DOI:
10.1039/C6RA01242F
(Communication)
RSC Adv., 2016,
6, 33031-33035
An ICT-based colorimetric and ratiometric fluorescent probe for hydrogen sulfide and its application in live cell imaging†
Received
15th January 2016
, Accepted 26th March 2016
First published on 29th March 2016
Abstract
A new naphthalimide-based fluorescent probe for the detection of hydrogen sulfide has been designed and developed. The probe exhibits high selectivity and sensitivity, with efficient colorimetric and ratiometric response for H2S under mild conditions. Furthermore, the probe was successfully used for fluorescence imaging of H2S in living cells.
Hydrogen sulfide (H2S), traditionally viewed as a toxic gas with an unpleasant odor of rotten eggs, has recently become known as the third endogenously produced gasotransmitter after nitric oxide (NO) and carbon monoxide (CO).1 Endogenous H2S is generated predominantly from cysteine or its derivatives by cystathionine-γ-lyase (CSE), cystathionine-β-synthase (CBS), cysteine aminotransferase (CAT), and 3-mercaptopyruvate sulfurtransferase (MST), in the cytosols and mitochondria of mammalian cells.2 Physiological levels of H2S appear to be involved in various important physiological processes, including relaxation of vascular smooth muscles, intervention of neurotransmission, and regulation of inflammation and vasodilation.3 On the other hand, the abnormal production of H2S has been linked to a variety of disease phenotypes such as Alzheimer's disease, Down's syndrome, hypertension, diabetes, and liver cirrhosis.4 Despite numerous reports, many details on the physiological roles of H2S are still under active investigation. Therefore, it is important to develop accurate tools for H2S detection and measurement in its native environments to facilitate detailed biological function studies.
Conventional techniques used for quantifying H2S, such as methylene blue method, monobromobimane method, electro chemical methods, colorimetric methods, and gas chromatography, often need complicated sample preparation and/or tissue or cell destruction, and are therefore not suitable for real-time and in situ analysis.5 Thus, current research has been focused on fluorescent methods for their ease of application in solution as well as their noninvasive and real-time detection capability in vivo.6
Since Chang's group reported the first-generation of fluorescent probe for H2S in 2011, a number of highly innovative H2S-probes have been developed based on the reduction of azides, nitros or hydroxylamine to amines mediated by H2S, or the nucleophilic attack of H2S, even the precipitation of metal sulfides.7 However, most of the reported probes responding to H2S with changes only in fluorescence intensity (based on the “Off–On” or “On–Off” mode), are significantly influenced by the excitation/emission efficiency, the sample environments, and the probe location, which may potentially pose problems for use in quantitative detection.8 In contrast, ratiometric fluorescent measurement, which uses the ratio of two fluorescent bands instead of the absolute intensity of one band for quantification, provides the practical advantage of built-in variability correction.9 Therefore, the development of highly sensitive and selective ratiometric fluorescent probe for the accurate detection of H2S is still in demand.
Naphthalimide dye is a well-known chromophore exhibiting strong intramolecular charge transfer (ICT) due to “push–pull” substituents, which results in a large spectral shift.10 To date, a number of naphthalimide-based probes have been developed for different analytes through the modulation of ICT efficiency (Fig. 1).7o,r,s,11 Inspired by these works, we report here a new naphthalimide-based colorimetric and ratiometric fluorescent probe, NS1, which is an azido phenyl derivative of 4-hydroxynaphthalimide, for the detection of H2S. Based on the specific reduction of azide by H2S to generate the corresponding amine,7a–d an azide group was selected as the reaction site, linked by p-aminobenzyl alcohol, a typical self-immolative linker.12 We envisioned that modifying the 4-hydroxyl donor into a more electron-withdrawing benzyl ether group that could be specifically decaged by H2S back to the hydroxyl would affect both ICT and emission color, and thereby resulted in colorimetric and ratiometric response in both color and fluorescence.
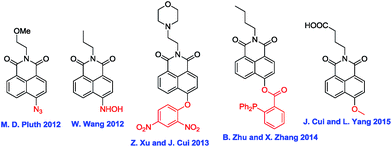 |
| Fig. 1 Some reported naphthalimide-based probes. | |
As shown in Scheme 2, NS1 can be readily prepared in three convenient steps under facile conditions with good yields starting with commercially available 4-bromo-1,8-naphthalic anhydride. The probe (NS1) was well characterized by 1H, 13C NMR, as well as HR-MS (ESI†).
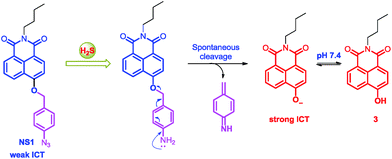 |
| Scheme 1 Colorimetric and ratiometric sensing of H2S by NS1. | |
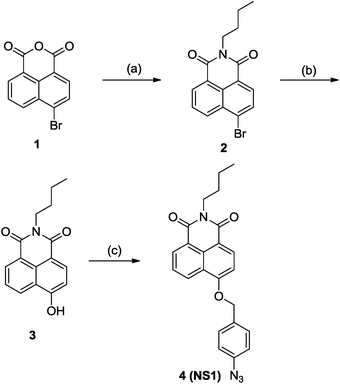 |
| Scheme 2 Synthesis of NS1: (a) n-butylamine, ethanol, reflux, 8 h, 93%; (b) (i) NaOMe, MeOH, reflux, 12 h; (ii) HI (57%), H2O, reflux, 12 h, 65% over two steps; (c) 1-azido-4-(chloromethyl)benzene, K2CO3, acetone, reflux, 12 h, 72%. | |
With NS1 in hand, we firstly assessed the UV-vis spectroscopic properties of NS1 in HEPES buffer solution (10 mM, pH 7.4, containing 50% EtOH). NS1 (10.0 μM) displayed a moderate UV-vis absorption around 370 nm.
Upon incremental addition of NaHS (0–200.0 equiv.), the peak at 370 nm dramatically decreased, and a new band at 455 nm, which is characteristic for the 4-hydroxynaphthalimide fluorophore, appeared instantly with a clear isosbestic point at 403 nm (red-shift about 85 nm with a marked color change from colorless to light yellow), indicating that compound 3 was formed in the present of NaHS (Fig. 2 and S1, ESI†). Furthermore, a good linear relationship (R2 = 0.993) was observed between the changes in the ratios of absorbance at 455 and 370 nm (A455 nm/A370 nm) with NaHS in the range of 0–60.0 equiv. (Fig. S2, ESI†).
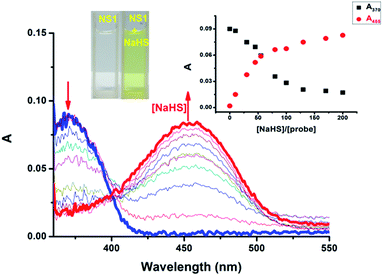 |
| Fig. 2 Absorption spectra of NS1 (10.0 μM) in HEPES buffer solution (10 mM, pH 7.4, containing 50% EtOH) in the presence of different concentrations of NaHS (0–200.0 equiv.). Inset 1: the absorbance at 370 nm and 455 nm as a function of NaHS concentration. Inset 2: cuvette images of probe NS1 before and after addition of NaHS. | |
The emission spectra of NS1 and its fluorescent titration with NaHS were recorded in HEPES buffer solution (10 mM, pH 7.4, containing 50% EtOH) (Fig. 3 and S3, ESI†). As expected, NS1 alone is blue-fluorescent (λex = 410 nm, λem = 444 nm, Φ = 0.11, Table S1, ESI†) due to the weak intramolecular charge transfer effect from 4-benzyl ether group to 1,8-diamide (Scheme 1). However, upon progressive addition of NaHS (0–200.0 equiv.), the emission band at 444 nm rapidly decreased and a new emission band at 539 nm appeared instantly with a clear isostilbic point at 493 nm (red-shift about 95 nm with an obvious emission color change from blue to yellowish-green), which is attributed to the cleavage of p-azidobenzyl ether group by the H2S-induced reduction of the azido moiety to the corresponding amine followed by spontaneous 1,6-elimination reaction to form compound 3 with an electron-donating hydroxyl group (Fig. 3 and Scheme 1). Moreover, the fluorescence titration curve revealed that the fluorescence intensity ratios of NS1 at 539 nm and 444 nm (I539 nm/I444 nm) increased linearly with increasing concentrations of NaHS in the range of 0–80 equiv. (Fig. S4, ESI†). Based on these results, the detection limit of NS1 for NaHS was calculated to be 1.7 × 10−6 M.
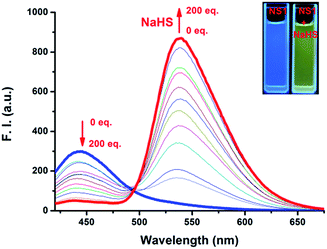 |
| Fig. 3 Fluorescence spectra of NS1 (10.0 μM) in HEPES buffer solution (10 mM, pH 7.4, containing 50% EtOH) in the presence of different concentrations of NaHS (0–200.0 equiv.) (λex = 410 nm). Inset: cuvette images of probe NS1 before and after addition of NaHS taken under a hand-held UV-lamp (λex = 365 nm). | |
The plausible mechanism of the H2S-induced fluorescence response was shown in Scheme 1. Efforts were then made to explore the nature of the H2S-induced response. To this end, a comparison of fluorescent spectra between the NS1–NaHS system and compound 3 was made to confirm the generation of 3 after treatment with H2S (Fig. S5, ESI†).
Moreover, 1H NMR titration experiment was also conducted to clarify the actual NS1/NaHS interaction. As shown in Fig. S6, ESI,† the five aromatic protons attributed to naphthalimide (H-2, H-3, H-5, H-6, and H-7) were all shifted upfield by 0.2–1.2 ppm due to the formation of an electron-rich hydroxyl group at position 4 of naphthalimide. On the other hand, the two methylene protons at δ 5.48 ppm attributed to the H-7′ and the four aromatic protons (two AB quartets) at δ 7.21 (2H, J = 8.0 Hz, H-2′ and H-6′) and 7.63 (2H, J = 8.0 Hz, H-3′ and H-5′) assigned to the p-azidobenzyl ether moiety were also obviously shifted upfield due to the formation of (4-aminophenyl)methanethiol after the addition of NaHS (Fig. S6, and Scheme S2, ESI†). These results agree well with the optical responses.
Subsequently, the time-dependence of the fluorescence was also evaluated in the presence of H2S in HEPES buffer solution (10 mM, pH 7.4, containing 50% EtOH) (Fig. 4 and S7, ESI†). The results showed that the ratios of fluorescent intensity at 444 nm and 539 nm (I444 nm/I539 nm) remarkably decreased to the minimum value within 90 minutes (Fig. S7, ESI†). Accordingly, the observed rate constant (kobs) for the formation of compound 3 has been calculated to be 1.8 × 10−4 min−1 (Fig. 4, ESI†).
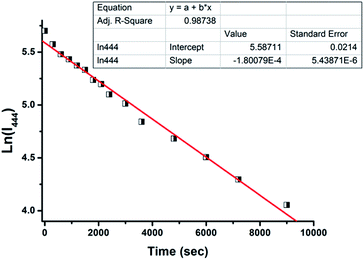 |
| Fig. 4 Kinetic plot of fluorescence emission intensity at 444 nm of the pseudo-first order reaction of 10.0 μM NS1 to 1 mM NaHS (HEPES buffer solution, 10 mM, pH 7.4, containing 50% EtOH) (λex = 410 nm). | |
Continuing on, the fluorescence titration of NS1 with various reactive sulfur species and coexisting ions was conducted to examine the selectivity (Fig. 5 and S8–S10, ESI†). Much to our delight, the tested various sulfur species (GSH, Cys, HSO3−, S2O42−, S2O32−, SO32−) and the examined common coexisting ions (ClO−, I−, Cu2+, Fe3+) showed nominal changes to the fluorescence spectra of NS1. It should be mentioned that NS1 still responded to H2S sensitively even in the presence of GSH and Cys (Fig. 5 and S10, ESI†). Therefore, these results suggest that NS1 displays high selectivity toward H2S in neutral aqueous solution.
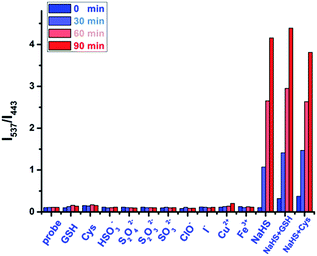 |
| Fig. 5 Fluorescence responses of NS1 (10.0 μM) to various reactive sulfur species and coexisting ions (including GSH, Cys, HSO3−, S2O42−, S2O32−, SO32−, ClO−, I−, Cu2+, Fe3+, and NaHS) at 1 mM. Bars represent emission intensity ratios I539 nm/I444 nm at 0, 30, 60, and 90 min after addition of each analyte in HEPES buffer solution (10 mM, pH 7.4, containing 50% EtOH) (λex = 410 nm). | |
Moreover, the H2S-sensing ability of NS1 at a wide range of pH values was investigated. As depicted in Fig. S11, ESI,† NS1 alone was inert to pH in the range of 3.2–10.5. On the other hand, it readily responded to H2S within the biologically relevant pH range (6.2–10.5). These results indicate that NS1 could be used in living cells without interference from pH effects.
Due to the favorable properties of NS1 in vitro, the potential utility of NS1 in living cells was studied. MCF-7 cells were incubated with 5.0 μM of NS1 for 20 min at 37 °C and only weak green fluorescence was observed (Fig. 6a). The cells were then treated with NaHS (100, and 200 μM, respectively) for 30 min at 37 °C, which resulted in a dramatic increase of intracellular fluorescence (Fig. 6b and c). These obvious changes indicated that NS1 was cell membrane permeable and capable of imaging H2S in living cells.
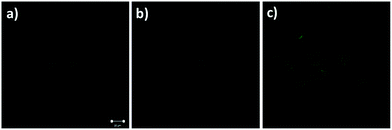 |
| Fig. 6 Fluorescence image of MCF-7 cells incubated with NS1 (5.0 μM) for 20 min, and then washed quickly with PBS for imaging (a). The cells were then treated with NaHS (100, and 200 μM, respectively) for 30 min which resulted in a dramatic increase in intracellular fluorescence (b), and (c), respectively. The cells were observed under confocal microscopy (ZEISS LSM780) with excitation at 405 nm and emission at 507–601 nm. | |
In conclusion, we have rationally developed a new naphthalimide-based fluorescent probe for the detection of hydrogen sulfide. The probe displayed a specific colorimetric and ratiometric fluorescence response towards H2S under mild conditions. Furthermore, fluorescence imaging of H2S in living cells indicated that this probe might be favorable for biological applications.
Acknowledgements
This work was supported by the National Natural Science Foundation of China (20902082), the Project Grants 521 Talents Cultivation of Zhejiang Sci-Tech University, and the Program for Innovative Research Team of Zhejiang Sci-Tech University (13060052-Y).
Notes and references
-
(a) Y. Han, J. Qin, X. Chang, Z. Yang and J. Du, Cell. Mol. Neurobiol., 2006, 26, 101 CrossRef PubMed;
(b) R. Wang, Antioxid. Redox Signaling, 2003, 5, 493 CrossRef CAS PubMed;
(c) L. Li, P. Rose and P. K. Moore, Annu. Rev. Pharmacol. Toxicol., 2011, 51, 169 CrossRef CAS PubMed.
-
(a) G. A. Benavides, G. L. Squadrito, R. W. Mills, H. D. Patel, T. S. Isbell, R. P. Patel, V. M. Darley-Usmar, J. E. Doeller and D. W. Kraus, Proc. Natl. Acad. Sci. U. S. A., 2007, 104, 17977 CrossRef CAS PubMed;
(b) M. Ishigami, K. Hiraki, K. Y. Umemura, O. K. Ishii and H. Kimura, Antioxid. Redox Signaling, 2009, 11, 205 CrossRef CAS PubMed;
(c) O. Kabil and R. Banerjee, J. Biol. Chem., 2010, 285, 21903 CrossRef CAS PubMed;
(d) S. Singh and R. Banerjee, Biochim. Biophys. Acta, 2011, 1814, 1518 CrossRef CAS PubMed.
-
(a) K. Qu, S. W. Lee, J. S. Bian, C. M. Low and P. T. H. Wong, Neurochem. Int., 2008, 52, 155 CrossRef CAS PubMed;
(b) R. Hosoki, N. Matsuki and H. Kimura, Biochem. Biophys. Res. Commun., 1997, 237, 527 CrossRef CAS PubMed;
(c) W. Zhao, J. Zhang, Y. Lu and R. Wang, EMBO J., 2001, 20, 6008 CrossRef CAS PubMed;
(d) D. J. Elsey, R. C. Fowkes and G. F. Baxter, Cell Biochem. Funct., 2010, 28, 95 CrossRef CAS PubMed.
-
(a) K. Eto, T. Asada, K. Arima, T. Makifuchi and H. Kimura, Biochem. Biophys. Res. Commun., 2002, 293, 1485 CrossRef CAS PubMed;
(b) Y. Kaneko, Y. Kimura, H. Kimura and I. Niki, Diabetes, 2006, 55, 1391 CrossRef CAS PubMed;
(c) G. Yang, L. Wu, B. Jiang, W. Yang, J. Qi, K. Cao, Q. Meng, A. K. Mustafa, W. Mu, S. Zhang, S. H. Snyder and R. Wang, Science, 2008, 322, 587 CrossRef CAS PubMed.
-
(a) U. Hannestad, S. Margheri and B. Sorbo, Anal. Biochem., 1989, 178, 394 CrossRef CAS PubMed;
(b) J. Radford-Knoery and G. A. Cutter, Anal. Chem., 1993, 65, 976 CrossRef CAS;
(c) D. Jimenez, R. Martinez-Manez, F. Sancenon, J. V. Ros-Lis, A. Benito and J. Soto, J. Am. Chem. Soc., 2003, 125, 9000 CrossRef CAS PubMed;
(d) N. S. Lawrence, J. Davis and R. G. Compton, Talanta, 2000, 50, 771 CrossRef;
(e) N. S. Lawrence, J. Davis, L. Jiang, T. G. J. Jones, S. N. Davies and R. G. Compton, Electroanalysis, 2000, 12, 1453 CrossRef CAS.
-
(a) K. P. Carter, A. M. Young and A. E. Palmer, Chem. Rev., 2014, 114, 4564 CrossRef CAS PubMed;
(b) X. Chen, T. Pradhan, F. Wang, J. S. Kim and J. Yoon, Chem. Rev., 2012, 112, 1910 CrossRef CAS PubMed;
(c) V. S. Lin, W. Chen, M. Xian and C. J. Chang, Chem. Soc. Rev., 2015, 44, 4596 RSC;
(d) H. Zhu, J. Fan, B. Wang and X. Peng, Chem. Soc. Rev., 2015, 44, 4337 RSC;
(e) R. T. K. Kwok, C. W. T. Leung, J. W. Y. Lam and B. Z. Tang, Chem. Soc. Rev., 2015, 44, 4228 RSC;
(f) J. Yin, Y. Hu and J. Yoon, Chem. Soc. Rev., 2015, 44, 4691 RSC;
(g) M. Kaur and D. H. Choi, Chem. Soc. Rev., 2015, 44, 58 RSC;
(h) Z. Guo, S. Park, J. Yoon and I. Shin, Chem. Soc. Rev., 2014, 43, 16 RSC;
(i) L. Yuan, W. Lin, K. Zheng, L. He and W. Huang, Chem. Soc. Rev., 2013, 42, 622 RSC;
(j) J. Fan, M. Hu, P. Zhan and X. Peng, Chem. Soc. Rev., 2013, 42, 29 RSC.
-
(a) A. R. Lippert, E. J. New and C. J. Chang, J. Am. Chem. Soc., 2011, 133, 10078 CrossRef CAS PubMed;
(b) H. Peng, Y. Cheng, C. Dai, A. L. King, B. L. Predmore, D. J. Lefer and B. A. Wang, Angew. Chem., Int. Ed., 2011, 50, 9672 CrossRef CAS PubMed;
(c) B. Chen, W. Li, C. Lv, M. Zhao, H. Jin, H. Jin, J. Du, L. Zhang and X. Tang, Analyst, 2013, 138, 946 RSC;
(d) J. Zhang and W. Guo, Chem. Commun., 2014, 50, 4214 RSC;
(e) Q. Qiao, M. Zhao, H. Lang, D. Mao, J. Cui and Z. Xu, RSC Adv., 2014, 4, 25790 RSC;
(f) Y. Cai, L. Li, Z. Wang, J. Z. Sun, A. Qin and B. Z. Tang, Chem. Commun., 2014, 50, 8892 RSC;
(g) Z. Wu, Z. Li, L. Yang, J. Han and S. Han, Chem. Commun., 2012, 48, 10120 RSC;
(h) S. K. Bae, C. H. Heo, D. J. Choi, D. Sen, E.-H. Joe, B. R. Cho and H. M. A. Kim, J. Am. Chem. Soc., 2013, 135, 9915 CrossRef CAS PubMed;
(i) L. Zhang, S. Li, M. Hong, Y. Xu, S. Wang, Y. Liu, Y. Qian and J. Zhao, Org. Biomol. Chem., 2014, 12, 5115 RSC;
(j) M.-Y. Wu, K. Li, J.-T. Hou, Z. Huang and X.-Q. Yu, Org. Biomol. Chem., 2012, 10, 8342 RSC;
(k) L. Yuan and Q.-P. Zuo, Sens. Actuators, B, 2014, 196, 151 CrossRef CAS;
(l) C. Tang, Q. Zheng, S. Zong, Z. Wang and Y. Cui, Sens. Actuators, B, 2014, 202, 99 CrossRef CAS;
(m) X. Cao, W. Lin, K. Zheng and L. He, Chem. Commun., 2012, 48, 10529 RSC;
(n) D. Maity, A. Raj, P. K. Samanta, D. Karthigeyan, T. K. Kundu, S. K. Patib and T. Govindaraju, RSC Adv., 2014, 4, 11147 RSC;
(o) T. Liu, Z. Xu, D. R. Spring and J. Cui, Org. Lett., 2013, 15, 2310 CrossRef CAS PubMed;
(p) L. Long, L. Zhou, L. Wang, S. Meng, A. Gong, F. Du and C. Zhang, Org. Biomol. Chem., 2013, 11, 8214 RSC;
(q) Y. Qian, J. Karpus, O. Kabil, S.-Y. Zhang, H.-L. Zhu, R. Banerjee, J. Zhao and C. He, Nat. Commun., 2011, 2, 495 CrossRef PubMed;
(r) W. Xuan, R. Pan, Y. Cao, K. Liu and W. Wang, Chem. Commun., 2012, 48, 10669 RSC;
(s) L. A. Montoya and M. D. Pluth, Chem. Commun., 2012, 48, 4767–4769 RSC;
(t) X. Wang, J. Sun, W. Zhang, X. Ma, J. Lv and B. Tang, Chem. Sci., 2013, 4, 2551 RSC;
(u) K. Sasakura, K. Hanaoka, N. Shibuya, Y. Mikami, Y. Kimura, T. Komatsu, T. Ueno, T. Terai, H. Kimura and T. Nagano, J. Am. Chem. Soc., 2011, 133, 18003 CrossRef CAS PubMed;
(v) F. Hou, J. Cheng, P. Xi, F. Chen, L. Huang, G. Xie, Y. Shi, H. Liu, D. Bai and Z. Zeng, Dalton Trans., 2012, 41, 5799 RSC;
(w) X. Li, Y. Gong, K. Wu, S. H. Liang, J. Cao, B. Yang, Y. Hu and Y. Han, RSC Adv., 2014, 4, 36106 RSC;
(x) M. Zhao, H. Li, H. Li, Q. Qiao, C. Cao and Z. Xu, RSC Adv., 2015, 5, 86355 RSC;
(y) J. Cheng, B. Shao, S. Zhang, Y. Hu and X. Li, RSC Adv., 2015, 5, 65203 RSC;
(z) K. Huang, L. Yu, P. Xu, X. Zhang and W. Zeng, RSC Adv., 2015, 5, 17797 RSC;
(a
a) J. Li, C. Yin and F. Huo, RSC Adv., 2015, 5, 2191 RSC;
(a
b) T. Saha, D. Kand and P. Talukdar, RSC Adv., 2015, 5, 1438 RSC;
(a
c) L. Zhang, W.-Q. Meng, L. Lu, Y.-S. Xue, C. Li, F. Zou, Y. Liu and J. Zhao, Sci. Rep., 2014, 4, 5870 CAS;
(a
d) L. Zhang, S. Li, M. Hong, Y. Xu, S. Wang, Y. Liu, Y. Qian and J. Zhao, Org. Biomol. Chem., 2014, 12, 5115 RSC.
- Y. Tang, D. Lee, J. Wang, G. Li, J. Yu, W. Lin and J. Yoon, Chem. Soc. Rev., 2015, 44, 5003–5015 RSC.
-
(a) S. Zhang, M. Zhao, W. Zhu, Y. Xu and X. Qian, Dalton Trans., 2015, 44, 9740 RSC;
(b) K. Huang, L. Yu, P. Xu, X. Zhang and W. Zeng, RSC Adv., 2015, 5, 17797 RSC;
(c) J.-L. Tang, C.-Y. Li, Y.-F. Li and C.-X. Zou, Chem. Commun., 2014, 50, 15411 RSC;
(d) L. Wang, L. Long, L. Zhou, Y. Wu, C. Zhang, Z. Han, J. Wang and Z. Da, RSC Adv., 2014, 4, 59535 RSC;
(e) J. Zhou, Y. Li, J. Shen, Q. Li, R. Wang, Y. Xu and X. Qian, RSC Adv., 2014, 4, 51589 RSC;
(f) C. S. Lim, G. Masanta, H. J. Kim, J. H. Han, H. M. Kim and B. R. Cho, J. Am. Chem. Soc., 2011, 133, 11132 CrossRef CAS PubMed.
-
(a) H.-I. Un, S. Wu, C.-B. Huang, Z. Xu and L. Xu, Chem. Commun., 2015, 51, 3143 RSC;
(b) D. Li, X. Chen, Y. Li, T. Cheng, W. Zhu, Y. Xu and X. Qian, Anal. Methods, 2014, 6, 8890 RSC;
(c) Z. Xu, J. Yoon and D. R. Spring, Chem. Commun., 2010, 46, 2563 RSC.
-
(a) Z.-R. Dai, G.-B. Ge, L. Feng, J. Ning, L.-H. Hu, Q. Jin, D.-D. Wang, X. Lv, T.-Y. Dou, J.-N. Cui and L. Yang, J. Am. Chem. Soc., 2015, 137, 14488 CrossRef CAS PubMed;
(b) C. Liu, H. Wu, Z. Wang, C. Shao, B. Zhu and X. Zhang, Chem. Commun., 2014, 50, 6013 RSC.
- C. A. Blencowe, A. T. Russell, F. Greco, W. Hayes and D. W. Thornthwaite, Polym. Chem., 2011, 2, 773 RSC.
Footnote |
† Electronic supplementary information (ESI) available: Experimental details, characterization of the compounds, and additional spectroscopic data. See DOI: 10.1039/c6ra01242f |
|
This journal is © The Royal Society of Chemistry 2016 |
Click here to see how this site uses Cookies. View our privacy policy here.