DOI:
10.1039/C6RA02556K
(Paper)
RSC Adv., 2016,
6, 24484-24490
Synthesis and characterization of N-heterocyclic carbene-palladium(II) chlorides-1-methylindazole and -1-methylpyrazole complexes and their catalytic activity toward C–N coupling of aryl chlorides†
Received
28th January 2016
, Accepted 25th February 2016
First published on 26th February 2016
Abstract
A series of N-heterocyclic carbene-palladium(II) chlorides-1-methylindazole and -1-methylpyrazole complexes was successfully synthesized and fully characterized by X-ray single crystal diffraction. In addition, initial investigations of their catalytic activity showed that they were efficient catalysts in the C–N coupling of primary and secondary amines with aryl chlorides at low catalyst loadings.
Introduction
Aryl amines are very important compounds in organic synthesis because of their potential applications in chemistry, pharmaceuticals, materials sciences and industries.1 Besides the abundant traditional methods for the synthesis of such compounds,2 the palladium-catalysed C–N cross-coupling reactions have become one of the most powerful methods due to their high efficiency of diverse substrates under mild conditions.3 Among the organic electrophiles involved, aryl chlorides seem to be the most desirable ones due to their lower cost, easy availability and higher stability compared to their iodide and bromide counterparts in spite of their lower reactivity.4 To achieve efficient coupling of aryl chlorides, kinds of tertiary phosphine ligands, which are usually air- and thermal-sensitive, have been synthesized to date.5 On the other hand, during the past years, N-heterocyclic carbenes (NHCs), which usually are air- and thermal-insensitive compared to phosphine ligands, have also been developed and successfully applied in the palladium-catalysed C–N coupling of aryl chlorides.6 It may be noted here that in the palladium–phosphine catalytic systems, usually excess ligands over palladium salts are mandatory; while different from free phosphine ligands, NHCs are usually used as their palladium complexes, which thus have strict NHC/Pd ratio. In addition, the palladium salts and NHCs are in the same molecule, both of which can be added simultaneously, thus will also simplify the experimental procedures to some extent. However, their applications toward ideal practicality are still hampered by at least one of the following facts: (1) lengthy pathways are necessary for the synthesis of NHC precursors and the corresponding palladium complexes, especially for the synthesis of NHC precursors; (2) toxic pyridine derivatives are used as the solvents and ancillary ligands; (3) high catalyst loadings are required (usually 1.0–5.0 mol%). Therefore, great room still remains for the NHC–Pd(II) complexes-catalysed C–N coupling of aryl chlorides, especially with respect to the easy availability of the complexes and the lower loadings in catalysis.
Recently, we have developed a series of novel NHC–Pd(II)-2-aryl-4,5-dihydro-oxazole complexes from commercially available imidazolium salts such as IPr·HCl [1,3-bis-(2,6-diisopropylphenyl)imidazolium chloride], IMes·HCl [1,3-bis-(2,4,6-trimethylphenyl)imidazolium chloride], and IXy·HCl [1,3-bis-(2,6-dimethylphenyl)imidazolium chloride], PdCl2 and 2-aryl-4,5-dihydrooxazoles in a one-pot procedure under mild conditions and have showed that they were efficient catalysts in the C–N coupling of primary and secondary amines with aryl chlorides at the catalyst loading of 0.5 mol%.6c The reported method includes the advantages of easy availability of the NHC–Pd(II) complexes and high efficiency in C–N coupling of aryl chlorides. These results thus prompted us to further develop new NHC–Pd(II) complexes and investigate their applications toward C–N coupling of aryl chlorides. In this case, a new series of NHC–Pd(II)-1-methylindazole and -1-methylpyrazole complexes was successfully obtained in good to high yields, and they demonstrated efficient catalytic activity toward C–N coupling of primary and secondary amines with aryl chlorides at lower catalyst loadings to 0.03 mol%. Herein, we report these results in detail.
Results and discussion
Synthesis of NHC–Pd(II) complexes
The NHC–Pd(II) complexes were achieved by slightly modifying our previous process.6c In the general one-pot procedure, a mixture of commercially available imidazolium salts 1 (1.1 mmol), PdCl2 (1.0 mmol), 1-methylindazole 2a or 1-methylpyrazole 2b and K2CO3 (1.04 mmol) in THF (3.0 mL) was stirred at 80 °C for 18 h. After normal flash chromatography by SiO2 column, pure products 3 can then be obtained in acceptable to high yields (Table 1).
Table 1 Synthesis of NHC–Pd(II) complexes 3

|
Entrya |
1 (R) |
2 (equiv.) |
Yieldb (%) |
All reactions were carried out using 1 (1.1 mmol), PdCl2 (1.0 mmol), 2 and K2CO3 (1.04 mmol) in THF (3.0 mL) at 80 °C for 18 h. Isolated yields. |
1 |
1a (2,6-iPr2) |
2a (2.0) |
3a, 51 |
2 |
1a |
2a (3.0) |
3a, 76 |
3 |
1a |
2b (3.0) |
3b, 25 |
4 |
1a |
2b (4.0) |
3b, 91 |
5 |
1b (2,6-Me2) |
2b (3.0) |
3c, 9 |
6 |
1b |
2b (4.0) |
3c, 83 |
7 |
1c (2,4,6-Me3) |
2b (3.0) |
3d, 21 |
8 |
1c |
2b (4.0) |
3d, 70 |
All these four complexes were fully characterized by NMR, MS, and elemental analysis. In addition, all of them are stable enough in open air under ambient conditions. Crystals of 3a–d were grown in the mixture of ethyl acetate and dichloromethane, which were then characterized by X-ray single crystal diffraction analysis. The molecular structures of them are shown in Fig. 1–4, including representative bond lengths and bond angles, and selected crystallographic data of them are shown in Table 2. As expected, all complexes showed slightly distorted square-planar geometries around all palladium centers, which are coordinated by four ligands such as one carbene carbon atom, one N atom and two chlorine atoms, with the carbene carbon atom and N atom in the trans-configuration. The two chlorine atoms are almost perpendicular to the plane of NHC and 1-methylindazole or 1-methylpyrazole.
 |
| Fig. 1 The molecular structure of complex 3a showing 30% probability ellipsoids; all hydrogen atoms are omitted for clarity. Selected bond lengths (Å) and bond angles (°): Pd(1)–C(1) = 1.9680(19), Pd(1)–N(3) = 2.0862(16), Pd(1)–Cl(2) = 2.2892(5), Pd(1)–Cl(1) = 2.2938(5), N(1)–C(1) = 1.358(2), N(2)–C(1) = 1.348(2); C(1)–Pd(1)–N(3) = 177.25(7), C(1)–Pd(1)–Cl(2) = 90.15(5), N(3)–Pd(1)–Cl(2) = 90.06(5), C(1)–Pd(1)–Cl(1) = 90.55(5), N(3)–Pd(1)–Cl(1) = 89.27(5), Cl(2)–Pd(1)–Cl(1) = 178.975(19). | |
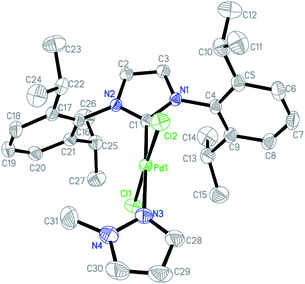 |
| Fig. 2 The molecular structure of complex 3b showing 30% probability ellipsoids; all hydrogen atoms are omitted for clarity. Selected bond lengths (Å) and bond angles (°): Pd(1)–C(1) = 1.972(3), Pd(1)–N(3) = 2.093(3), Pd(1)–Cl(2) = 2.2938(10), Pd(1)–Cl(1) = 2.3020(10), N(1)–C(1) = 1.349(4), N(2)–C(1) = 1.352(4); C(1)–Pd(1)–N(3) = 178.06(13), C(1)–Pd(1)–Cl(2) = 88.69(9), N(3)–Pd(1)–Cl(2) = 89.84(10), C(1)–Pd(1)–Cl(1) = 92.82(9), N(3)–Pd(1)–Cl(1) = 88.68(10), Cl(2)–Pd(1)–Cl(1) = 178.18(4). | |
 |
| Fig. 3 The molecular structure of complex 3c showing 30% probability ellipsoids; all hydrogen atoms are omitted for clarity. Selected bond lengths (Å) and bond angles (°): Pd(1)–C(1) = 1.968(3), Pd(1)–N(3) = 2.088(3), Pd(1)–Cl(2) = 2.2948(11), Pd(1)–Cl(1) = 2.3178(11), N(1)–C(1) = 1.367(4), N(2)–C(1) = 1.358(4); C(1)–Pd(1)–N(3) = 173.78(11), C(1)–Pd(1)–Cl(2) = 89.40(12), N(3)–Pd(1)–Cl(2) = 88.60(8), C(1)–Pd(1)–Cl(1) = 91.73(11), N(3)–Pd(1)–Cl(1) = 90.84(8), Cl(2)–Pd(1)–Cl(1) = 174.30(4). | |
 |
| Fig. 4 The molecular structure of complex 3d showing 30% probability ellipsoids; all hydrogen atoms are omitted for clarity. Selected bond lengths (Å) and bond angles (°): Pd(1)–C(1) = 1.972(4), Pd(1)–N(3) = 2.089(3), Pd(1)–Cl(1) = 2.2931(13), Pd(1)–Cl(2) = 2.3048(13), N(1)–C(1) = 1.352(5), N(2)–C(1) = 1.349(5); C(1)–Pd(1)–N(3) = 177.35(16), C(1)–Pd(1)–Cl(1) = 92.65(11), N(3)–Pd(1)–Cl(1) = 89.06(12), C(1)–Pd(1)–Cl(2) = 88.96(11), N(3)–Pd(1)–Cl(2) = 89.40(12), Cl(1)–Pd(1)–Cl(2) = 177.57(5). | |
Table 2 Crystal data and structure refinement details for complexes 3a–d
Complex |
3a |
3b |
3c |
3d |
Formula |
C35H44Cl2N4Pd |
C31H42Cl2N4Pd·CH3CO2Et·H2O |
C23H26Cl2N4Pd |
C25H30Cl2N4Pd |
Formula weight |
698.04 |
754.11 |
535.78 |
563.83 |
Temperature of measurement (K) |
100(2) |
100(2) |
100(2) |
100(2) |
Crystal system |
Monoclinic |
Monoclinic |
Triclinic |
Monoclinic |
Space group |
P21/C |
P21/n |
P![[1 with combining macron]](https://www.rsc.org/images/entities/char_0031_0304.gif) |
P21/n |
a (Å) |
10.5287(11) |
15.909(3) |
9.1155(18) |
16.484(4) |
b (Å) |
12.2427(12) |
14.083(2) |
13.036(3) |
16.074(4) |
c (Å) |
26.839(3) |
17.085(3) |
20.075(4) |
21.788(6) |
α (°) |
90 |
90 |
87.103(4) |
90 |
β (°) |
94.179(2) |
92.674(3) |
89.823(4) |
109.467(4) |
γ (°) |
90 |
90 |
89.630(4) |
90 |
Volume (Å3) |
3450.3(6) |
3823.4(10) |
2382.4(9) |
5443(2) |
Z |
4 |
4 |
4 |
8 |
Crystal size (mm) |
0.29 × 0.25 × 0.22 |
0.29 × 0.23 × 0.21 |
0.31 × 0.25 × 0.22 |
0.32 × 0.26 × 0.23 |
F(000) |
1448 |
1576 |
1088 |
2034 |
Theta range for data collection (°) |
1.52–26.00 |
1.71–26.00 |
1.02–26.00 |
1.35–26.00 |
Goodness-of-fit on F2 |
1.008 |
1.107 |
1.047 |
1.086 |
Final R indices [I > 2σ(I)] |
R1 = 0.0228, wR2 = 0.0737 |
R1 = 0.0418, wR2 = 0.1325 |
R1 = 0.0321, wR2 = 0.0890 |
R1 = 0.0464, wR2 = 0.1399 |
R indices (all data) |
R1 = 0.0250, wR2 = 0.0824 |
R1 = 0.0505, wR2 = 0.1428 |
R1 = 0.0456, wR2 = 0.1006 |
R1 = 0.0712, wR2 = 0.1626 |
C–N coupling reactions
Once the structures of the above NHC–Pd(II) complexes 3 were fully characterized, their activity toward C–N coupling of aryl chlorides was then investigated systematically. The representative results are illustrated in Table 3. Initially, using morpholine 4a (1.2 mmol) and chlorobenzene 5a (1.0 mmol) as the substrates, 3a (0.1 mol%) as the catalyst, a variety of bases (1.3 equiv.) were tested in THF (1.0 mL) at 90 °C for 1 h. In these cases, KOtBu gave the best yield (93%) (Table 3, entry 1) over NaOtBu (84%) and KOH (20%). In the presence of other bases such as LiOtBu, NaOH, K2CO3, Na2CO3, KHCO3, Cs2CO3, K3PO4 and CH3COOK, almost no desired product can be detected. In the presence of KOtBu, some common solvents were tested, and the same yield was obtained in toluene (Table 3, entry 2), while in polar solvents such as DMF and DMSO, very low yields were observed in both cases (4% and 16%, respectively). Based on the above results, the catalytic activity of complex 3a was further tested in decreased loading of 0.05 mol% using KOtBu as the base in THF and toluene, respectively. It was found that by elevating the temperature to 110 °C, somewhat higher yield can still be achieved in THF within 1 h (Table 3, entry 4), while that in toluene became very low (Table 3, entry 5). Upon further decreasing the catalyst loading to 0.03 mol%, similar high yield can still be achieved when the reaction was performed in THF at 130 °C for 12 h (Table 3, entry 7). Based on the above results, other NHC–Pd(II) complexes 3 were also examined (Table 3, entries 10–12). For example, in the presence of NHC–Pd(II) complex 3b, product 6a can be obtained in 85% yield (Table 3, entry 10); however, very low yields were observed when complexes 3c and 3d were used as the catalysts under identical conditions (Table 3, entries 11 and 12).
Table 3 Optimization for the C–N coupling reaction

|
Entrya |
3 (X) |
Temp. (°C) |
Time (h) |
Yieldb (%) |
Otherwise specified, all reactions were carried out using 4a (1.2 mmol), 5a (1.0 mmol), 3 (X mol%), KOtBu (1.3 equiv.) in THF (1.0 mL). Isolated yields. Toluene instead of THF. |
1 |
3a (0.1) |
90 |
1 |
93 |
2c |
3a (0.1) |
90 |
1 |
93 |
3 |
3a (0.05) |
100 |
1 |
67 |
4 |
3a (0.05) |
110 |
1 |
67 |
5c |
3a (0.05) |
110 |
1 |
6 |
6 |
3a (0.03) |
110 |
12 |
69 |
7 |
3a (0.03) |
130 |
12 |
93 |
8 |
3a (0.01) |
110 |
12 |
22 |
9 |
3a (0.01) |
130 |
12 |
37 |
10 |
3b (0.05) |
110 |
1 |
87 |
11 |
3c (0.05) |
110 |
1 |
5 |
12 |
3d (0.05) |
110 |
1 |
15 |
With the optimal conditions established, the generality and limitation of the reactions between a variety of secondary amines and aryl chlorides was first tested. The results are shown in Table 4. Most reactions performed well enough in the presence of 0.05 mol% 3a at 110 °C within 1 h or 0.03 mol% 3a at 130 °C within 12 h, giving the corresponding coupling products 6 in good to almost quantitative yields. Diverse electronic and sterically-hindered substituents on the aryl chlorides are all tolerated in such transformation. For instance, electron-rich groups such as methoxy and methyl group and electron-poor group such as fluorine atom are all tolerated to give the corresponding products in good to almost quantitative yields. Sterically-hindered substituted 2-methylphenyl chloride 5d was also a suitable substrate to give products 6d, 6k and 6p in 83–99% yields (Table 4, entries 4, 5, 14 and 22). It seems that the relative position of the same substituents on the phenyl groups of aryl chlorides affected the reactions to some extent. For example, meta-substituted aryl chlorides are better than para-ones, giving higher yields in all cases (Table 4, entries 1 vs. 3, 6 vs. 8 and 10 vs. 9). To our pleasure, heterocyclic chloride such as 2-chloropyridine 5j was also a good substrate, giving products 6s–6u in good to high yields (Table 4, entries 25–27). In addition, these three reactions were also tested in the presence of PEPPSI6af under identical conditions, similar or a litter lower yields were observed in these cases.
Table 4 NHC–Pd(II) complex 3a-catalysed C–N coupling of secondary amines with aryl chlorides

|
Entrya |
4 |
5 |
Conditions |
Yieldb (%) |
Otherwise specified, the reaction conditions were as follows: 4 (0.84 mmol), 5 (0.7 mmol), KOtBu (1.3 equiv.), THF (1.0 mL) and (A) 3a (0.05 mol%), 110 °C, 1 h or (B) 3a (0.03 mol%), 130 °C, 12 h. Isolated yields. The time was prolonged to 3 h. The time was prolonged to 6 h and the yields in the parathesis were obtained catalysed by PEPPSI (ref. 6af). |
1 |
4a |
 |
5b (3-OMe) |
|
A |
6b, 95 |
2 |
4a |
5b |
B |
6b, 90 |
3 |
4a |
5c (4-OMe) |
A |
6c, 84 |
4 |
4a |
5d (2-Me) |
A |
6d, 91 |
5 |
4a |
5d |
B |
6d, 94 |
6 |
4a |
5e (3-Me) |
A |
6e, 94 |
7 |
4a |
5e |
B |
6e, 88 |
8c |
4a |
5f (4-Me) |
A |
6f, 89 |
9 |
4a |
5g (4F) |
A |
6g, 81 |
10 |
4a |
5h (3F) |
A |
6h, 93 |
11 |
4a |
5h |
B |
6h, 82 |
12 |
4a |
5i (4-vinyl) |
A |
6i, 86 |
13 |
4b |
 |
5b |
B |
6j, 95 |
14 |
4b |
5d |
B |
6k, 99 |
15 |
4b |
5e |
B |
6l, 86 |
16 |
4c |
 |
5b |
A |
6m, 90 |
17 |
4c |
5b |
B |
6m, 96 |
18 |
4c |
5e |
A |
6n, 80 |
19 |
4c |
5e |
B |
6n, 96 |
20 |
4d |
 |
5b |
A |
6o, 91 |
21 |
4d |
5b |
B |
6o, 99 |
22 |
4d |
5d |
B |
6p, 83 |
23 |
4d |
5e |
B |
6q, 99 |
24 |
4d |
5f |
B |
6r, 99 |
25d |
4a |
|
5j |
 |
A |
6s, 96 (89) |
26d |
4c |
|
5j |
A |
6t, 80 (77) |
27d |
4d |
|
5j |
A |
6u, 85 (85) |
Usually, primary amines were thought to be more poor organonucleophiles in the palladium-catalysed C–N coupling reactions. Based on the above satisfactory results on the NHC–Pd(II) complex 3a catalysed C–N coupling between secondary amines and aryl chlorides, kinds of primary amines were also subjected to the optimal conditions to test the generality and limitations. The results are shown in Table 5. To our delight, it was found that the desired mono-arylated products 8 can also be achieved in good to almost quantitative yields under identical conditions. For instance, electron-rich, -poor and sterically-hindered groups substituted anilines are all tolerated to give the corresponding products in good to almost quantitative yields. The amination reactions between sterically-encumbered substrates seem to be a challenge in the previously reported methods, especially at low catalyst loadings. However, to our delight, both anilines and aryl chlorides bearing sterically-hindered substituents are suitable substrates under identical conditions. For example, 2-methylaniline 7b, 2-methoxyaniline 7c, 2,4-dimethylaniline 7e, 2,4,6-trimethylaniline 7f, 2,6-dimethylaniline 7g and 2,6-di-isopropylaniline 7i are all good substrates under the optimal conditions. In addition, 2-methylphenyl chloride 5d, 2,6-dimethylphenyl chloride 5k and 2,6-di-isopropylphenyl chloride 5l are all compatible.
Table 5 NHC–Pd(II) complex 3a-catalysed C–N coupling of primary amines with aryl chlorides

|
Entrya |
7 (R′) |
5 |
Conditions |
Yieldb (%) |
Otherwise specified, the reaction condition were as follows: 7 (0.84 mmol), 5 (0.7 mmol), KOtBu (1.3 equiv.), THF (1.0 mL) and (A) 3a (0.05 mol%), 110 °C, 1 h or (B) 3a (0.03 mol%), 130 °C, 12 h. Isolated yields. The time was prolonged to 3 h. |
1c |
7a (H) |
5d (2-Me) |
A |
8a, 98 |
2 |
7b (2-Me) |
5d |
A |
8b, 98 |
3c |
7c (2-OMe) |
5d |
A |
8c, 99 |
4 |
7c |
5d |
B |
8c, 98 |
5 |
7d (4-Me) |
5d |
A |
8d, 98 |
6 |
7d (4-Me) |
5d |
B |
8d, 98 |
7 |
7e (2,4-Me2) |
5d |
A |
8e, 98 |
8 |
7e |
5d |
B |
8e, 98 |
9 |
7f (2,4,6-Me3) |
5d |
A |
8f, 98 |
10 |
7g (2,6-Me2) |
5d |
A |
8g, 98 |
11 |
7g |
5d |
B |
8g, 98 |
12 |
7h (4-OMe) |
5d |
A |
8h, 98 |
13c |
7i (2,6-iPr2) |
5d |
A |
8i, 92 |
14 |
7j (4-F) |
5d |
A |
8j, 84 |
15 |
7j |
5d |
B |
8j, 98 |
16 |
7b |
5k (2,6-Me2) |
A |
8g, 97 |
17 |
7f |
5k |
A |
8k, 96 |
18 |
7g |
5k |
A |
8l, 97 |
19c |
7f |
5l (2,6-iPr2) |
A |
8m, 95 |
To make the complex more practicable in organic synthesis, the reactions of alkyl amine such as cyclohexylamine with aryl chlorides were also investigated under the optimal conditions. It was found that all reactions performed well enough to give the desired mono-arylated amines 9 in good to high yields (Scheme 1).
 |
| Scheme 1 Complex 3a catalysed coupling of cyclohexylamine with aryl chlorides. | |
In addition, gram-scale reactions between morpholine (42 mmol) and aryl chlorides (35 mmol) were also carried out in the presence of KOtBu (1.3 equiv.) and complex 3a (0.03 mol%) in THF (10.0 mL) at 130 °C for 12 h, also giving the corresponding coupling products in high yields in both cases (Scheme 2).
 |
| Scheme 2 Complex 3a catalysed gram-scale reactions. | |
Experimental
General procedures
NMR spectra were recorded at 500 MHz (for 1H NMR) or 125 MHz (for 13C NMR), respectively. 1H and 13C NMR spectra recorded in CDCl3 solutions were referenced to TMS (0.00 ppm) and the residual solvent peak (77.0 ppm), respectively. J values are given in Hz. The organic solvents used were dried by standard methods. The mass analyzer type for the high resolution mass spectra (HRMS) is quadrupole (for ESI). All amines were distilled prior to using. Other commercially obtained reagents were used without further purification. Flash column chromatography was performed on silica gel (300–400 mesh). X-ray single crystallography was performed on a Bruker APEX II area-detector graphite-monochromated Mo Kα radiation (λ = 0.71073 Å). The crystal data collection and refinement parameters are summarized in Table 2. Absorption correction was performed by SADABS (Bruker, 2002) program. The structures were solved by direct methods using the SHELXS-97 program and refined by full-matrix least squares techniques on F2.
General procedure for the synthesis of NHC–Pd(II) complexes 3
Under a N2 atmosphere, a mixture of imidazolium salts 1 (1.1 mmol), PdCl2 (1.0 mmol), K2CO3 (1.04 mmol), the indicated amounts of 2 (Table 1) and anhydrous THF (3.0 mL) in a sealed tube was stirred at 80 °C for 18 h. Then the solvent was removed under reduced pressure, and the residue was purified by flash chromatography on silica gel (eluent: petroleum ether/ethyl acetate = 60
:
1 to 10
:
1) to give the pure NHC–Pd(II) complexes 3 as yellow solids.
Compound 3a. Yellow solid. mp 287 °C (decomposed). 1H NMR (CDCl3, 500 MHz, TMS) δ 7.82 (s, 1H), 7.56–7.52 (m, 3H), 7.40 (d, J = 8.0 Hz, 4H), 7.28 (d, J = 7.5 Hz, 1H), 7.17–7.15 (m, 3H), 7.02 (t, J = 7.5 Hz, 1H), 3.97 (s, 3H), 3.19 (hept, J = 6.5 Hz, 4H), 1.48 (d, J = 6.5 Hz, 12H), 1.12 (d, J = 6.5 Hz, 12H). 13C NMR (CDCl3, 125 MHz) δ 154.9, 146.9, 140.3, 135.1, 134.6, 130.2, 127.4, 125.0, 123.9, 122.9, 121.11, 121.08, 108.8, 36.0, 28.8, 26.4, 23.0. MS (ESI): 661 [M − Cl]+. HRMS (ESI) calcd for C35H44ClN4Pd [M − Cl]+: 661.2294; found: 661.2281. Anal. calcd for C35H44Cl2N4Pd: C, 60.22%; H, 6.53%; N, 8.03%; found: C, 60.09%; H, 6.24%; N, 8.04%. IR (neat) ν 2960, 2925, 2860, 1618, 1506, 1464, 1441, 1413, 1379, 1351, 1329, 1211, 1155, 1121, 1056, 1031, 972, 944, 911, 816, 802, 783 cm−1.
Compound 3b. Yellow solid. mp 295 °C (decomposed). 1H NMR (CDCl3, 500 MHz, TMS) δ 7.51 (t, J = 7.5 Hz, 2H), 7.37 (d, J = 8.0 Hz, 4H), 7.27 (d, J = 2.5 Hz, 1H), 7.13 (s, 2H), 7.11 (d, J = 2.5 Hz, 1H), 6.04 (t, J = 2.5 Hz, 1H), 3.80 (s, 3H), 3.17 (hept, J = 7.0 Hz, 4H), 1.46 (d, J = 7.0 Hz, 12H), 1.10 (d, J = 7.0 Hz, 12H). 13C NMR (CDCl3, 125 MHz) δ 155.2, 146.9, 140.6, 135.1, 132.1, 130.2, 124.9, 123.9, 106.3, 39.4, 28.8, 26.3, 23.0. MS (ESI): 611 [M − Cl]+. HRMS (ESI): calcd for C31H42ClN4Pd [M − Cl]+: 611.2136; found: 611.2154. Anal. calcd for C31H42Cl2N4Pd: C, 57.46; H, 6.53; N, 8.65. Found: C, 57.50; H, 6.61; N, 8.71. IR (neat) ν 2960, 2921, 2866, 1522, 1468, 1457, 1411, 1382, 1346, 1330, 1284, 1208, 1120, 1105, 1083, 1060, 937, 889, 801, 764 cm−1.
Compound 3c. Yellow solid. mp 287 °C (decomposed). 1H NMR (CDCl3, 500 MHz, TMS) δ 7.36 (t, J = 7.5 Hz, 2H), 7.27 (s, 2H), 7.25 (s, 2H), 7.24 (dd, J = 2.5, 0.5 Hz, 1H), 7.14–7.13 (m, 3H), 6.05 (t, J = 2.5 Hz, 1H), 3.74 (s, 3H), 2.41 (s, 12H). 13C NMR (CDCl3, 125 MHz) δ 150.3, 140.5, 137.5, 137.0, 132.2, 129.4, 128.4, 123.9, 106.4, 39.2, 19.2. HRMS (ESI): calcd for C23H26ClN4Pd [M − Cl]+, 499.0811; found, 499.0899. Anal. calcd for C23H26Cl2N4Pd: C, 51.56; H, 4.89; N, 10.46. Found: C, 51.60; H, 4.98; N, 10.26. MS (ESI): 499 [M − Cl]+. HRMS (ESI) calcd for C23H26ClN4Pd [M − Cl]+: 499.0881; found: 499.0899. IR (neat) ν 2963, 1523, 1475, 1406, 1361, 1331, 1285, 1261, 1224, 1159, 1098, 1019, 945, 866, 797, 779, 760 cm−1.
Compound 3d. Yellow solid. mp 291 °C (decomposed). 1H NMR (CDCl3, 500 MHz, TMS) δ 7.26 (d, J = 2.5 Hz, 1H), 7.14 (d, J = 2.5 Hz, 1H), 7.08 (s, 2H), 7.06 (s, 4H), 6.05 (t, J = 2.5 Hz, 1H), 3.78 (s, 3H), 2.39 (s, 6H), 2.35 (s, 12H). 13C NMR (CDCl3, 125 MHz) δ 153.1, 140.5, 139.1, 136.6, 135.1, 132.1, 129.1, 124.0, 106.3, 39.2, 21.1, 19.1. MS (ESI): 527 [M − Cl]+. HRMS (ESI): calcd for C25H30ClN4Pd [M − Cl]+, 527.1214; found, 527.1195. Anal. calcd for C25H30Cl2N4Pd: C, 53.25; H, 5.36; N, 9.94. Found: C, 52.99; H, 5.32; N, 9.83. IR (neat) ν 3523, 2968, 2921, 2855, 1609, 1520, 1486, 1438, 1425, 1410, 1374, 1338, 1281, 1261, 1224, 1162, 1101, 1076, 1039, 992, 928, 868, 853, 801, 750 cm−1.
General procedure for the NHC–Pd(II) complex 3a catalysed C–N coupling reactions
Under a N2 atmosphere, NHC–Pd(II) complex 3a (0.05 or 0.03 mol%), KOtBu (1.3 equiv.), THF (1.0 mL), amines (0.84 mmol) and aryl chlorides (0.7 mmol) were successively added into a sealed tube. The mixture was stirred vigorously at the indicated temperature and specified time shown in Tables 3–5. Then the solvent was removed under reduced pressure and the residue was purified by flash column chromatography (SiO2) to give the pure products.
Conclusions
In conclusion, from easily available starting materials such as NHC·HCl, PdCl2 and 1-methylindazole and 1-methylpyrazole, a new type of NHC–PdCl2 complexes was obtained in acceptable to high yields in a one-pot procedure under mild conditions, and the structures of all of them were unambiguously determined by X-ray single crystal diffraction. Their catalytic activity was initially tested by the C–N coupling between secondary and primary amines with aryl chlorides. It was found that low catalyst loadings such as 0.03 mol% are sufficient for such transformation. Compared to other well-defined NHC–Pd(II) complexes such as PEPPSI-type complexes, they have at least one of the following advantages such as easy availability, lower cost and lower toxicity of the ancillary ligands, and small amounts of them in the synthesis of the complexes, easy synthesis, and lower catalyst loading. Catalytic activities of these complexes in other transformations are underway in this laboratory.
Acknowledgements
Financial support from the National Natural Science Foundation of China (No. 21471115) is greatly appreciated. Quan Zhou thanks the University Students' Innovative Undertaking Training Program for financial support.
Notes and references
-
(a) S. Suwanprasop, T. Nhujak, S. Roengsumran and A. Petsom, Ind. Eng. Chem. Res., 2004, 43, 4973 CrossRef CAS;
(b) Amines: Synthesis, Properties and Application, ed. S. A. Lawrence, Cambridge University Press, Cambridge, 2004 Search PubMed;
(c) D. O'Hagan, Nat. Prod. Rep., 2000, 17, 435 RSC;
(d) A. Belfield, G. R. Brown and A. J. Foubister, Tetrahedron, 1999, 55, 11399 CrossRef CAS;
(e) Industrial Organic Chemistry, ed. K. Weissermel and H. J. Arpe, Wiley-VCH, Weinheim, Germany, 1997 Search PubMed;
(f) P. N. Craig, in Comprehensive Medicinal Chemistry, ed. C. J. Drayton, Pergamon Press, New York, 1991, vol. 8 Search PubMed.
-
(a) March's Advanced Organic Chemistry: Reactions, Mechanisms and Structure, ed. M. B. Smith and J. March, John Wiley & Sons Inc., New York, 5th ed, 2001 Search PubMed;
(b) H. Heaney, Chem. Rev., 1962, 62, 81 CrossRef CAS.
- For some recent selected reviews, please see:
(a) J. Bariwal and E. Van der Eycken, Chem. Soc. Rev., 2013, 42, 9283 RSC;
(b) R. J. Lundgren and M. Stradiotto, Chem.–Eur. J., 2012, 18, 9758 CrossRef CAS PubMed;
(c) D. S. Surry and S. L. Buchwald, Angew. Chem., Int. Ed., 2008, 47, 6338 CrossRef CAS PubMed;
(d) J. F. Hartwig, Acc. Chem. Res., 2008, 41, 1534 CrossRef CAS PubMed.
-
(a) R. B. Bedford, C. S. J. Cazin and D. Holder, Coord. Chem. Rev., 2004, 248, 2283 CrossRef CAS;
(b) A. F. Littke and G. C. Fu, Angew. Chem., Int. Ed., 2002, 41, 4176 CrossRef CAS;
(c) V. V. Grushin and H. Alper, in Activation of Unreactive Bonds and Organic Synthesis, ed. S. Murai, Springer, Berlin, 1999, pp. 193–226 Search PubMed;
(d) V. V. Grushin and H. Alper, Chem. Rev., 1994, 94, 1047 CrossRef CAS.
- For some representative examples, please see:
(a) S. M. Wong, O. Y. Yuen, P. Y. Choy and F. Y. Kwong, Coord. Chem. Rev., 2015, 293–294, 158 CrossRef CAS;
(b) S. M. Wong, C. M. So and F. Y. Kwong, Synlett, 2012, 23, 1132 CrossRef CAS;
(c) K. H. Chung, C. M. So, S. M. Wong, C. H. Luk, Z.-Y. Zhou, C. P. Lau and F. Y. Kwong, Synlett, 2012, 23, 1181 CrossRef CAS;
(d) S. Rodriguez, B. Qu, N. Haddad, D. C. Reeves, W.-J. Tang, H. Lee, D. Krishnamurthy and C. H. Senanayake, Adv. Synth. Catal., 2011, 353, 533 CrossRef CAS;
(e) R. J. Lundgren, A. Sappong-Kumankumah and M. Stradiotto, Chem.–Eur. J., 2010, 16, 1983 CrossRef CAS PubMed;
(f) G.-S. Chen, W. H. Lam, W. S. Fok, H. W. Lee and F. Y. Kwong, Chem.–Asian J., 2007, 2, 306 CrossRef CAS PubMed;
(g) L. Ackermann, J. H. Spatz, C. J. Gschrei, R. Born and A. Althammer, Angew. Chem., Int. Ed., 2006, 45, 7627 CrossRef CAS PubMed;
(h) D. Liu, W.-Z. Gao, Q. Dai and X.-M. Zhang, Org. Lett., 2005, 7, 4907 CrossRef CAS PubMed;
(i) F. Rataboul, A. Zapf, R. Jackstell, S. Harkal, T. Riermeier, A. Monsees, U. Dingerdissen and M. Beller, Chem.–Eur. J., 2004, 10, 2983 CrossRef CAS PubMed;
(j) S. Urgaonkar, M. Nagarajan and J. G. Verkade, Org. Lett., 2003, 5, 815 CrossRef CAS PubMed;
(k) R. A. Singer, S. Caron, R. E. McDermott, P. Arpin and N. M. Do, Synthesis, 2003, 1727 CrossRef CAS;
(l) N. Kataoka, Q. Shelby, J. P. Stambuli and J. F. Hartwig, J. Org. Chem., 2002, 67, 5553 CrossRef CAS PubMed;
(m) G. Y. Li, G. Zheng and A. F. Andrew, J. Org. Chem., 2001, 66, 8677 CrossRef CAS PubMed;
(n) J. P. Wolfe, H. Tomori, J. P. Sadighi, J.-J. Yin and S. L. Buchwald, J. Org. Chem., 2000, 65, 1158 CrossRef CAS PubMed.
-
(a) Y. Zhang, V. César and G. Lavigne, Eur. J. Org. Chem., 2015, 2042 CrossRef CAS;
(b) S. Sharif, R. P. Rucker, N. Chandrasoma, D. Mitchell, M. J. Rodriguez, R. D. J. Froese and M. G. Organ, Angew. Chem., Int. Ed., 2015, 54, 9507 CrossRef CAS PubMed;
(c) P. Huang, Y.-X. Wang, H.-F. Yu and J.-M. Lu, Organometallics, 2014, 33, 1587 CrossRef CAS;
(d) J. Yang, P.-H. Li, Y.-C. Zhang and L. Wang, Dalton Trans., 2014, 43, 14114 RSC;
(e) J. Yang, P.-H. Li, Y.-C. Zhang and L. Wang, J. Organomet. Chem., 2014, 766, 73 CrossRef CAS;
(f) J. L. Krinsky, A. Martínez, C. Godard, S. Castillón and C. Claver, Adv. Synth. Catal., 2014, 356, 460 CrossRef CAS;
(g) Y. Zhang, V. César, G. Storch, N. Lugan and G. Lavigne, Angew. Chem., Int. Ed., 2014, 53, 6482 CrossRef CAS PubMed;
(h) G. Bastug and S. P. Nolan, Organometallics, 2014, 33, 1253 CrossRef CAS;
(i) M. Pompeo, J. L. Farmer, R. D. J. Froese and M. G. Organ, Angew. Chem., Int. Ed., 2014, 53, 3223 CrossRef CAS PubMed;
(j) W.-W. Fang, J. Jiang, Y. Xu, J.-F. Zhou and T. Tu, Tetrahedron, 2013, 69, 673 CrossRef CAS;
(k) S. Meiries, G. Le Duc, A. Chartoire, A. Collado, K. Speck, K. S. Athukorala Arachchige, A. M. Z. Slawin and S. P. Nolan, Chem.–Eur. J., 2013, 19, 17358 CrossRef CAS PubMed;
(l) G. Le Duc, S. Meiries and S. P. Nolan, Organometallics, 2013, 32, 7547 CrossRef CAS;
(m) A. R. Martin, Y. Makida, S. Meiries, A. M. Z. Slawin and S. P. Nolan, Organometallics, 2013, 32, 6265 CrossRef CAS;
(n) S. Meiries, K. Speck, D. B. Cordes, A. M. Slawin and S. P. Nolan, Organometallics, 2013, 32, 330 CrossRef CAS;
(o) A. Chaetoire, A. Boreux, A. R. Martin and S. P. Nolan, RSC Adv., 2013, 3, 3840 RSC;
(p) K. H. Hoi, J. A. Coggan and M. G. Organ, Chem.–Eur. J., 2013, 19, 843 CrossRef CAS PubMed;
(q) W.-X. Chen and L.-X. Shao, J. Org. Chem., 2012, 77, 9236 CrossRef CAS PubMed;
(r) L. Zhu, Y.-M. Ye and L.-X. Shao, Tetrahedron, 2012, 68, 2414 CrossRef CAS;
(s) S. Meireis, A. Chaartoire, A. M. Z. Slawin and S. P. Nolan, Organometallics, 2012, 31, 3402 CrossRef;
(t) A. Chartoire, X. Frogneux, A. Boreux, A. M. Z. Slawin and S. P. Nolan, Organometallics, 2012, 31, 6947 CrossRef CAS;
(u) A. Chartoire, X. Frogneux and S. P. Nolan, Adv. Synth. Catal., 2012, 354, 1897 CrossRef CAS;
(v) K. H. Hoi, S. Çalimsiz, R. D. J. Froese, A. C. Hopkinson and M. G. Organ, Chem.–Eur. J., 2012, 18, 145 CrossRef CAS PubMed;
(w) K. H. Hoi and M. G. Organ, Chem.–Eur. J., 2012, 18, 804 CrossRef CAS PubMed;
(x) L. Zhu, T.-T. Gao and L.-X. Shao, Tetrahedron, 2011, 67, 5150 CrossRef CAS;
(y) T. Tu, W.-W. Fang and J. Jiang, Chem. Commun., 2011, 47, 12358 RSC;
(z) M.-T. Chen, D. A. Vicic, W. J. Chain, M. L. Turner and O. Navarro, Organometallics, 2011, 30, 6770 CrossRef CAS;
(a
a) M.-T. Chen, D. A. Vicic, M. L. Turner and O. Navarro, Organometallics, 2011, 30, 5052 CrossRef CAS;
(a
b) K. H. Hoi, S. Çalimsiz, R. D. J. Froese, A. C. Hopkinson and M. G. Organ, Chem.–Eur. J., 2011, 17, 3086 CrossRef CAS PubMed;
(a
c) N. Marion, O. Navarro, E. C. Ecarnot, A. Bell, D. Amoroso and S. P. Nolan, Chem.–Asian J., 2010, 5, 841 CrossRef CAS PubMed;
(a
d) Z. Jin, S.-X. Guo, X.-P. Gu, L.-L. Qiu, H.-B. Song and J.-X. Fang, Adv. Synth. Catal., 2009, 351, 1575 CrossRef CAS;
(a
e) O. H. Winkelmann, A. Riekstins, S. P. Nolan and O. Navarro, Organometallics, 2009, 28, 5809 CrossRef CAS;
(a
f) M. G. Organ, M. Abdel-Hadi, S. Avola, I. Dubovyk, N. Hadei, E. A. B. Kantchev, C. J. O'Brien, M. Sayah and C. Valente, Chem.–Eur. J., 2008, 14, 2443 CrossRef CAS PubMed;
(a
g) N. Marion, O. Navarro, J.-G. Mei, E. D. Stevens, N. M. Scott and S. P. Nolan, J. Am. Chem. Soc., 2006, 128, 4101 CrossRef CAS PubMed.
Footnote |
† Electronic supplementary information (ESI) available. CCDC references numbers 1445502–1445505 for complexes 3a–d, respectively. For ESI and crystallographic data in CIF or other electronic format see DOI: 10.1039/c6ra02556k |
|
This journal is © The Royal Society of Chemistry 2016 |