DOI:
10.1039/C6RA00692B
(Paper)
RSC Adv., 2016,
6, 31831-31839
Fluorescent active ruthenium(II) complex units containing bpy or phen or dmp ligands anchored on branched poly(ethylenimine): DNA binding and in vitro biological assessment†
Received
9th January 2016
, Accepted 16th March 2016
First published on 18th March 2016
Abstract
Anticancer targeted ruthenium(II) complex units containing bpy(2,2′-bipyridine), phen(1,10-phenanthroline) or 2,9-dmp(2,9-dimethyl 1,10-phenanthroline) anchored on a branched polyethylenimine molecule (RuL-BPEI) were synthesised. These complexes are highly stable, emissive, redox active and show a quasi-reversible response in cyclic voltammetry. Synthesised compounds were characterized by infra-red, UV-visible, NMR, CHN, and AES analysis methods. The interaction of these RuL-BPEI samples with calf thymus DNA was explored using electronic absorption spectroscopy, emission spectroscopy, cyclic voltammetry and circular dichroism techniques. Antimicrobial activities of these polymer metal complexes have been investigated against a range of various bacteria, including clinically resistant microbes. And also anticancer properties have been tested with MCF-7 breast cancer cell lines. All results were positive.
1. Introduction
Ruthenium(II) complexes containing N,N′ aromatic symmetrical ligands display enantiomeric selectivity in binding to DNA.1–3 The studies on ruthenium anticancer compounds are of great importance, leading to the identification of indazoliumbis-indazoletetrachlororuthenate (KP1019, FFC14A) and imidazoliumtrans-imidazoledimethylsulfoxidetetrachlororuthenate (NAMI-A), respectively.4–18 Reduction of ruthenium(III) to ruthenium(II) also directs the solution chemistry of these compounds as shown by experimental and theoretical studies.12,16,19,20 The use of 2,2′-bipyridine and aryl-beta-diketonato ligands have also brought a series of Ru(II)-2,2′-bipyridyl complexes with substituted diazopentane-2,4-diones. Screening compounds with naphtyldiazopentane-2,4-dione as a co-ligand yielded an interesting cytotoxicity on a distinct panel of tumour cells in vitro.21–23 Generally the ruthenium compounds showed a similar activity on sensitive and platinum-resistant cells, comparable to that indicated by cisplatin and greater than that of carboplatin in the sensitive cells.24 Also the selective metastasis inhibition of NAMI-A may be due to an interaction with a cellular target different than DNA. While no unequivocal proof is known yet, the outcomes of several trials support the hypothesis that metastasis reduction is due to the modulation of cell cytoskeleton, prominent to inhibition of the invasion processes.
These investigations reveal that ruthenium complexes are presently an object of great consideration in the area of medicinal chemistry, as antitumor agents with selective antimetastatic properties, enhanced permeability retention (EPR) and low toxicity. Ruthenium compounds appear to invade tumour cells reasonably well and bind strongly to DNA.4 The octahedral coordination geometry preferred by ruthenium(II) complexes makes them advantageous because they are more tuneable anticancer agents than platinum(II) compounds. Therefore the synthesis of many new ruthenium metal complexes, which bind to DNA by non-covalent interactions while intercalative binding, is very important.
In this paper we have analyzed RuL-BPEI DNA binding studies in vitro, both antimicrobial as well as anticancer with the MCF7 cell line therapeutic profile. There are variety of advantages for using polymer ruthenium bipyridyl and phenanthroline complexes including being emissive in nature, and we have developed many biodegradable, high transfection efficient, hydrophilic, cationic branched polyethyleneimine (BPEI) with lower atomic weight metals like cobalt and copper containing N,N′ type ligand complexes. In this direction we have chosen anti-metastatic ruthenium chelated 2,2′-bipyridine (bpy)/1,10-phenanthroline (phen)/2,9-dimethyl 1,10-phenanthroline (dmp) complexes which are attached to the water soluble cationic biodegradable polymeric ligand BPEI.
2. Materials and methods
2.1. Materials
RuCl3·xH2O (Alfa Aesar) and 2,9-dimethyl-1,10-phenanthroline (Sigma Aldrich) were used as received. Branched polyethylenimine (BPEI) 25 kDa and calf thymus DNA were obtained from Sigma Aldrich. All salts and acids were purchased from Merk and Loba chemie (India). Tris–HCl was purchased from Loba Chemie, and sodium chloride (NaCl) was purchased from SISCO Research laboratories, India. A solution of CT-DNA in the buffer gave a ratio of UV absorbance at 260 nm to 280 nm of ∼1.8–1.9
:
1, indicating that the DNA was sufficiently free of protein. All the experiments involving the interaction of RuP-BPEI with CT-DNA were carried out with twice distilled water in buffer containing 5 mM Tris–HCl/50 mM NaCl at pH 7.1.
2.2. Synthesis of RuL-BPEI
2.2.1. Precursor complexes, [Ru(NN)2Cl2] (NN: bipyridine (bpy) or 1,10-phenanthroline (phen) or 2,9-dimethyl 1,10-phenanthroline (2,9-dmp)).
The precursor complexes were synthesised by a reported procedure.25 Briefly, a mixture of RuCl3·3H2O (1.02 g, 3.896 mmol), bpy (1.217 g, 7.792 mmol) or phen (1.4042 g, 7.792 mmol) or 2,9-dmp (1.627 g, 7.792 mmol) and LiCl3 (≈1.0 g) in 25 ml of dimethyl formamide was heated at reflux for 24 h. The reaction mixture was stirred magnetically throughout this period. After that, the mixture was cooled to room temperature, 250 ml of acetone was added and the resultant solution was kept at 0 °C overnight. The dark green-black microcrystalline product obtained was filtered off. The solid was washed three times with 25 ml portions of water followed by 25 ml portion of diethyl ether and then the solid was dried over vacuum.
2.2.2.
RuL-BPEI (RuB-BPEI, RuP-BPEI and RuD-BPEI with corresponding ligand bpy or phen or 2,9-dmp respectively).
In a round bottomed flask, 0.968 g of Ru(bpy)2Cl2 (2 mM) was taken in 30 ml of methanol. To this BPEI (1 g) dissolved in 20 ml of methanol was added. The mixture was warmed at 60 °C for about 12 h with continuous stirring manually. The appearance of the reaction mixture turned to a dark brown colour. Then the reaction mixture was filtered and the filtrate was dialysed for a week against a water/ethanol mixture. Finally RuB-BPEI was obtained like a brown coloured filmy substance after the solvent was rotary evaporated. The other two compounds, RuP-BPEI and RuD-BPEI, were synthesised with a slight modification of reaction conditions along with changing the precursors [Ru(phen)2Cl]Cl 1.065 g (2 mM) or [Ru(2,9-dmp)2Cl]Cl 1.176 g (2 mM) in place of Ru(bpy)2Cl2 in the reaction solution (Scheme 1).
2.2.3. Ruthenium analysis.
The ruthenium chelate content of the complexes (RuL-BPEI) was determined by Atomic Emission Spectroscopy (AES).
2.2.4. Spectral analysis of RuB-BPEI.
IR (KBr pellets, cm−1): 3427 (N–H Str), 1750 (C
N), 1649 (C
C), 1H-NMR: (DMSO, δ ppm) 9.08, 8.77–8.79, 8.56, 8.39, 8.08, 8.05, 7.92, 779, 3.6, 2.86, 2.7, 2.49–2.52 (m), 2.09. UV (nm): 244, 291, 342, 484; CHN analysis: % (wt 1.05 mg), C: 43.46; H: 5.7, N: 16.16.
2.2.5. Spectral analysis of RuP-BPEI.
IR (KBr pellets, cm−1): 3409 (N–H Str), 1733 (C
N), 1626 (C
C), 1H-NMR: (DMSO, δ ppm) 9.9, 9.8, 9.77, 9.02, 8.89, 8.83, 8.7, 8.59, 8.49–8.37, 8.28, 8.14, 808, 7.92, 7.57, 7.36, 3.16, 2.89, 2.76, 2.72. UV (nm): 266, 291, 466; CHN analysis: % (wt 1.052 mg), C: 47.19; H: 5.66, N: 11.79.
2.2.6. Spectral analysis of RuD-BPEI.
IR (KBr pellets, cm−1): 3413, (N–H str), 1631 (C
N), 1567 (C
C), 1H-NMR: (DMSO, δ ppm): 8.670, 8.650 (d), 8.535, 8.515 (d), 8.183, 7.984, 7.915, 7.895, 7.683–7.664 (d), 3.152, 2.853, 2.492, 1.834, 1.209. UV (nm): 208, 223, 269, 493; CHN analysis: % (wt 1.426 mg), C: 44.18; H: 6.34, N: 16.35.
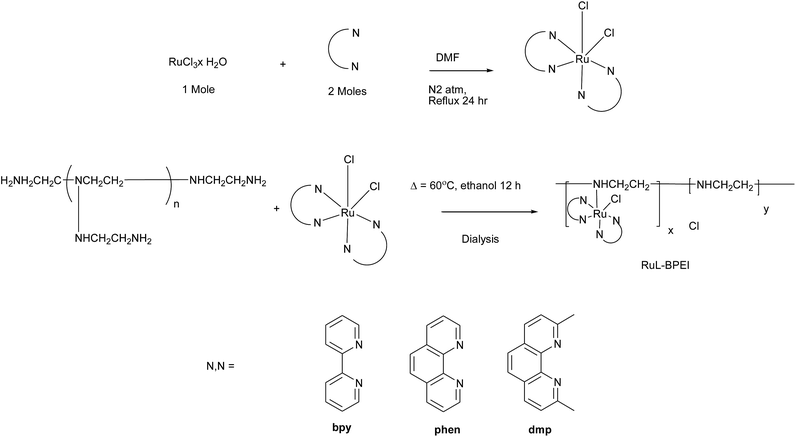 |
| Scheme 1 Systematic synthetic procedure of the synthesis of RuL-BPEI (Table 1). | |
2.3. DNA binding experiments
The DNA binding experiments were carried out using UV-visible absorption studies, fluorescence spectroscopy, cyclic voltammetry and circular dichroism.
2.4. Biological evaluation
2.4.1. Antimicrobial susceptibility test.
Antimicrobial activity was evaluated for a range of microorganisms by assessing the Minimum Inhibitory Concentration (MIC) by the broth microdilution method using 96-well microtiter plates. In continuation, the Minimum Bactericidal Concentration (MBC) was assessed by checking the viability using nutrient agar plates for bacteria and Sabouraud dextrose agar for the yeast, C. albicans. The disk diffusion assay was performed using Mueller-Hinton agar plates for bacteria and Sabouraud dextrose agar for yeast. Gram positive bacteria (Staphylococcus aureus MTCC 2940 and Bacillus subtilis NCIM 2063), Gram negative bacteria (Escherichia coli MTCC 1610, Vibrio cholerae MTCC 3904 and Salmonella typhi MTCC 3917) and yeast (Candida albicans MTCC 227) were the reference strains used for both the tests (MTCC = Microbial Type Culture Collection, Chandigarh, India and NCIM = National Collection of Industrial Microorganisms, Pune, India).
2.4.2. Anticancer studies.
2.4.2.1. MTT assay.
A miniaturized viability assay using 3-(4,5-di-methyithiazol-2-yl)-2,5-diphenyl-2H-tetrazolium bromide (MTT) was carried out with MCF-7 cells according to the method described in Mosmann, 1983.26 The gross morphological changes in the treated cells were observed in a phase contrast microscope (Carl Zeiss, India, Germany) and photographed. The cells were then assayed by addition of 20 μl of 5 mg μl−1 in phosphate-buffered saline (PBS). The plates were wrapped with aluminium foil and incubated for 4 h at 37 °C. The purple formazan product was dissolved by addition of 100 μl of 1% DMSO to each well. The absorbance was monitored at 570 nm (measurement) and 630 nm (reference) using a 96 well plate reader (Bio-rad, Hercules, CA, USA). Data were collected for duplicates and used to calculate the respective means.
2.4.2.2. AO/EB staining assay.
The MCF-7 cells were seeded in 6-well plates and allowed to reach 80% confluence. The cells were then treated with the IC50 concentration of compounds RuB-BPEI, RuP-BPEI, and RuD-BPEI and incubated for 24 h. The cells were trypsinized and pelleted and then suspended in PBS. A drop of cell suspension was placed on a glass slide and stained with AO (acridine orange) and EB (ethidium bromide) (1% in PBS, separately; mixed in a 1
:
1 ratio) (Sigma Chemical Co., St. Louis, MO, USA), and a cover slip was laid over it to reduce light diffraction. At random 100 cells were observed in a fluorescent microscope (Carl Zeiss, Jena, Germany) fitted with a 377–355 nm filter and examined at ×400 magnification.
3. Results and discussion
3.1. Synthesis and characterization of RuL-BPEI
The RuL-BPEI complexes were synthesized by the ligand substitution method in which one of the chloride ligands in [Ru(bpy/phen/2,9-dmp)2Cl2] was replaced by the amine group of BPEI.27–321H NMR spectra of the RuL-BPEI complexes show peaks in the aromatic region, which might be assigned to the aromatic ligands of ruthenium(II) chelates present in the polyethylenimine backbone. Methylene protons of BPEI gave broad signals between δ 2.4 and 3.7 ppm.
The UV-Visible absorption spectra of RuL-BPEI complexes clearly show intense ligand field transitions for RuB-BPEI at 291 nm, for RuP-BPEI at 266 nm and for RuD-BPEI at 269 nm and a band around 484 nm for RuB-BPEI, 466 nm for RuP-BPEI and 493 nm for RuD-BPEI, due to the Ru → N(σ) charge transfer (MLCT) transitions which are similar to ordinary known octahedral type ruthenium(II) complexes of the same nature as polymer bound complexes. In addition, the microanalysis of various elements (C, H, and N) and AES were used to work out the molecular formula. In the IR spectra of RuL-BPEI, BPEI shows N–H, C–H (sym. & asym.) and C–N stretching vibrations around 3427; 2854 & 2925 and 1060 cm−1, respectively. Furthermore, aromatic ring stretching frequencies of bpy, phen and 2,9-dmp ligands appeared around 1649 and 1476 cm−1 for ν(C
N) and ν(C
C), respectively. The ring vibrations observed for the ligands bpy, phen and 2,9-dmp around 852 cm−1, 817 cm−1 and 872 cm−1 are red shifted to around 778 cm−1, 772 cm−1 and 860 cm−1 in RuL-BPEI. These shifts can be explained by the donation of a lone pair of electrons from the polymeric amine to the central ruthenium ion resulting in the decrease of the electron pulling nature of the metal centre from the aromatic ring. This evidence clearly shows the formation of a coordination bond between the ruthenium centre and BPEI.
3.2. DNA binding studies
3.2.1. Electronic absorption spectroscopy.
In general the metal complexes can bind to DNA via both covalent and/or non-covalent interactions.33 Non-covalent interactions include intercalation between the bases, and binding to the minor groove, major groove, sugar–phosphate backbone and three way junction34,35 and the electrostatic binding mode.36 The binding efficiency of the ruthenium complex units of RuL-BPEI with CT-DNA was obtained through a change in the UV-Vis absorption spectra. The transition of bpy or phen or dmp ligands in the Ru(II) complex units in the presence of CT-DNA have shown shifts.37 And the intrinsic binding constant (Kb) of these ruthenium complex units with DNA was calculated according to the following equation | [DNA]/(εa − εf) = [DNA]/(εb − εf) + 1/Kb(εb − εf) | (1) |
where, [DNA] is the concentration of DNA. εa, εf, and εb correspond to the apparent extinction coefficient, the extinction coefficient for the unbound ruthenium complex units and its entirely DNA-bound combination, respectively. In the plots of [DNA]/(εa − εf) versus [DNA], Kb was given by the ratio of the slope to the intercept.
The interaction of the RuL-BPEI complexes with CT-DNA was studied and interpreted through the UV-visible absorption spectrophotometric titration method.33–36 The complexes show absorptions around 291, 266 and 269 nm respectively for RuB-BPEI, RuP-BPEI and RuD-BPEI, corresponding to aromatic ligand field transitions (shown in Fig. 1). Additionally, RuB-BPEI shows another peak around 244 nm in the π–π* region. On addition of CT DNA to RuB-BPEI, hypochromism was observed in the ligand field transitions and hyperchromism was observed in the π–π* region indicating operation of the electrostatic mode of interaction as the binding mode between this complex and DNA.38,39 For RuP-BPEI, hypochromism with a minor red shift was observed in the ligand field transition. This suggests that intercalation of this complex with DNA occurs involving a stacking interaction between the complex and DNA. In the case of RuD-BPEI, hypochromism with a second shoulder peak was observed in the ligand field transition region indicating an intercalative mode of binding between the complex and DNA. The binding constants (Kb) were calculated using eqn (1). For RuB-BPEIKb = 1.10 × 105 M−1, for RuP-BPEIKb = 1.63 × 106 M−1 and for RuD-BPEIKb = 1.12 × 104 M−1. These binding constants are comparable to that of simple ruthenium(II) complexes such as [Ru(bpy)3]2+ (1.4 × 106 M−1), Δ[Ru(phen)3]2+ (4.9 × 104 M−1), Λ[Ru(phen)3]2+ (2.8 × 104 M−1)40 and free BPEI.41 The low binding constant of RuD-BPEI is due to the presence of methyl groups in the 2,9-dmp ligand which decreased the binding strength through steric hindrance.
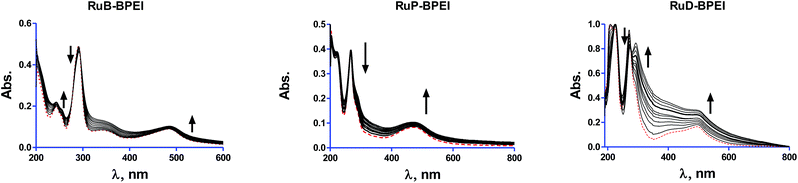 |
| Fig. 1 Absorption spectra of RuL-BPEI in the absence (dashed line) and in the presence (solid line) of a gradual addition of 10 μl up to 100 μl of [CT-DNA] = 2.00 × 10−3 M, 4.9 × 10−3 M and 9.8 × 10−3 M respectively. | |
Table 1 The degrees of coordination of RuL-BPEI
Compounds |
x
|
RuB-BPEI
|
0.0120 |
RuP-BPEI
|
0.0245 |
RuD-BPEI
|
0.0834 |
3.2.2. Fluorescence spectroscopy.
The complexes RuB-BPEI and RuP-BPEI are emissive between 630 and 700 nm when excited at 450 nm and the emission intensity increases in the presence of DNA (Fig. 2). This may be attributed to the fact that the Ru(II) complex moiety was protected by the hydrophobic DNA molecule which prevents quenching by water molecules thereby the emission intensity has increased. The fluorescence of the compound RuD-BPEI is very weak, so competitive binding studies were done using ethidium bromide as the fluorescent material for this compound. The emission intensity of EB–DNA was found to decrease on adding RuD-BPEI. The emission spectra of the DNA–EB system in the absence and the presence of RuD-BPEI are shown in Fig. 2. The decrease of the fluorescence intensity is due to the release of free EB molecules from the DNA–EB complex. Fluorescence quenching is explained by the Stern–Volmer equation:where F0 and F demonstrate the fluorescence intensities in the absence and in the presence of a quencher, respectively. Ksv is the Stern–Volmer constant and [Q] is the concentration of the quencher.42 The emission intensity of EB–DNA was found to decrease on adding RuD-BPEI. This emission spectra of the DNA–EB system in the absence and the presence of RuD-BPEI are shown in Fig. 2. The decrease of the fluorescence intensity is due to the release of free EB molecules from the DNA–EB complex. As usual, the Stern–Volmer equation was used to find out Ksv values for all the compounds which are tabulated in Table 2.
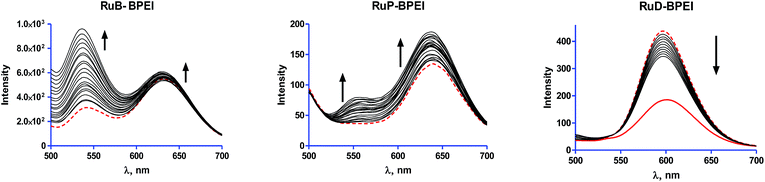 |
| Fig. 2 Emission spectra of RuB-BPEI and RuP-BPEI in the absence (dashed line) and in the presence (solid line) of DNA. [DNA] = 20 μM [ruthenium(II) complex units] = 0–3 mM. Emission spectra of EB bound DNA in the absence (dashed line) and in the presence (solid) of RuD-BPEI. [DNA] = 50 μM [EB] = 20 μM [ruthenium(II) complex units] = 0–3 mM. | |
Table 2 The Ksv values for RuL-BPEI complexesa
Compounds |
K
sv for the ruthenium(II) complex units L Mol−1 |
Free BPEI association constant 1.2 × 10−5 M−1 (ref. 41).
|
RuB-BPEI
|
1.89 × 102 |
RuP-BPEI
|
2.85 × 102 |
RuD-BPEI
|
2.62 × 102 |
3.2.4. Circular dichroism.
The circular dichroism spectral technique gave useful information on the interaction between CT-DNA and RuL-BPEI because DNA conformation has been changed by the adduct formation. Circular dichroism of the DNA solution was recorded over a range of 200–320 nm. The positive band intensity decreased on addition of RuL-BPEI complexes to CT-DNA and the negative band intensities of DNA gradually increased and, in particular, greatly increased in the presence of RuP-BPEI, indicating an increase in DNA helicity along with unfolding of the DNA structure on interaction of DNA with complexes (Fig. 4).
3.3. Antimicrobial susceptibility test
Both MIC and MBC were evaluated for the synthesized RuL-BPEI compounds (Table 3). The MIC of RuB-BPEI differs with the nature of the pathogens in the range of 200–500 μg ml−1. The lowest MIC value of 200 μg ml−1 was obtained in E. coli MTCC 1610 and S. aureus MTCC 2940 and the highest value of 500 μg ml−1 was obtained in B. subtilis NCIM 2063. However, the MBC values were uniformly 400 μg ml−1 except in the case of B. subtilis where no MBC could be obtained even when tested at a higher concentration of 700 μg ml−1. No inhibition could be obtained in the disk diffusion assay performed for B. subtilis while it was present for other pathogens which in turn was less than the reference antibiotics. In the RuP-BPEI and RuD-BPEI, MIC values ranges between 250 and 500 μg ml−1 and was highest in the case of B. subtilis NCIM 2063. However, the MBC values ranged between 300 and 500 μg ml−1 except in the case of B. subtilis where no MBC could be obtained even when tested at a higher concentration of 700 μg ml−1. As in RuL-BPEI, no inhibition could be obtained in the disk diffusion assay performed for B. subtilis while it was present for other pathogens which was less than the reference antibiotics. So, the antimicrobial ability of RuL-BPEI compounds differ with pathogens. RuB-BPEI was better for certain pathogens (E. coli MTCC 1610 and S. typhi MTCC 3917) while RuP-BPEI and RuD-BPEI were better for the rest (S. aureus MTCC 2940, V. Cholerae MTCC 3904 & C. albicans MTCC 227) but less efficacious than the antibiotics. The trends in the MIC, MBC and disk diffusion assay indicate that RuL-BPEI can find applications as a bacteriostatic agent against Gram positives (except B. subtilis), Gram negatives and fungi static against C. albicans.
Table 3 MIC, MBC values and zone of inhibition obtained for the synthesized compounds and reference antibiotics against the pathogenic microbesa
Compounds |
Methods |
Escherichia coli MTCC 1610 |
Staphylococcus aureus MTCC 2940 |
Salmonella typhi MTCC 3917 |
Vibrio cholerae MTCC 3904 |
Bacillus subtilis NCIM 2063 |
Candida albicans MTCC 227 |
In BPEI, the nil antimicrobial effect was recorded for polymer (BPEI) solution alone in most of the microorganisms. A good zone effect was observed in Candida albicans, whereas a lesser zone effect was detected against Salmonella typhi (see ESI).
|
RuB-BPEI
|
MIC value of the compound (μg ml−1) |
200 |
200 |
250 |
250 |
500 |
250 |
MBC value of the compound (μg ml−1) |
400 |
400 |
400 |
400 |
— |
400 |
Diameter of zone of inhibition (mm) of the compound at 500 μg per disk |
11 |
10 |
12 |
8 |
0 |
8 |
RuP-BPEI
|
MIC value of the compound (μg ml−1) |
250 |
250 |
300 |
250 |
500 |
250 |
MBC value of the compound (μg ml−1) |
300 |
400 |
500 |
350 |
— |
400 |
Diameter of zone of inhibition (mm) of the compound at 500 μg per disk |
12 |
12 |
15 |
10 |
0 |
8 |
RuD-BPEI
|
MIC value of the compound (μg ml−1) |
250 |
250 |
300 |
250 |
500 |
250 |
MBC value of the compound (μg ml−1) |
300 |
400 |
500 |
350 |
— |
400 |
Diameter of zone of inhibition (mm) of the compound at 500 μg per disk |
11 |
11 |
13 |
10 |
0 |
7 |
![[thin space (1/6-em)]](https://www.rsc.org/images/entities/char_2009.gif) |
Diameter of zone of inhibition (mm) of reference antibiotics (μg per disk, in parenthesis)
|
|
Amphicilin (10) |
16 |
17 |
10 |
22 |
15 |
26 |
|
Ciprofloxacin (5) |
48 |
34 |
31 |
46 |
32 |
30 |
|
Co trimoxazole (25) |
42 |
36 |
11 |
25 |
11 |
16 |
|
Amoxyclav (30) |
22 |
20 |
12 |
18 |
12 |
28 |
|
Nitrofurantoin (300) |
26 |
17 |
14 |
22 |
0 |
26 |
|
Norfloxacin (10) |
44 |
34 |
26 |
46 |
31 |
36 |
|
Fosfomycin (200) |
46 |
40 |
10 |
19 |
32 |
28 |
|
Amikacin (30) |
40 |
36 |
29 |
40 |
30 |
40 |
|
Gentamycin (10) |
40 |
40 |
29 |
44 |
26 |
34 |
|
Ceftriaxone (30) |
36 |
36 |
23 |
28 |
30 |
36 |
3.4. Cytotoxicity studies
RuL-BPEI was tested for its anticancer activity with MCF-7 cells which are suitable in vitro model systems for the study. A miniaturized viability assay was carried out using 3-(4,5-di-methyithiazol-2-yl)-2,5-diphenyl-2H-tetrazolium bromide (MTT) according to a literature method.26 The analytes, BPEI and RuL-BPEI, were dissolved in 1% DMSO, diluted in culture medium and used to treat the MCF-7 cells over a complex concentration of 10 to 100 μg ml−1 for a period of 24 h. 1% DMSO was used as the solvent control.
The percentage inhibition was calculated, from this data, using the formula:
The IC50 value was evaluated as the concentration of the compounds that is required to reduce the absorbance to half that of the control. This IC50 value in terms of ruthenium complex units for RuB-BPEI is 5.5241 × 10−7 mol dm−3, for RuP-BPEI is 1.0157 × 10−8 mol dm−3 and RuD-BPEI is 5.8394 × 10−6 mol dm−3 with MCF-7 cells indicating that, compared to BPEI45 and other mimic systems, the activity of the ruthenium complexes is modest46 and, in terms of ruthenium complex units, nearly shows that a lower concentration leads to better activity (see Table 4).
Table 4 MTT anti-proliferative assay IC50 of RuL-BPEI with MCF-7 breast cancer cellsa
Compounds |
IC50, for 24 h (μg ml−1) |
[Ru] based concentration (mol dm−3) |
Control: BPEI see ESI; IC50 of cis-platin is 0.98 ± 0.09 μM for the MCF-7 cell lines.47
|
RuB-BPEI
|
41 ± 0.5 |
5.52 × 10−7 |
RuP-BPEI
|
36 ± 0.5 |
1.02 × 10−8 |
RuD-BPEI
|
43 ± 0.5 |
5.84 × 10−6 |
3.5. Evaluation of apoptosis
To distinguish apoptosis, at a basic level, was done by AO/EB staining, to envisage and count the number of viable and apoptotic cells.48,49 After adding MCF-7 cells with the complexes at their respective IC50 concentrations for 24 h, the cells were assessed for gross cytological changes. These cytological changes showed that the cells were committed to cell death, mostly through apoptosis and to a certain extent necrosis. This method shows that the RuL-BPEI complexes act as good anticancer agents (Fig. 5).
4. Conclusion
Some ruthenium(II) complexes containing bpy or phen or dmp ligands anchored on a branched poly(ethyleneimine) molecule have been developed. The mode and strength of binding of the systems with CT DNA were evaluated through UV-Visible absorption, fluorescence, cyclic voltammetry, and circular dichroism methods. Among the systems the mode of binding of the bipyridine ligated complexes to CT DNA is electrostatic, while phenanthroline and dimethyl phenanthroline containing complexes bind to CT DNA through an intercalative mode. Circular dichroism studies show that the DNA structure unfolds along with increased helicity on binding with these compounds. The antimicrobial studies suggest that these polymer ruthenium(II) complexes can find applications as bacteriostatic agents against Gram positives (except B. subtilis), Gram negatives and fungistatic agents against C. albicans. The in vitro cytotoxicity study with MCF-7 cells demonstrates that all the compounds have good anticancer activity and good retention power. So these types of fluorescent polymer ruthenium(II) complexes are alternatives as better anti-cancer drugs.
Acknowledgements
We are grateful to the UGC-SAP and DST-FIST programmes of the department of chemistry, BDU. UGC-RFSMS is acknowledged for the financial support for JRF and SRF to I. Pradeep. One of the authors S. A. thanks the DST for sanction of a research scheme, Grant No. SR/S1/IC-13/2009, CSIR for the scheme, Grant No. 01(2461)/11/EMR-II and UGC for the scheme, Grant No. 41-223/2012(SR).
References
- J. K. Barton, J. Biomol. Struct. Dyn., 1983, 1, 621–632 CAS.
- C. V. Kumar, J. K. Barton and N. J. Turro, J. Am. Chem. Soc., 1985, 107, 5518–5523 CrossRef CAS.
- J. K. Barton, A. Danishefsky and J. Goldberg, J. Am. Chem. Soc., 1984, 106, 2172–2176 CrossRef CAS.
- C. G. Hartinger, S. Zorbas-Seifried, M. A. Jakupec, B. Kynast, H. Zorbas and B. K. Keppler, J. Inorg. Biochem., 2006, 100, 891–904 CrossRef CAS PubMed.
- E. Alessio, G. Mestroni, A. Bergamo and G. Sava, Curr. Top. Med. Chem., 2004, 4, 1525–1535 CrossRef CAS PubMed.
- S. Kapitza, M. Pongratz, M. A. Jakupec, P. Heffeter, W. Berger, L. Lackinger, B. K. Keppler and B. Marian, J. Cancer Res. Clin. Oncol., 2005, 131, 101–110 CrossRef CAS PubMed.
- S. Kapitza, M. A. Jakupec, M. Uhl, B. K. Keppler and B. Marian, Cancer Lett., 2005, 226, 115–121 CrossRef CAS PubMed.
- G. Sava, S. Zorzet, C. Turrin, F. Vita, M. Soranzo, G. Zabucchi, M. Cocchietto, A. Bergamo, S. DiGiovine, G. Pezzoni, L. Sartor and S. Garbisa, Clin. Cancer Res., 2003, 9, 1898–1905 CAS.
- A. Bergamo, S. Zorzet, B. Gava, A. Sorc, E. Alessio, E. Iengo and G. Sava, Anti-Cancer Drugs, 2000, 11, 665–672 CrossRef CAS PubMed.
- B. Cebrián-Losantos, A. A. Krokhin, I. N. Stepanenko, R. Eichinger, M. A. Jakupec, V. B. Arion and B. K. Keppler, Inorg. Chem., 2007, 46, 5023–5033 CrossRef PubMed.
-
A. Barca, B. Pani, M. Tamaro and E. Russo, Mutation Research/Fundamental and Molecular Mechanisms of Mutagenesis, Molecular interactions of ruthenium complexes in isolated mammalian nuclei and cytotoxicity on V79 cells in culture, 1999, 423, pp. 171–181 Search PubMed.
- J. Chen, L. Chen, S. Liao, K. Zheng and L. Ji, J. Phys. Chem. B, 2007, 111, 7862–7869 CrossRef CAS PubMed.
- E. E. M. Brouwers, M. M. Tibben, H. Rosing, J. H. M. Schellens and J. H. Beijnen, Rapid Commun. Mass Spectrom., 2007, 21, 1521–1530 CrossRef CAS PubMed.
- M. Groessl, E. Reisner, C. G. Hartinger, R. Eichinger, O. Semenova, A. R. Timerbaev, M. A. Jakupec, V. B. Arion and B. K. Keppler, J. Med. Chem., 2007, 50, 2185–2193 CrossRef CAS PubMed.
- P. Mura, M. Camalli, L. Messori, F. Piccioli, P. Zanello and M. Corsini, Inorg. Chem., 2004, 43, 3863 CrossRef CAS PubMed.
- A. V. Vargiu, A. Robertazzi, A. Magistrato, P. Ruggerone and P. Carloni, J. Phys. Chem. B, 2008, 112, 4401–4409 CrossRef CAS PubMed.
- A. R. Timerbaev, A. V. Rudnev, O. Semenova, C. G. Hartinger and B. K. Keppler, Anal. Biochem., 2005, 341, 326–333 CrossRef CAS PubMed.
- C. G. Hartinger, S. Hann, G. Koellensperger, M. Sulyok, M. Groessl, A. R. Timerbaev, A. V. Rudnev, G. Stingeder and B. K. Keppler, Int. J. Clin. Pharmacol. Ther., 2005, 43(12), 583–585 CrossRef CAS PubMed.
- M. Bacac, A. C. G. Hotze, K. V. D. Schilden, J. G. Haasnoot, S. Pacor, E. Alessio, G. Sava and J. Reedijk, J. Inorg. Biochem., 2004, 98, 402–412 CrossRef CAS PubMed.
- P. Schluga, C. G. Hartinger, A. Egger, E. Reisner, M. Galanski, M. A. Jakupec and B. K. Keppler, Dalton Trans., 2006, 1796–1802, 10.1039/b511792e.
- S. Rathinasamy, S. S. Karki, S. Bhattacharya, L. Manikandan, S. G. Prabakaran, M. Gupta and U. K. Mazumder, J. Enzyme Inhib. Med. Chem., 2006, 21, 501–507 CrossRef CAS PubMed.
- L. Mishra, A. K. Yadaw, S. Bhattacharya and S. K. Dubey, J. Inorg. Biochem., 2005, 99, 1113–1118 CrossRef CAS PubMed.
- V. Djinović, M. Momčilović, S. Grgurić-Šipka, V. Trajković, M. M. Stojković, D. Miljković and T. Sabo, J. Inorg. Biochem., 2004, 98, 2168–2173 CrossRef PubMed.
- A. C. G. Hotze, M. Bacac, A. H. Velders, B. A. J. Jansen, H. Kooijman, A. L. Spek, J. G. Haasnoot and J. Reedijk, J. Med. Chem., 2003, 46, 1743–1750 CrossRef CAS PubMed.
- B. P. Sullivan, D. J. Salmon and T. J. Meyer, Inorg. Chem., 1978, 17, 3334–3341 CrossRef CAS.
- T. Mosmann, J. Immunol. Methods, 1983, 65, 55–63 CrossRef CAS PubMed.
- R. S. Kumar and S. Arunachalam, Polyhedron, 2006, 25, 3113–3117 CrossRef CAS.
- R. S. Kumar, S. Arunachalam, V. S. Periasamy, C. P. Preethy, A. Riyasdeen and M. A. Akbarsha, Polyhedron, 2008, 27, 1111–1120 CrossRef CAS.
- J. Lakshmipraba, S. Arunachalam, D. A. Gandi and T. Thirunalasundari, Eur. J. Med. Chem., 2011, 46, 3013–3021 CrossRef CAS PubMed.
-
E. Tsuchida and H. Nishide, in Molecular Properties, Springer Berlin Heidelberg, 1977, vol. 24, ch. 1, pp. 1–87 Search PubMed.
- G. Vignesh, S. Arunachalam, S. Vignesh and R. A. James, Spectrochim. Acta, Part A, 2012, 96, 108–116 CrossRef CAS PubMed.
- G. Vignesh, R. Senthilkumar, P. Paul, V. S. Periasamy, M. A. Akbarsha and S. Arunachalam, RSC Adv., 2014, 4, 57483–57492 RSC.
- Q.-L. Zhang, J.-G. Liu, H. Chao, G.-Q. Xue and L.-N. Ji, J. Inorg. Biochem., 2001, 83, 49–55 CrossRef CAS PubMed.
- A. Oleksi, A. G. Blanco, R. Boer, I. Usón, J. Aymamí, A. Rodger, M. J. Hannon and M. Coll, Angew. Chem., Int. Ed., 2006, 45, 1227–1231 CrossRef CAS PubMed.
- R. Indumathy, M. Kanthimathi, T. Weyhermuller and B. U. Nair, Polyhedron, 2008, 27, 3443–3450 CrossRef CAS.
- F. Arjmand, M. Muddassir and R. H. Khan, Eur. J. Med. Chem., 2010, 45, 3549–3557 CrossRef CAS PubMed.
- R. S. Kumar, S. Arunachalam, V. S. Periasamy, C. P. Preethy, A. Riyasdeen and M. A. Akbarsha, Eur. J. Med. Chem., 2008, 43, 2082–2091 CrossRef CAS PubMed.
- J. Han, S. Kim, T.-S. Cho and J.-C. Lee, Macromol.
Res., 2004, 12, 276–281 CAS.
- M. S. Mohamed, A. A. Shoukry and A. G. Ali, Spectrochim. Acta, Part A, 2012, 86, 562–570 CrossRef CAS PubMed.
- S. Satyanarayana, J. C. Dabrowiak and J. B. Chaires, Biochemistry, 1992, 31, 9319–9324 CrossRef CAS PubMed.
- Y.-L. Zhou and Y.-Z. Li, Spectrochim. Acta, Part A, 2004, 60, 377–384 CrossRef.
- K. S. Ghosh, B. K. Sahoo, D. Jana and S. Dasgupta, J. Inorg. Biochem., 2008, 102, 1711–1718 CrossRef CAS PubMed.
- S.-S. Zhang, S.-Y. Niu, B. Qu, G.-F. Jie, H. Xu and C.-F. Ding, J. Inorg. Biochem., 2005, 99, 2340–2347 CrossRef CAS PubMed.
- M. T. Carter, M. Rodriguez and A. J. Bard, J. Am. Chem. Soc., 1989, 111, 8901–8911 CrossRef CAS.
- Y. Liu, D.-C. Wu, W.-D. Zhang, X. Jiang, C.-B. He, T. S. Chung, S. H. Goh and K. W. Leong, Angew. Chem., Int. Ed., 2005, 44, 4782–4785 CrossRef CAS PubMed.
- X. Li, A. K. Gorle, T. D. Ainsworth, K. Heimann, C. E. Woodward, J. Grant Collins and F. Richard Keene, Dalton Trans., 2015, 44, 3594–3603 RSC.
- R. M. Lord, A. J. Hebden, C. M. Pask, I. R. Henderson, S. J. Allison, S. L. Shepherd, R. M. Phillips and P. C. McGowan, J. Med. Chem., 2015, 58, 4940–4953 CrossRef CAS PubMed.
- W. C. Earnshaw, L. M. Martins and S. H. Kaufmann, Annu. Rev. Biochem., 1999, 68, 383–424 CrossRef CAS PubMed.
- G. Häcker, Cell Tissue Res., 2000, 301, 5–17 CrossRef.
Footnote |
† Electronic supplementary information (ESI) available: Characterisation, in vitro study images. See DOI: 10.1039/c6ra00692b |
|
This journal is © The Royal Society of Chemistry 2016 |