated (Table 3). According to the data in Table 1, good to high yields were obtained in the esterification reactions of carboxylic acids in the presence of catalyst 1a. The carboxylic acids (Table 3) were converted to esters in yields ranging from 55–88% using catalyst 1a.
Table 3 Esters yields obtained in esterification reactions catalysed by 1aa

|
Entry |
Carboxylic acid |
Yieldb (%) |
Reaction conditions: carboxylic acid (0.40 mmol); ethanol (10 mL) and 1a (2 mol%) was performed at 80 °C and time (4 h). Analysed by GC-MS and 1H NMR. |
1 |
Palmitic acid |
88 |
2 |
Acetic acid |
83 |
3 |
Butyric acid |
86 |
4 |
Caprylic acid |
81 |
5 |
Oleic acid |
76 |
6 |
Stearic acid |
55 |
7 |
Myristic acid |
59 |
We also investigated the reuse of 1a in these reactions. After completing the reaction, the catalyst was easily separated by filtration, washed with dichloromethane and subsequently oven dried at 80 °C for 2 h prior to use in a subsequent reaction process. Once dried, the residue was used in successive reactions, and the yields were monitored. 1a was successfully used in five successive reactions without significant loss of catalytic activity (Fig. 4).
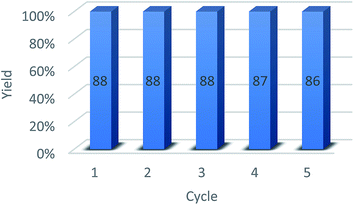 |
| Fig. 4 Reuse of 1a catalyst for the esterification of palmitic acid with ethanol. | |
Experimental
Chemicals and reagents
All of the chemicals were obtained from commercially available sources and used without further purification. The reactions did not require anhydrous conditions. The GC-MS analyses were carried out on a Shimadzu GC-2010 Plus gas chromatograph coupled with a MS QP2010 Ultra Shimadzu mass spectrometer (Tokyo, Japan), using a DB5 capillary column (30 m length, 0.25 mm id, 0.25 mm film thickness). The 1H- and 13C-NMR spectra were recorded on a Varian Mercury spectrometer at 300 MHz and 75 MHz, respectively.
General procedure for the synthesis of calix[n]arenes
CX4SO3H and CX6SO3H were synthesized in our laboratory according to previously published procedures. First, p-tert-butylcalix[4]arene and p-tert-butylcalix[6]arene were prepared using the Gutsche and Iqbal method.11a Second, p-tert-butylcalix[4]arene or p-tert-butylcalix[6]arene were dealkylated by treatment with aluminium chloride in the presence of toluene and phenol according to the method described by Gutsche and Lin.11b The preparation of CX4SO3H and CX6SO3H was carried out by treatment of calix[4]arene and calix[6]arene with concentrated sulfuric acid (98% wt) (Shinkai et al.).11c
General procedure for the synthesis of 1a and 1b
1a and 1b were synthesized using a sol–gel technique. A mixture of CX4SO3H (0.600 g) or CX6SO3H (0.500 g), water (4.5 mL or 3.7 mL, respectively) and 1 mol L−1 hydrochloric acid (0.5 mL or 0.4 mL, respectively) was added to tetraethyl orthosilicate (12.50 mL or 10.40 mL, respectively). The mixture was stirred for 5 h at room temperature. Then, the mixture was allowed to stand for 3 days, and after breaking the formed hydrogel with a Teflon stick, the sample was dried under vacuum for one week at room temperature. The powder was washed with distilled water (3 × 10 mL) and dried under vacuum.
Acidity in aqueous media
The acid capacity was determined by titration with 5 × 10−3 M NaOH (aq).12 In a typical experiment, 30 mg of solid 1a was added to 10 mL of deionized water. The resulting suspension was allowed to equilibrate and the titrated by dropwise addition of a 5 × 10−3 M NaOH solution using phenolphthalein (0.2%) as the pH indicator.
Acidity in non-aqueous media
The catalyst acidity of 1a (50 mg, catalyst 15%) was determined by potentiometric titration of a suspension consisting of the solid catalyst in acetonitrile using a solution containing n-butylamine in acetonitrile (0.025 N) in a Metrohm 794 Basic Titrino apparatus with a double junction electrode.13
X-ray diffraction
The crystallographic phase of supported 1a was analysed by ex situ XRD. The XRD patterns were collected using a Rigaku DMax 2500 PC instrument that was operated at 40 kV and 150 mA with Cu Kα (λ = 1.5406 Å) radiation. The data were collected in a 2θ range from 5° to 50° with a 0.5° divergence slit and a 0.3 mm receiving slit in fixed time mode with a 0.02° step size.
General procedure for the synthesis of esters
Carboxylic acid (0.40 mmol) and 2 mol% of the catalyst (CX4SO3H or CX6SO3H, active phase) were combined with ethanol (10 mL) in a 50 mL round-bottom flask equipped with a stir bar. The reaction was refluxed (80 °C) for the appropriate amount of time (4 h). After completion of the reaction, the reaction mixture was concentrated under vacuum to afford the crude product, which was analysed by GC-MS.
Conclusions
In conclusion, the results clearly demonstrate the possibility of preparing new p-sulfonic acid calix[4]arene heterogeneous catalyst 1a with a high catalytic activity for the esterification of carboxylic acids. The corresponding ester products were obtained in good to high yield (55–88%) with excellent selectivity. Some of the other advantages of catalyst 1a include easy product separation and purification, non-corrosive, and low cost. In addition, the catalyst is considered to be eco-friendly because it can be reused five times. The solid catalyst is highly specific and active, and its stability under liquid-phase reaction conditions indicates the potential for applications in a continuous flow reactor, which would further decrease the cost of ester production.
Acknowledgements
The authors acknowledge the financial support from the Coordenação de Aperfeiçoamento de Pessoal de Nível Superior (CAPES), Fundação de Amparo à Pesquisa do Estado de Minas Gerais (FAPEMIG), and Conselho Nacional de Desenvolvimento Científico e Tecnológico (CNPq). S. A. F. was supported by CNPq research fellowships.
Notes and references
- J. Otera, Esterification: methods, reactions and applications, Wiley-VCH Verlag GmbH &Co. KGaA, Weinheim, 1st edn, 2003 Search PubMed.
-
(a) A. C. Carmo, L. K. C. de Souza, C. E. F. de Costa, E. Longo, J. R. Zamian and G. N. Rocha Filha, Fuel, 2009, 88, 461 CrossRef CAS;
(b) R. S. Thombal, A. R. Jadhav and V. H. Jadhav, RSC Adv., 2015, 5, 12981 RSC;
(c) A. Orjuela, A. J. Yanez, A. Santhanakrishnan, C. T. Lira and D. J. Miller, Chem. Eng. J., 2012, 188, 98 CrossRef CAS.
-
(a) B. Karimi, H. M. Mirzaei, A. Mobarakia and H. Vali, Catal. Sci. Technol., 2015, 5, 3624 RSC;
(b) C. Grondal, M. Jeanty and D. Enders, Nat. Chem., 2010, 2, 167 CrossRef CAS PubMed;
(c) X. Jie, Y. Shang, P. Hu and W. Su, Angew. Chem., 2013, 125, 3718 CrossRef;
(d) J. B. Liu, L. Nie, H. Yan, L. H. Jiang, J. Weng and G. Lu, Org. Biomol. Chem., 2013, 11, 8014 RSC;
(e) Y. Peng, L. Luo, C. S. Yan, J. J. Zhang and Y. W. Wang, J. Org. Chem., 2013, 78, 10960 CrossRef CAS PubMed;
(f) C. K. Y. Lee, A. B. Holmes, S. V. Ley, I. F. McConvey, B. Al-Duri, G. A. Leeke, R. C. D. Santos and J. P. K. Seville, Chem. Commun., 2005, 2175 RSC;
(g) W. R. Reynolds, P. Plucinski and C. G. Frost, Catal. Sci. Technol., 2014, 4, 948 RSC;
(h) P. P. Giovannini, O. Bortolini, A. Cavazzini, R. Greco, G. Fantin and A. Massi, Green Chem., 2014, 16, 3904 RSC;
(i) J. Lim, S. S. Lee and J. Y. Ying, Chem. Commun., 2010, 46, 806 RSC;
(j) A. Gömann, J. A. Deverell, K. F. Munting, R. C. Jones, T. Rodemann, A. J. Canty, J. A. Smith and R. M. Guijt, Tetrahedron, 2009, 65, 1450 CrossRef.
-
(a) I. K. Mbaraka and B. H. Shanks, J. Catal., 2005, 229, 365 CrossRef CAS;
(b) I. K. Mbaraka and B. H. Shanks, J. Catal., 2006, 244, 78 CrossRef CAS;
(c) L. Sherry and J. A. Sullivan, Catal. Today, 2011, 175, 471 CrossRef CAS;
(d) P. L. Dhepe, M. Ohashi, S. Inagaki, M. Ichikawa and A. Fukuoka, Catal. Lett., 2005, 102, 163 CrossRef CAS;
(e) A. Karam, J. C. Alonso, T. I. Gerganova, P. Ferreira, N. Bion, J. Barrault and F. Jérôme, Chem. Commun., 2009, 7000 RSC.
-
(a) P. A. S. Abranches, E. V. V. Varejão, C. M. da Silva, A. de Fátima, T. F. F. Magalhães, D. L. da Silva, M. A. de Resende-Stoianoff, S. Reis, C. S. Nascimento Jr, W. B. de Almeida, I. M. Figueiredo and S. A. Fernandes, RSC Adv., 2015, 5, 44317 RSC;
(b) E. V. Varejão, A. de Fátima and S. A. Fernandes, Curr. Pharm. Des., 2013, 19, 6507 CrossRef;
(c) L. M. Arantes, E. V. V. Varejão, K. J. Pelizzaro-Rocha, C. M. S. Cereda, E. de Paula, M. P. Lourenço, H. A. Duarte and S. A. Fernandes, Chem. Biol. Drug Des., 2014, 83, 550 CrossRef CAS PubMed;
(d) J. V. de Assis, M. G. Teixeira, C. G. P. Soares, J. F. Lopes, G. S. L. Carvalho, M. C. S. Lourenço, M. V. de Almeida, W. B. de Almeida and S. A. Fernandes, Eur. J. Pharm. Sci., 2012, 47, 539 CrossRef CAS PubMed;
(e) D. L. da Silva, E. C. Tavares, L. S. Conegero, A. de Fátima, R. A. Pilli and S. A. Fernandes, J. Inclusion Phenom. Macrocyclic Chem., 2011, 69, 149 CrossRef.
-
(a) X. Yao, X. Wang, T. Jiang, X. Ma and H. Tian, Langmuir, 2015, 31, 13647 CrossRef CAS PubMed;
(b) F. Rodler, B. Schade, C. M. Jaeger, S. Backes, F. Hampel, C. Boettcher, T. Clark and A. Hirsch, J. Am. Chem. Soc., 2015, 137, 3308 CrossRef CAS PubMed;
(c) K. Kobayashi and M. Yamanaka, Chem. Soc. Rev., 2015, 44, 449 RSC.
-
(a) J. Matthias, R. Yuliya, M. Olena, M. Thorsten, M. Ingo, D. Gregor, E. M. Piotr, G. Juergen, B. Volker and J. Andreas, Nat. Nanotechnol., 2009, 4, 225 CrossRef PubMed;
(b) M. Zhang, X. Yan, F. Huang, Z. Niu and H. W. Gibson, Acc. Chem. Res., 2014, 47, 1995 CrossRef CAS PubMed.
-
(a) R. De Zorzi, N. Guidolin, L. Randaccio, R. Purrello and S. Geremia, J. Am. Chem. Soc., 2009, 131, 2487 CrossRef CAS PubMed;
(b) G. Brancatelli, R. De Zorzi, N. Hickey, P. Siega, G. Zingone and S. Geremia, Cryst. Growth Des., 2012, 12, 5111 CrossRef CAS.
-
(a) J. B. Simões, D. L. da Silva, A. de Fátima and S. A. Fernandes, Curr. Org. Chem., 2012, 16, 949 CrossRef;
(b) D. L. da Silva, B. S. Terra, M. R. Lage, A. L. T. G. Ruiz, C. C. da Silva, J. E. de Carvalho, J. W. M. Carneiro, F. T. Martins, S. A. Fernades and A. de Fátima, Org. Biomol. Chem., 2015, 13, 3280 RSC;
(c) J. B. Simões, A. de Fátima, A. A. Sabino, L. C. A. Barbosa and S. A. Fernandes, RSC Adv., 2014, 4, 18612 RSC;
(d) J. B. Simões, A. de Fátima, A. A. Sabino, F. J. T. de Aquino, D. L. da Silva, L. C. A. Barbosa and S. A. Fernandes, Org. Biomol. Chem., 2013, 11, 5069 RSC;
(e) D. L. da Silva, S. A. Fernandes, A. A. Sabino and A. de Fátima, Tetrahedron Lett., 2011, 52, 6328 CrossRef CAS;
(f) A. G. Sathicq, N. A. Liberto, S. A. Fernandes and G. P. Romanelli, C. R. Chim., 2015, 18, 374 CrossRef CAS;
(g) D. M. Homden and C. Redshaw, Chem. Rev., 2008, 108, 5086 CrossRef CAS PubMed.
-
(a) R. Natalino, E. V. V. Varejão, M. J. da Silva, A. L. Cardoso and S. A. Fernandes, Catal. Sci. Technol., 2014, 4, 1369 RSC;
(b) S. A. Fernandes, R. Natalino, M. J. da Silva and C. F. Lima, Catal. Commun., 2012, 26, 127 CrossRef CAS;
(c) S. A. Fernandes, R. Natalino, P. A. R. Gazolla, M. J. da Silva and G. N. Jham, Tetrahedron Lett., 2012, 53, 1630 CrossRef CAS.
-
(a) C. D. Gutsche and M. Iqbal, Org. Synth., 1990, 68, 234 CrossRef CAS;
(b) C. D. Gutsche and L. G. Lin, Tetrahedron, 1986, 42, 1633 CrossRef CAS;
(c) S. Shinkai, S. Mori, T. Tsubaki, T. Sone and O. Manabe, Tetrahedron Lett., 1984, 25, 5315 CrossRef CAS.
- J. J. Martínez, E. Nope, H. Rojas, M. H. Brijaldo, F. Passos and G. Romanelli, J. Mol. Catal. A: Chem., 2014, 392, 235 CrossRef.
- S. G. Casuscelli, M. E. Crivello, C. F. Perez, G. Ghione, E. R. Herrero, L. R. Pizzio, P. G. Vázquez, C. V. Cáceres and M. N. Blanco, Appl. Catal., A, 2004, 274, 115 CrossRef CAS.
|
This journal is © The Royal Society of Chemistry 2016 |