DOI:
10.1039/C6RA00983B
(Paper)
RSC Adv., 2016,
6, 32046-32051
A ratiometric fluorescent probe for quantification of alkaline phosphatase in living cells†
Received
12th January 2016
, Accepted 21st March 2016
First published on 24th March 2016
Abstract
A ratiometric fluorescent probe with strong intramolecular charge transfer (ICT) character has been designed for the detection of alkaline phosphatase (ALP). In the presence of ALP, probe 1 showed a dramatic bathochromic shift from 550 to 650 nm in the fluorescence spectrum and an obvious ratiometric signal with an isoemissive point was observed. The spectral change was attributed to the hydrolysis and cleavage of a phosphate group from the probe that changed the ability of the intramolecular charge transfer. The fluorescence intensity ratio displayed a linear relationship against the concentration of ALP in the concentration range from 50 to 200 U L−1. Other biological species, including lysozyme, trypsin, pepsin, acetyl cholinesterase, carboxylesterase, and bovine serum albumin, did not induce distinct spectral changes of the probe, indicating its selective sensing ability to ALP. Furthermore, probe 1 was also successfully applied to image endogenous ALP activity in living cells.
Introduction
Alkaline phosphatase (ALP) is a hydrolase that is responsible for the removal of phosphate groups from many kinds of biological substrates such as proteins, nucleic acids, carbohydrates and other small molecules.1 It is widespread in mammalian tissues, but mostly distributed in the intestine, placenta, bone, liver and kidney.2 The average serum ALP range of normal adults varies from 40 U L−1 to 150 U L−1.3 Abnormal expression, mainly related to elevated levels of ALP is usually in connection with some diseases, i.e., osteoporosis,3 hepatitis,4 prostatic cancer,5 and bone cancer.6 Therefore, the alkaline phosphatase has been identified as an important biomarker for clinical diagnostics.7 Those techniques which could reveal the information of ALP, such as its distribution and activity, are very helpful for medical researchers to know the mechanism of these diseases so as to find an efficient cure method.
Recently, various assays for ALP detection have been developed, including electrochemistry,8,9 colorimetric method,10,11 and surface enhancement Raman scattering assay.12 Although these provide efficient ways for ALP detection, they often need laborious experimental procedures or expensive instruments, and thus they are not appropriate for applications in living cells. Alternatively, fluorescent probes especially those based on small molecules have attracted much attention due to their intrinsic advantages such as low background noise, high sensitivity and selectivity.13–15 However, till now, only a few fluorescent probes have been developed for ALP sensing. For example, Song and coauthors developed a phosphorylated tetraphenylethene derivative as a fluorescence turn-on probe based on aggregation induced emission mechanism.3 Kim and coauthors reported a series of fluorescent probes based on iminocoumarin-benzothiazole for ALP imaging in living cells.16,17 Although these probes showed good sensing properties, their shorter emission wavelength and single wavelength as output signal will limit their potential applications in living cells. Therefore, fluorescent probes with longer emission wavelength and multiple output signals are more preferable.
In this communication, we have designed a dicyano-based compound with strong ICT character as a fluorescent probe for the detection of ALP (Scheme 1). The phosphate group functions as a recognizing group that can be released from the probe in the presence of ALP, as well as a modulator to tune the ICT ability of the phenolic hydroxyl through the hydrolysis of phosphomonoester. As expected, the probe 1 upon interaction with ALP shows a distinct emission red shift from 550 nm to 650 nm. Therefore, the ICT process could be regulated that can result in ratiometric signals both in the absorption and fluorescence spectra. Ratiometric fluorescent probes which employ two fluorescence intensity as output signal are more desirable as they are free of interference from autofluorescence, probe concentration and emission intensity.18 The intensity ratio of the probe also shows a linear relationship with ALP concentration through a wide concentration range of ALP. Besides, the probe 1 was applied to endogenous enzyme activity imaging in living cells.
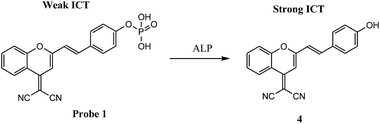 |
| Scheme 1 Dephosphorylation of the probe 1 in the presence of ALP. | |
Experimental
Chemicals and materials
Diethyl chlorophosphate and 4-hydroxybenzaldehyde were purchased from Energy Chemical. Iodotrimethylsilane, triethylamine, calcium hydride, and 2′-hydroxyacetophenone were purchased from J&K Chemicals. Anhydrous tetrahydrofuran was purchased from Tianjin Heowns Biochemical Technology. Malononitrile and 4-dimethylaminopyridine were purchased from Sino Reagent. Alkaline phosphatase from calf intestine was purchased from Sigma Aldrich. Other enzymes used in selectivity experiment were purchased from Amresco LLD, Acros Organics and BoiDee Biotechnology. Anhydrous dichloromethane was prepared by refluxing analytical grade dichloromethane with calcium hydride. Unless noted, all the chemicals were of analytical grade and used as received without further purification.
Instrument
1H NMR and 13C NMR spectra were recorded on a Bruker 400 MHz NMR spectrometer instrument. Mass spectra were recorded on Waters GCT Premier or Shimadzu LC-MS 2010 mass spectrometer, respectively. Absorption spectra and fluorescence spectra were recorded on Hitachi U-3900 UV-Vis absorption spectrophotometer and Hitachi F-4600 fluorescence spectrophotometer, respectively. Fluorescence imaging was taken with a Nikon C1si laser scanning confocal microscope.
Cell viability
Hela cells in a 96-well plate were firstly cultured using DMEM for 12 h. Then the cell medium was exchanged with fresh medium containing different amount of probe (final concentration, 0, 2, 4, 6, 8, 10 μM). Hela cells were further cultured for 2 h after the medium was exchanged with fresh medium again. The cells were further incubated for 24 h at 37 °C under the atmosphere of 5% CO2. Then the cell medium was exchanged with a mixture of 100 μL fresh medium and 20 μL MTT (5 mg mL−1). After incubation for 4 h under the same condition, the cell medium was discarded and DMSO (100 μL) was added. All wells were then measured at 570 nm, and cell viability of untreated cells was set to 100% as a reference.
ALP imaging in living cells
As placental ALP is over-expressed in human uterine cervical cancer cells, Hela cells were used as the cell experiment. Cells were cultured in three confocal dishes in the culture medium overnight at 37 °C under a CO2 (5%) atmosphere. Then three confocal dishes were treated differently and imaged on a Nikon C1si laser scanning confocal microscope. The images were obtained from the red channel with an excitation at 488 nm. In the first dish, Hela cells were imaged without treatment of the probe or sodium orthovanadate. In the second dish, Hela cells were incubated with the probe (10 μM) for 30 min before imaging, in the third dish, Hela cells were imaged after incubation with ALP inhibitor sodium orthovanadate (final concentration 1 mM) for 30 min, then with the probe (10 μM) for another 30 min.
Synthetic procedures of the probe 1
Preparation of 2-(2-methyl-4H-chromen-4-ylidene)malononitrile (compound 3)
Compound 3 was prepared in a modified way according to literature reported.19 2-Hydroxyacetophenone (0.5 g, 3.7 mmol) and sodium (0.6 g, 26.1 mmol) were added to a 50 mL flask charged with 20 mL anhydrous ethyl acetate. The mixture was stirred under room temperature for about 18 h. The mixture was slowly poured into 50 mL cold water then neutralized with dilute HCl solution. The organic portion was separated and dried with sodium sulfate, filtered and evaporated under reduced pressure to give the desired product which was used directly in the next step without further purification. The crude product was dissolved in 20 mL methanol in a 50 mL flask, and a few drops of hydrochloric acid was added. The reaction mixture was stirred under room temperature for 4 h. Then methanol was evaporated and the residue was extracted with dichloromethane and washed with saturated NaCl. The organic layer was dried, filtered and purified using silica gel column chromatography to obtain compound 2 as a yellow solid (0.3 g, 50%).
The intermediate 2 (0.4 g, 2.5 mmol) and malononitrile (0.22 g, 3.0 mmol) were added to 25 mL acetic anhydride with continuous stirring. The mixture was heated to reflux for 14 h to complete the reaction, then the solvent was evaporated. Water (50 mL) was added to the residue and the mixture was further refluxed for 30 min, followed by extraction with dichloromethane. The organic layer was dried, filtered and purified with silica gel column chromatography to yield the intermediate 3 as an orange solid (0.15 g, 29%). 1H NMR (400 MHz, CDCl3, ppm): δ = 8.92 (d, J = 8.7 Hz, 1H), 7.72 (t, J = 7.8 Hz, 1H), 7.46 (t, J = 7.0 Hz, 2H), 6.72 (s, 1H), 2.44 (s, 3H). MS (EI): m/z = 208.
Synthesis of 2-(2-(4-hydroxystyryl)-4H-chromen-4-ylidene)malononitrile (compound 4)
The intermediate 4 was prepared in a modified way according to literature reported.20 Compound 3 (0.15 g, 0.7 mmol), 4-hydroxybenzaldehyde (0.10 g, 0.8 mmol), together with catalytic amount of acetic acid (0.5 mL) and piperidine (0.5 mL) were added to a round-bottom flask with stirring anhydrous toluene (20 mL). The mixture was refluxed for 12 h then solvent was removed under vacuum. The crude product was further purified with silica gel column chromatography to obtain compound 4 as an orange solid (0.1 g, 44%). 1H NMR (400 MHz, DMSO-d6, ppm): δ = 10.15 (s, 1H), 8.74 (d, J = 8.4 Hz, 1H), 7.94–7.90 (m, 1H), 7.80 (d, J = 8.4 Hz, 1H), 7.70 (d, J = 16.0 Hz, 1H), 7.65–7.59 (m, 3H), 7.29 (d, J = 16.0 Hz, 1H), 6.96 (s, 1H), 6.86 (d, J = 8.6 Hz, 2H). MS (EI): m/z = 312.
Synthesis of 4-((E)-2-(4-(dicyanomethylidene)-4H-chromen-2-yl)ethenyl)phenyl diethyl phosphate (compound 5)
The intermediate 4 (0.20 g, 0.6 mmol) and DMAP (50 mg) were dissolved in dry THF. Diethyl chlorophosphate (0.15 g, 0.9 mmol) and triethylamine (0.3 mL) were added under the nitrogen atmosphere. The mixture was stirred at room temperature overnight. The reaction mixture was condensed under reduced pressure and purified with column chromatography to give the desired 5 as a light brown solid (0.11 g, 35%). 1H NMR (400 MHz, CDCl3, ppm): δ = 8.92 (d, J = 8 Hz, 1H), 7.75 (t, J = 8 Hz, 1H), 7.62–7.56 (m, 4H), 7.46 (t, J = 8 Hz, 1H), 7.31 (d, J = 8 Hz, 2H), 6.87 (s, 1H), 6.76 (d, J = 16 Hz, 1H), 4.29–4.22 (m, 4H), 1.38 (t, J = 7 Hz, 6H). 13C NMR (400 MHz, CDCl3, ppm): δ = 157.42, 152.86, 152.60, 152.52, 137.81, 134.80, 131.71, 129.54, 126.03, 120.95, 118.03, 116.74, 115.66, 107.01, 64.99, 64.93, 63.43, 58.52, 18.55, 16.24, 16.18. HRMS (EI): calcd m/z = 448.1188, obsd m/z = 448.1185.
Synthesis of 4-((E)-2-(4-(dicyanomethylidene)-4H-chromen-2-yl)ethenyl)phenyl phosphate (the probe 1)
4-((E)-2-(4-(Dicyanomethylidene)-4H-chromen-2-yl)ethenyl)phenyl diethyl phosphate (0.1 g, 0.2 mmol) was dissolved in anhydrous dichloromethane. Iodotrimethylsilane (2 equiv.) was added and the mixture was stirred under room temperature for 4 h. The solvent was removed to yield a dark solid (80 mg, 100%). 1H NMR (400 MHz, DMSO-d6, ppm): δ = 8.74 (d, J = 8.4 Hz, 1H), 7.93 (t, J = 8.2 Hz, 1H), 7.82–7.73 (m, 4H), 7.62 (t, J = 8.1 Hz, 1H), 7.46 (d, J = 16.1 Hz, 1H), 7.26 (d, J = 8.4 Hz, 2H), 7.03 (s, 1H), –OH (not found). 13C NMR (100 MHz, DMSO-d6, ppm): δ = 158.58, 153.69, 153.62, 153.31, 152.44, 138.34, 135.87, 131.24, 130.13, 126.61, 125.08, 121.06, 121.01, 119.51, 119.32, 117.59, 117.52, 116.25, 107.03, 60.71. HRMS (ESI): m/z = 391.0490 [M − H]−.
Results and discussion
The synthetic route of the probe 1 was shown in Scheme 2. The structure of 1 has been verified by 1H NMR, 13C NMR and mass spectra analyses. The intermediate 4 which undergoes an ICT process between the dicyano group and phenolic hydroxyl group was chosen as a fluorescence generator. Phosphate group was introduced to the compound 4 through a condensation and hydrolysis reaction to give the probe 1. The phosphate group functions as a recognizing group which could be selectively cleaved in the presence of alkaline phosphatase. As a weak electron withdrawing group, the presence of phosphate group could reduce the electron donating ability of the oxygen atom of phenolic hydroxyl group, thus different ICT intensities occurred before and after the cleavage of phosphate group, resulting in obvious spectral shift and intensity change.
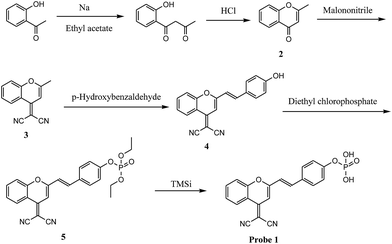 |
| Scheme 2 Synthetic procedures of the probe 1. | |
The intermediate 4 in Tris–HCl buffer solution (10 mM, pH = 7.4, 25 °C) shows a characteristic absorption band at 395 nm and an emission band at 650 nm, respectively (Fig. S1†). And its molar extinction coefficient at 395 nm is 1.1 × 104 M−1 cm−1. In contrast, the probe 1 showed distinct different characteristic absorption and emission bands peaked at 440 nm and 550 nm, respectively. The molar extinction coefficient of the probe 1 at 440 nm is 2.2 × 104 M−1 cm−1. Emission spectrum of the probe 1 changed with different pH conditions (Fig. S3†). The emission spectra of 1 remain stable in the pH range from 7 to 10, and it was sharply decreased in the acidic solution with pH from 7 to 3. Compound 4 is also pH sensitive from pH 3 to 10, as shown in Fig. S4.† In this work, the physiological pH was used for the spectral tests. In addition, fluorescence quantum yields of 1 and 4 were calculated to be 10.5% and 7.8%, respectively, using rhodamine 101 as the reference compound.21
Upon addition of ALP (500 U L−1) to the solution of the probe 1 (50 μM), the absorption spectrum was recorded every two minutes over 30 min (Fig. 1a). For every two minutes, the maximum absorption decreased gradually with a concomitant increase around 525 nm. However, its absorption around 395 nm only decreased slightly, becoming the maximum absorption after 1 was further incubated with ALP. After half an hour later, the reaction can reach a platform and the absorption cannot be variable. The fluorescence response of 1 towards ALP was recorded for half an hour under the same condition. As illustrated in Fig. 1b, the maximum emission decreased gradually with the time elapsing as 1 was incubated with ALP, and a new peak at 650 nm emerged simultaneously which progressively became dominant after further incubation. The probe 1 shows a colour change from yellow-green to light orange (Fig. S5†). Obviously, bathochromic shift of the spectra and color change were attributed to stronger an ICT process resulted from the cleavage of phosphate group by ALP. The absorption and emission spectra of the probe 1 after incubation with ALP for 30 min are very similar to those of the compound 4 (Fig. S2†), also indicating that 1 in the presence of ALP can produce enzymatic product 4. To further prove the conversion of 1 to 4 by ALP, enzymatic product has been studied through ESI mass spectrum as follows, the probe 1 (100 μM) was incubated with ALP in PBS buffer for 40 min, and the resulting precipitate was analyzed (Fig. S6†). It was found that the ESI peak of enzymatic product 4 (311.0825) was clearly observed and no signals for the probe 1 was found, indicating that the full conversion of the probe 1 into enzymatic product 4 in the presence of ALP.
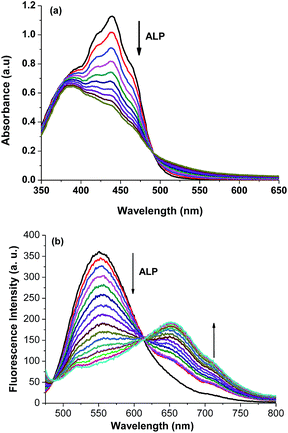 |
| Fig. 1 Time-dependent absorption (a) and emission (b) spectra of the probe 1 (50 μM) in the presence of ALP (500 U L−1) in Tris–HCl buffer solution (10 mM, pH = 7.4, 25 °C) recorded within 30 min. | |
In order to verify whether the probe 1 has the ability to quantify ALP concentration, the fluorescence response of 1 (50 μM) in Tris–HCl buffer (10 mM, pH = 7.4) towards different amount of ALP (10–500 U L−1) were recorded at room temperature. As shown in Fig. 2, higher ALP concentration give rise to higher fluorescence ratio (F650/F550). Moreover, the fluorescence ratio was plotted versus ALP concentration after the probe 1 was incubated with different amount of ALP for 30 min, and they exhibited a good linear relationship through a wide concentration range of 50–200 U L−1. The linear regression of equation is as follows: F650/F550 = 0.00325 × [ALP] + 0.0297 (U L−1), r = 0.99615. The detection limit calculated from the former equation is 3.8 U L−1, low enough for ALP detection in biological samples, because in human serum the normal ALP range is 40–150 U L−1.
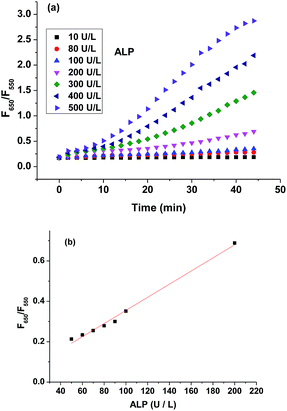 |
| Fig. 2 (a) Time-dependant fluorescence intensity ratio (F650/F550) of the probe 1 (50 μM) in the presence of different amount of ALP (10–500 U L−1) in Tris–HCl buffer solution (10 mM, pH = 7.4, 25 °C). (b) Fluorescence intensity ratio of 1 as a function of ALP concentration after ALP was incubated with different amount of ALP for 30 min. | |
Selectivity is a very important parameter for evaluating its practical application. To verify whether this probe is selective to ALP, fluorescence responses of 1 towards competitive biological analytes were investigated under the same conditions (Tris–HCl buffer, 10 mM, pH = 7.4) (Fig. 3). Other enzymes including pepsin, lysozyme, trypsin, acetylcholinesterase (AChE), human carboxylesterase (hCEs) and bovine serum albumin (BSA) were added to the solution of 1, respectively, and fluorescence responses were then recorded. The results showed that no significant fluorescence changes were observed, demonstrating its high selectivity toward ALP.
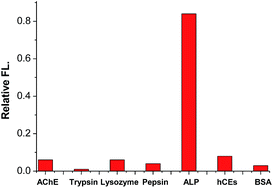 |
| Fig. 3 Fluorescence changes at 650 nm of the probe 1 in the presence of the same amount of AChE, trypsin, lysozyme, pepsin ALP, hCEs and BSA in Tris–HCl buffer solution (10 mM, pH = 7.4, 25 °C). | |
To further verify the hydrolysis reaction of 1 in the presence of ALP, its inhibition effect has been studied. Sodium orthovanadate is a well known inhibitor for alkaline phosphatase.22 Fluorescence responses of 1 (50 μM) with ALP (0.5 U mL−1) in the absence and presence of different amount of sodium orthovanadate were recorded after the mixture were incubated for 30 min. And fluorescence intensity ratio was plotted versus the incubation time. As displayed in Fig. 4, the ALP activity was inhibited in a dose dependent manner. Particularly, in the presence of 1 mM sodium orthovanadate, the fluorescence ratio shows no variations after 30 min, indicating a complete inhibition of ALP activity.
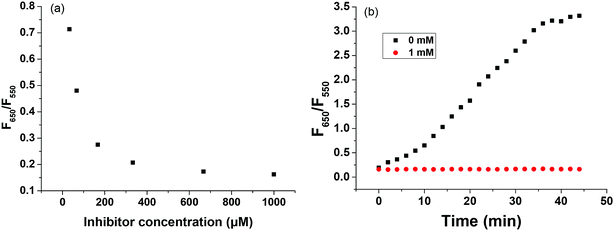 |
| Fig. 4 (a) Fluorescence intensity ratio (F650/F550) of the probe 1 in the presence of ALP and different amount of sodium orthovanadate after incubation for 30 min. (b) Fluorescence intensity ratio (F650/F550) change of 1 and ALP mixture in the absence and presence of sodium orthovanadate. | |
To find out whether the probe has a potential to be applied in fluorescence imaging for ALP activity in Hela cells, MTT assay was carried out to evaluate cell viability in the presence of different amount of 1. As shown in Fig. 5, the probe 1 shows very low cytotoxicity even at a concentration up to 10 μM. Thus, this probe is biocompatible and capable for the cell culture.
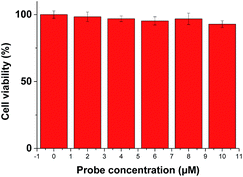 |
| Fig. 5 Cytotoxicity of the probe 1 with different concentrations in Hela cells. | |
With the low cytotoxicity and good selectivity, the probe 1 was further applied for imaging endogenous alkaline phosphatase activity in living cells. As well known, placental alkaline phosphatase could be expressed in Hela cells.17,23 Thus Hela cells were used for in vitro experiment. As shown in Fig. 6, Hela cells in the absence of 1 showed no fluorescence, while significant red fluorescence was observed after incubation with the intracellular ALP. This result indicates the existence of ALP in Hela cells. To further prove the fact that the fluorescence was triggered by the dephosphorylation of endogenous ALP in Hela cells, a comparison experiment was carried out in which Hela cells was incubated with ALP inhibitor (sodium orthovanadate 1 mM) for 30 min prior to the addition of 1. As expected, only negligible fluorescence was observed, indicating that this probe can function well to monitor ALP activity in living cells.
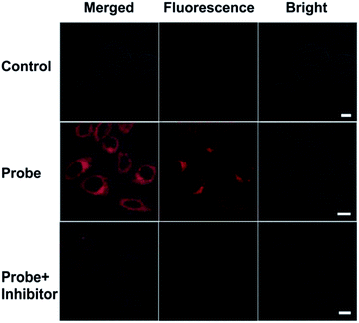 |
| Fig. 6 Confocal laser scanning imaging. First row: control; second row: Hela cells incubated with 1 (10 μM) for 30 min; third row: Hela cells incubated with sodium orthovanadate for 30 min, then incubated with 1 for another 30 min. | |
Conclusion
In summary, a ratiometric fluorescent probe for the sensitive sensing of ALP activity has been reported. In the presence of ALP, fluorescence intensity ratio showed a ratiometric fluorescence change, whereas other competitive biological species did not cause distinct fluorescence changes. The probe 1 also gives a linear relationship versus ALP concentration in a wide range from 50 to 200 U L−1, making it possible to quantify the concentration in intracellular ALP. With low cytotoxicity, the probe has been applied in fluorescence imagining in living cells. Confocal laser scanning imaging indicates that this probe is capable of imaging endogenous ALP activity in Hela cells.
Acknowledgements
This work was supported by Major State Basic Research Development Program of China (No. 2014CB932600) and the NNSF of China (Grant No. 21173244).
Notes and references
- Z. Song, R. T. Kwok, E. Zhao, Z. He, Y. Hong, J. W. Lam, B. Liu and B. Z. Tang, ACS Appl. Mater. Interfaces, 2014, 6, 17245–17254 CAS.
- X. Hou, Q. Yu, F. Zeng, J. Ye and S. Wu, J. Mater. Chem. B, 2015, 3, 1042–1048 RSC.
- Z. Song, Y. Hong, R. T. K. Kwok, J. W. Y. Lam, B. Liu and B. Z. Tang, J. Mater. Chem. B, 2014, 2, 1717 RSC.
- S. K. Mwilu, V. A. Okello, F. J. Osonga, S. Miller and O. A. Sadik, Analyst, 2014, 139, 5472–5481 RSC.
- G. Ramaswamy, V. R. Rao, L. Krishnamoorthy, G. Ramesh, R. Gomathy and D. Renukadevi, Indian J. Clin. Biochem., 2000, 15, 110–113 CrossRef CAS PubMed.
- M. Kleerekoper and F. Al-Khayer, Encycl. Endocr. Dis., 2004, 3, 425–431 CAS.
- J. Ozer, M. Ratner, M. Shaw, W. Bailey and S. Schomaker, Toxicology, 2008, 245, 194–205 CrossRef CAS PubMed.
- K. Ino, Y. Kanno, T. Arai, K. Y. Inoue, Y. Takahashi, H. Shiku and T. Matsue, Anal. Chem., 2012, 84, 7593–7598 CrossRef CAS PubMed.
- S. Goggins, C. Naz, B. J. Marsh and C. G. Frost, Chem. Commun., 2015, 51, 561–564 RSC.
- C. M. Li, S. J. Zhen, J. Wang, Y. F. Li and C. Z. Huang, Biosens. Bioelectron., 2013, 43, 366–371 CrossRef CAS PubMed.
- H. Jiao, J. Chen, W. Li, F. Wang, H. Zhou, Y. Li and C. Yu, ACS Appl. Mater. Interfaces, 2014, 6, 1979–1985 CAS.
- C. Ruan, W. Wang and B. Gu, Anal. Chem., 2006, 78, 3379–3384 CrossRef CAS PubMed.
- S. Maruyama, K. Kikuchi, T. Hirano, Y. Urano and T. Nagano, J. Am. Chem. Soc., 2002, 124, 10650–10651 CrossRef CAS PubMed.
- J. Zhang, R. E. Campbell, A. Y. Ting and R. Y. Tsien, Nat. Rev. Mol. Cell Biol., 2002, 3, 906–918 CrossRef CAS PubMed.
- X. Peng, J. Du, J. Fan, J. Wang, Y. Wu, J. Zhao, S. Sun and T. Xu, J. Am. Chem. Soc., 2007, 129, 1500–1501 CrossRef CAS PubMed.
- J. Park and Y. Kim, Bioorg. Med. Chem. Lett., 2013, 23, 2332–2335 CrossRef CAS PubMed.
- T.-I. Kim, H. Kim, Y. Choi and Y. Kim, Chem. Commun., 2011, 47, 9825–9827 RSC.
- X. Zhang, Y. Xiao and X. Qian, Angew. Chem., 2008, 47, 8025–8029 CrossRef CAS PubMed.
- Y. Zheng, M. Zhao, Q. Qiao, H. Liu, H. Lang and Z. Xu, Dyes Pigm., 2013, 98, 367–371 CrossRef CAS.
- D. Yu, Q. Zhang, S. Ding and G. Feng, RSC Adv., 2014, 4, 46561–46567 RSC.
- R. F. Kubin and A. N. Fletcher, J. Lumin., 1982, 27, 455–462 CrossRef.
- I. Gibbons, M. P. Cosson, J. A. Evans, B. H. Gibbons, B. Houck, K. H. Martinson, W. S. Sale and W. Tang, Proc. Natl. Acad. Sci. U. S. A., 1978, 75, 2220–2224 CrossRef CAS.
- F. Herz, A. Schermer, M. Halwer and L. H. Bogart, Arch. Biochem. Biophys., 1981, 210, 581–591 CrossRef CAS PubMed.
Footnote |
† Electronic supplementary information (ESI) available: Additional spectra and NMR copies. See DOI: 10.1039/c6ra00983b |
|
This journal is © The Royal Society of Chemistry 2016 |