DOI:
10.1039/C5RA27014F
(Paper)
RSC Adv., 2016,
6, 12647-12651
Photoswitchable aggregation-induced emission polymer containing dithienylethene and tetraphenylethene moieties†
Received
17th December 2015
, Accepted 20th January 2016
First published on 25th January 2016
Abstract
A photochromic aggregation-induced emission (AIE) active polymer containing dithienylethene (DTE) and tetraphenylethene (TPE) moieties was synthesized through a nucleophilic substitution reaction between 1,2-bis[5′-(4′′-hydroxyphenyl)-2′-ethylthien-3′-yl]perfluorocyclopentene and 1,2-bis[4-(bromomethyl)phenyl]-1,2-diphenylethene. The prepared polymer is soluble in various organic solvents and can be easily processed into thin film. The DTE chromophores in the polymer can undergo reversible photoisomerization between their open and closed forms upon irradiation with UV and visible light. Thus, the fluorescence of the prepared AIE polymer can be photoswitched.
Introduction
In recent years, tunable luminescent materials responding to external stimulus have attracted much attention for their potential applications in sensors, optical recording devices and others. To date, several kinds of stimulus responsive luminescent materials have been reported, such as deformation-induced chromic, thermochromic, photochromic, and many others luminescent systems.1–7 Among these, photochromic fluorescent materials have attracted increasing interest for their potential applications.8–16 Light can be manipulated very precisely and easily, these photoswitched material systems can be used for the optical writing and reading of data, super high-resolution fluorescence images as well as luminescent probes for bioimaging applications. Due to the excellent photoresponsibility, high thermal stability and fatigue resistance, dithienylethene (DTE) has been one of the most attractive photochromic molecules, which was developed by Irie and coworkers.17,18 The physicochemical properties, such as dielectric constant, refractive index, and redox of the two isomeric structures of DTE molecules are very different. Based on these properties, DTEs have been widely used as molecular switches to modulate the chirality, conductivity and self-assembly properties.19–26 Recently, the fluorescent DTE molecules, in which a fluorescent chromophore is linked to a DTE unit, have been well developed for their high on/off ratios and switching speeds.7,27–31 In most cases, the excited energy of the fluorescent chromophore part can be intramolecularly transferred to the photogenerated closed-ring isomer of DTE part, so the fluorescent state will be well quenched.
In the solid or aggregate state, the aggregation of the fluorophores caused by the strong intermolecular π–π interaction or intermolecular hydrogen bonding will debase or quench the emission (ACQ). Anyway, the solid or aggregate state will be often required for the optoelectronic applications of photoswitch. Tang and coworkers have reported the aggregation-induced emission (AIE) phenomenon, which offers a new path to solve the ACQ problem. Series of AIE active molecules have been synthesized.32–39 Among the AIE fluorophores, the tetraphenylethene (TPE) system has received much attention for its facile preparation, ready functionalization, and high photoluminescence efficiency. Up to date, a few photochromic AIE organic molecules have been reported.40,41 However, to use such low molecular weight materials in practical applications, the first method is to fabricate solid films by expensive vacuum vapour deposition process, the other method is to prepare films using doped polymer matrix, while the aggregation tendency of the guest molecules in the polymer host will be a general problem. One way to overcome this processing disadvantage is to synthesize polymers with relative high molecular weights, which can be easily fabricated into large-area films just by spin coating method.42–44
In this paper, we report the synthesis of a new photochromic AIE active polymer containing DTE and TPE moieties through polycondensation reaction. The DTE chromophores in the polymer could undergo photoisomerization between their open and closed forms when irradiated with UV and visible light respectively. Thus, the fluorescence of the prepared AIE polymer can be photoswitched both in aggregate state and in the solid film.
Experimental section
Materials and characterization
Tetrahydrofuran (THF) was purified by distillation with sodium and benzophenone under nitrogen. Ultrapure water (resistivity > 18 MΩ cm) was obtained from a Milli-Q water purification system and used for all experiments. All other reagents and solvents were purchased commercially and used without further purification.
1H NMR spectra and 13C NMR spectra were recorded on a JEOL JNM-ECA 600 spectrometers by using acetone-d6 or CDCl3 as the solvent. Relative molecular weights and molecular weight distributions were measured on a gel permeation chromatography (GPC) apparatus using THF as eluent at a flow rate of 1.0 mL min−1. The instrument was equipped with a refractive index detector and fitted with a PL gel 5 μm mixed-D column, which was calibrated by using linear polystyrene standards. Photoirradiation was carried out using a 300 W Compact-xenon lamp (Asahi Max-302) with different bandpass filter as light source. UV-Vis spectra were recorded on an Agilent Cary 300 UV-Vis spectrophotometer. Fluorescence spectra were recorded on a Hitachi F-4500 fluorescence spectrophotometer. High-resolution mass data were obtained with a Shimadzu LCMS-IT/TOF instrument. The aggregates morphologies were examined by TEM (H-7650B, HITACHI) with an accelerating voltage of 80–120 kV.
Synthesis of monomers and polymer
Synthesis of 1,2-bis[5′-(4′′-methoxyphenyl)-2′-ethylthien-3′-yl] perfluorocyclopentene (DTE-2OMe). To a anhydrous THF (30 mL) solution of 3-bromo-2-ethyl-5-(4-methoxyphenyl)thiophene24 (1.5 g, 5 mmol) was added 3.4 mL n-BuLi (1.6 M in a hexane solution, 5.44 mmol) at −78 °C under Ar. After 1 h, perfluorocyclopentene (0.53 g, 2.5 mmol) was added to the reaction mixture, which was then further stirred at −78 °C for 1 h and allowed to warm to 25 °C. The mixture was poured into water and extracted with ether. The combined organic extract was washed with water, dried over anhydrous MgSO4, filtered and evaporated under a reduced pressure. Then the residue was purified by silica gel column using petroleum ether/CH2Cl2 (4
:
1 by volume) to allow isolation of DTE-2OMe in 70% yield. 1H NMR (600 MHz, acetone-d6, δ): 0.96 (t, 6H), 2.45 (q, 4H), 3.83 (s, 6H), 6.98 (d, 4H), 7.32 (s, 2H), 7.58 (d, 4H), 13C NMR (150 MHz, acetone-d6, δ): 160.5, 148.6, 143.3, 127.6, 126.7, 124.8, 122.0, 115.2, 55.7, 23.0, 15.9. HRMS MS+: calcd for C31H26F6O2S2 608.1273, found 608.1272.
Synthesis of 1,2-bis[5′-(4′′-hydroxyphenyl)-2′-ethylthien-3′yl] perfluorocyclopentene (DTE-2OH). BBr3 (0.6 mL) in dichloromethane (3 mL) was added dropwise slowly into DTE-2OMe (0.61 g, 1 mmol) dissolved in dichloromethane (20 mL) under Ar at 0 °C. After reaction for 8 h at room temperature and then quenched by methanol, the mixture was poured into an excess of water and extracted from the water using dichloromethane (100 mL). After desiccating and concentrating the organic phase, the residue was purified by silica gel column using CH2Cl2/ethyl acetate (20
:
1 by volume) to allow isolation of DTE-2OH in 95% yield. 1H NMR (600 MHz, CDCl3, δ): 0.96 (t, 6H), 2.31 (q, 4H), 6.85 (d, 4H), 7.10 (s, 2H), 7.43 (d, 4H), 13C NMR (150 MHz, CDCl3, δ): 155.6, 148.2, 142.2, 127.3, 126.7, 124.4, 121.3, 116.0, 22.6, 15.5. HRMS [M − H]−: calcd for C29H22F6O2S2 579.0893, found 579.0892.
Synthesis of 1,2-bis[4-(bromomethyl)phenyl]-1,2-diphenylethene (TPE-2Br). 1,2-Bis[4-(bromomethyl)phenyl]-1,2-diphenylethene had been synthesized according to the previous report.42 Into a 250 mL round-bottom flask were added 1,2-bis(4-methylphenyl)-1,2-diphenylethene (1.0 g, 2.77 mmol), freshly recrystallized N-bromosuccinimide (1.08 g, 6.094 mmol), and catalytic amount of benzoyl peroxide (0.027 g, 0.11 mmol) in 20 mL of carbon tetrachloride. The solution was refluxed for 10 h. After cooled to room temperature, the solution was filtered and the filtrate was concentrated. The crude product was purified by a silica gel column using petroleum ether/CH2Cl2 (5
:
1 by volume) as eluent. A white solid was obtained in 40% yield (0.6 g). 1H NMR (600 MHz, CDCl3, δ): 4.40 (m, 4H), 6.98 (m, 8H), 7.11 (m, 10H), 13C NMR (150 MHz, CDCl3, δ): 143.9, 143.3, 140.9, 136.0, 131.8, 131.5, 128.7, 128.0, 126.8, 33.8. HRMS [M + K]+: calcd for C28H22Br2 556.9701, found 556.9703.
Photochromic AIE active polymer. TPE-2Br (0.1 g, 0.193 mmol), DTE-2OH (0.112 g, 0.193 mmol), K2CO3 (0.267 g, 1.93 mmol), KI (0.1 g) and DMF (5 mL) were added into a vacuum-evacuated, argon-filled 25 mL two-necked round-bottomed flask and kept at 85 °C for 48 hours. Then the solution was poured into plenty of water and the precipitate was collected and dried. The product was dissolved in THF and precipitated into plenty of petroleum ether. The final product was vacuum dried at 60 °C for 24 h. Mn (GPC) = 9000 and PDI = 2.2.
Preparation of polymer aggregates. A stock solution of the prepared polymer was prepared in THF with a concentration of 1 mg mL−1. Aliquots of the stock solution were transferred to 10 mL volumetric flasks, into which appropriate volumes of THF and water were added dropwise under vigorous stirring. The water contents of the final solutions were changed from 0 to 90 vol%. UV and photoluminescence spectra were immediately measured once the solutions were prepared.
Preparation of polymer film. The homogeneous solution of polymer in anhydrous N,N-dimethylformamide (DMF) were filtered through 0.45 μm membranes and spin-coated onto quartz glass slides. The spin-coated films were dried at 70 °C under vacuum for 48 h and were stored in a desiccator for further measurements.
Results and discussion
Synthesis and characterization
The synthetic procedure for the open form AIE active polymer is shown in the Scheme 1. DTE-2OMe was first prepared by the coupling reaction between 3-bromo-2-ethyl-5-(4-methoxyphenyl) thiophene and perfluorocyclopentene. Then the monomer DTE-2OH was synthesized by the demethylation of DTE-2OMe using boron tribromide in dichloromethane. TPE-2Br was synthesized by bromination of 1,2-bis(4-methylphenyl)-1,2-diphenylethene, which was prepared by the McMurry homo-coupling of 4-methylbenzophenone in the presence of TiCl4 and zinc powder in tetrahydrofuran. Then, the AIE active polymer was prepared through nucleophilic substitution reaction between DTE-2OH and TPE-2Br in DMF using K2CO3 and KI as HBr absorbent and catalyst, respectively. The obtained polymer shows good solubility in common organic solvents, such as CHCl3, THF, and DMF.
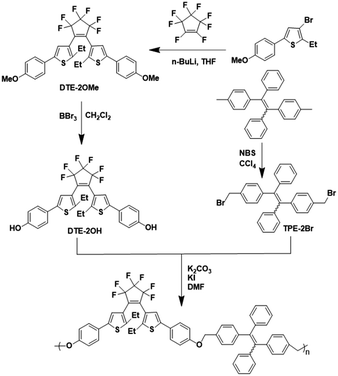 |
| Scheme 1 Synthetic route of the photochromic AIE active polymer. | |
The 1H NMR spectra of the polymer as well as the monomers (DTE-2OH and TPE-2Br) in CDCl3 are shown in the provided ESI (Fig. S1†). It can be seen that the resonance of –CH2– protons near the TPE part at δ 4.40 (peak 6) have shifted to lower magnetic field (δ 4.98, peak f) after the nucleophilic substitution reaction. The number-average molar mass (Mn) and PDI are about 9000 and 2.2, respectively, which are determined by GPC measurement provided in ESI (Fig. S2†).
Photochromic properties
The photochromic behavior of the polymer was studied by UV-Vis absorption spectroscopy. The UV-Vis spectra of the photochromic polymer in solutions and as solid film were recorded over different irradiation time intervals until photostationary states were reached. The UV-Vis spectra of the polymer in THF varying with irradiation time are shown in Fig. 1a and b.
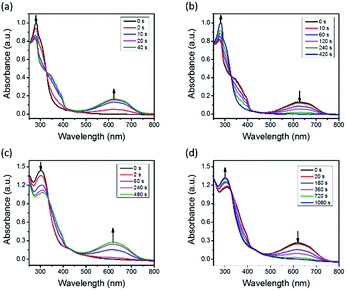 |
| Fig. 1 UV-Vis spectra of AIE polymer (a) in THF (10−5 M), DTE in open form upon irradiation with 300 nm UV light, (b) in THF (10−5 M), DTE in closed form upon irradiation with 620 nm visible light, (c) as solid film, DTE in open form upon irradiation with 300 nm UV light, (d) as solid film, DTE in closed form upon irradiation with 620 nm visible light. | |
The absorption maximum of the polymer in THF appears at about 283 nm. Upon irradiation with UV light (λ = 300 nm, 0.14 mW cm−2), the color of the THF solution (10−5 M) gradually turns to blue. It corresponds the new absorption band with maximum at 622 nm, which indicates the formation of the closed-ring isomer of DTE chromophores in the polymer. After about 40 s irradiation with 300 nm UV light, the photostationary state of the THF solution is reached. The maximum absorbance at 622 nm during UV light irradiation can be fitted to the first-order exponential decay function (Fig. 2a). Then the blue THF solution is irradiated with visible light (λ = 620 nm, 0.9 mW cm−2), it turns back to the colorless state, which means that the DTE chromophores changes from the closed-ring isomer to its open-ring counterpart. After several minutes of irradiation by visible light, the absorption band with maximum at 622 nm will totally disappear (Fig. 1b). The maximum absorbance at 622 nm during visible light irradiation can be fitted to the first-order exponential decay function (Fig. 2b). The DTE containing polymer shows obvious photochromic properties in other solvents such as CHCl3 and DMF (Fig. S3 and S4†). The photochromic behavior of the polymer in solid state was also studied. The DTE chromophores in the polymer film can also undergo photoisomerization between their open and closed forms when irradiate with UV and visible light. The UV-Vis spectra changes of the polymer film upon irradiation with UV and visible light are shown in Fig. 1c and d. The results are similar with that of in solutions. The maximum absorbance at 616 nm during UV and visible light irradiation can also be fitted to the first-order exponential decay function (Fig. 2c and d).
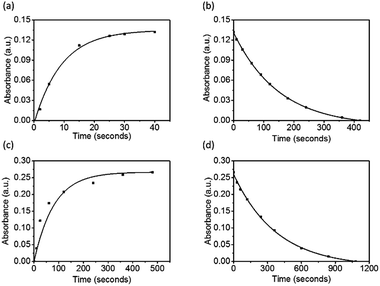 |
| Fig. 2 Maximum absorbance (622 nm for THF solution, 616 nm for solid film) of DTE chromophores of the polymer recorded over different irradiation time intervals (a) in THF (10−5 M) with 300 nm UV light irradiation, (b) then the photostationary state THF solution irradiated with 620 nm visible light, (c) as solid film with 300 nm UV light irradiation, (d) then the photostationary state film irradiated with 620 nm visible light. | |
Photoswitchable aggregation-induced emission properties
The photoluminescence behaviors of the polymer in the solution, aggregate state and solid film were studied. The aggregates were obtained by adding water into their THF solutions under vigorous stirring. When the diluted THF solution was excited with 380 nm light, it can be seen from the fluorescence spectrum that no emission can be detected in the molecularly dissolved state. Then a large amount of water was added into the THF solution, an intense photoluminescence peak (about 497 nm) was recorded under the same measurement condition.
It can be seen from Fig. 3a and b that the fluorescence intensity gradually increases as the water fraction increases. As water is a poor solvent for the polymer, the polymeric chains will aggregate in the THF/water mixtures with high water contents. Then, the prepared polymer is induced to emit by aggregate formation. Fig. 4 gives the TEM image of the formed aggregates in THF–water mixture (90% water content). It can be seen that spherical particles have been formed in the mixed solvents. The diameter of the formed aggregate particle is about a few hundred nanometers. Different solvents systems were also used to study the AIE properties such as DMF–water and CHCl3–hexane mixtures (Fig. S5†). It can be seen that the solvents had much effect on the fluorescence spectra of the AIE polymer. Compared with that in THF–water mixture, red-shifts and blue-shifts in emission spectra are found in the DMF–water and CHCl3–hexane mixture, respectively. From the above results, it can be concluded that the phenyl rings of TPE can still rotate in the solution, although the TPE unit has been incorporated into the main chain structure. Anyway, the intramolecular rotation in the aggregate state is restricted, which leads to the light emission. By using 9,10-diphenyanthracene as a standard, the fluorescence quantum yields (ΦF) of the polymer in THF solution and aggregated form (90% water content) were estimated to be approximately 0.024% and 2.4% respectively.
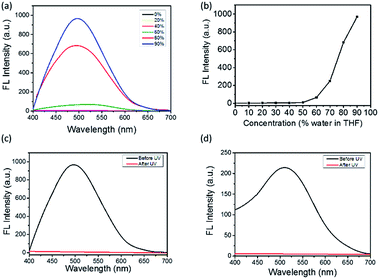 |
| Fig. 3 (a) Fluorescence spectra of the AIE polymer in different THF– water solvent mixtures (excitation at 380 nm), (b) relationship between maximum emission intensity (at 497 nm) of the aggregates in THF–water solvent mixtures and the water content, (c) fluorescence spectra (excitation at 380 nm) of the polymer aggregates in THF–water solvent mixtures (90% water content) before and after irradiation with UV light at 300 nm, (d) fluorescence spectra (excitation at 380 nm) of the polymer film before and after irradiation with UV light at 300 nm. | |
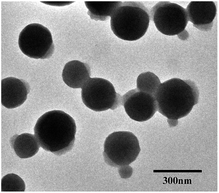 |
| Fig. 4 Typical TEM images of the aggregates of the prepared AIE polymer in THF–water mixtures (90% water content). | |
The maximum FL intensity at emission 497 nm (in THF–water mixture) at every water fraction is showed in Fig. 3b. Compared to THF/water mixtures, obvious 10 nm red-shifts in emission spectra is found for the polymer film (maximum at 507 nm in Fig. 3d).
As mentioned in Fig. 1, the open form DTE can change to its closed-ring isomer after irradiation with UV light, thus the excited energy of the fluorescent chromophore TPE can be intramolecularly transferred to the photogenerated closed-ring isomer and the fluorescent state will be quenched. Fig. 3c and d give the fluorescence spectra of the polymer before and after irradiated by 300 nm UV light in aggregate state and solid film, respectively. It can be seen that the polymer in both aggregate state and solid film shows obvious fluorescence (excitation at 380 nm) before irradiation with 300 nm light, while the fluorescence from the TPE moiety is totally quenched after the 300 nm UV light irradiation.
When the closed-ring DTEs in the polymer change back to open form by irradiation with 620 nm visible light, the obvious fluorescence can be detected. Fig. 5a gives the fluorescence spectra of the polymer film after irradiation with UV and visible light, respectively. It can be seen that the obvious fluorescence can be found again after the visible light irradiation. Due to the reversible photoisomerization of DTE chromophores between their open and closed forms upon irradiation with UV and visible light, the fluorescence of the polymer thus can be photoswitched repeatedly (Fig. 5b).
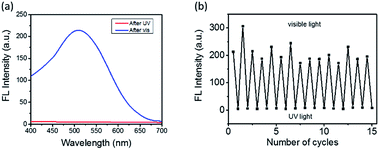 |
| Fig. 5 (a) Reversible fluorescence switching of polymer film (excitation 380 nm) using UV light and visible light, (b) cycling behaviour fluorescence switching of polymer film (excitation 380 nm, emission 507 nm) using UV light and visible light. | |
Conclusions
A photochromic aggregation-induced emission (AIE) active polymer, which was composed of dithienylethene and tetraphenylethene moieties, was synthesized through nucleophilic substitution reaction. Upon irradiation with UV and visible light, the DTE chromophores in the polymer can undergo photoisomerization between their open and closed forms. Thus, the fluorescence of the prepared AIE polymer can be photoswitched.
Acknowledgements
This project is supported by Natural Science Foundation of China (Grant No. 21474056).
Notes and references
- N. Chandrasekharan and L. A. Kelly, J. Am. Chem. Soc., 2001, 123, 9898 CrossRef CAS PubMed.
- T. Mutai, H. Tomoda, T. Ohkawa, Y. Yabe and K. Araki, Angew. Chem., Int. Ed., 2008, 47, 9522 CrossRef CAS PubMed.
- J. Kunzelman, M. Kinami, B. R. Crenshaw, J. D. Protasiewicz and C. Weder, Adv. Mater., 2008, 20, 119 CrossRef CAS.
- C. Lowe and C. Weder, Adv. Mater., 2002, 14, 1625 CrossRef CAS.
- T. Mutai, H. Satou and K. Araki, Nat. Mater., 2005, 4, 685 CrossRef CAS PubMed.
- D. Yan, J. Lu, J. Ma, M. Wei, D. G. Evans and X. Duan, Angew. Chem., Int. Ed., 2011, 50, 720 CrossRef CAS PubMed.
- M. Irie, T. Fukaminato, T. Sasaki, N. Tamai and T. Kawai, Nature, 2002, 420, 759 CrossRef CAS PubMed.
- F. M. Raymo and M. Tomasulo, J. Phys. Chem. A, 2005, 109, 7343 CrossRef CAS PubMed.
- Z. Tian, W. Wu and A. D. Q. Li, ChemPhysChem, 2009, 10, 2577 CrossRef CAS PubMed.
- T. Fukaminato, J. Photochem. Photobiol., C, 2011, 12, 177 CrossRef CAS.
- I. Yildiz, E. Deniz and F. M. Raymo, Chem. Soc. Rev., 2009, 38, 1859 RSC.
- H. Tian and S. Yang, Chem. Soc. Rev., 2004, 33, 85 RSC.
- P. Belser, L. De Cola, F. Hartl, V. Adamo, B. Bozic, Y. Chriqui, V. M. Iyer, R. T. F. Jukes, J. Kühni, M. Querol, S. Roma and N. Salluce, Adv. Funct. Mater., 2006, 16, 195 CrossRef CAS.
- T. Fukaminato, E. Tateyama and N. Tamaoki, Chem. Commun., 2012, 48, 10874 RSC.
- G. Copley, J. G. Gillmore, J. Crisman, G. Kodis, C. L. Gray, B. R. Cherry, B. D. Sherman, P. A. Liddell, M. M. Paquette, L. Kelbauskas, N. L. Frank, A. L. Moore, T. A. Moore and D. Gust, J. Am. Chem. Soc., 2014, 136, 11994 CrossRef CAS PubMed.
- E. Deniz, M. Tomasulo, J. Cusido, S. Sortino and F. M. Raymo, Langmuir, 2011, 27, 11773 CrossRef CAS PubMed.
- M. Irie and M. Mohri, J. Org. Chem., 1998, 53, 803 CrossRef.
- M. Irie, Chem. Rev., 2000, 100, 1685 CrossRef CAS PubMed.
- F. Luo, B. Chen, Z. Wang and H. Tian, Adv. Funct. Mater., 2003, 13, 223 CrossRef.
- G. M. Tsivgoulis and J. M. Lehn, Angew. Chem., Int. Ed., 1995, 34, 1119 CrossRef CAS.
- T. B. Norsten and N. R. Branda, J. Am. Chem. Soc., 2001, 123, 1784 CrossRef CAS PubMed.
- Y. Zou, T. Yi, Z. Xiao, Y. Li, Y. Li, X. Gao, C. Wu, M. Yu and H. Huang, J. Am. Chem. Soc., 2008, 130, 15750 CrossRef CAS PubMed.
- N. Katsonis, T. Kudernac, M. Walko, S. J. van der Molen, B. J. van Wees and B. L. Feringa, Adv. Mater., 2006, 18, 1397 CrossRef CAS.
- Y. He, Y. Yamamoto, W. Jin, T. Fukushima, A. Saeki, S. Seki, N. Ishii and T. Aida, Adv. Mater., 2010, 22, 829 CrossRef CAS PubMed.
- Y. He, Y. Zhu, Z. Chen, W. He and X. Wang, Chem. Commun., 2013, 49, 5556 RSC.
- Z. Chen, Y. He and X. Wang, Macromol. Rapid Commun., 2011, 32, 977 CrossRef CAS PubMed.
- C. Yun, J. You, J. Kim, J. Huh and E. Kim, J. Photochem. Photobiol., C, 2009, 10, 111 CrossRef CAS.
- T. Fukaminato and M. Irie, Adv. Mater., 2006, 18, 3225 CrossRef CAS.
- R. Metivier, S. Badre, R. Meallet-Renault, P. Yu, R. B. Pansu and K. Nakatani, J. Phys. Chem. C, 2009, 113, 11916 CAS.
- T. Fukaminato, T. Sasaki, T. Kawai, N. Tamai and M. Irie, J. Am. Chem. Soc., 2004, 126, 14843 CrossRef CAS PubMed.
- C. Li, H. Yan, L. Zhao, G. Zhang, Z. Hu, Z. Huang and M. Zhu, Nat. Commun., 2014, 5, 5709 CrossRef CAS PubMed.
- J. Luo, Z. Xie, J. W. Y. Lam, L. Cheng, H. Chen, C. Qiu, H. S. Kwok, X. Zhan, Y. Liu, D. Zhu and B. Z. Tang, Chem. Commun., 2001, 18, 1740 RSC.
- Y. Hong, J. W. Y. Lam and B. Z. Tang, Chem. Soc. Rev., 2011, 40, 536 RSC.
- Y. Hong, J. W. Y. Lam and B. Z. Tang, Chem. Commun., 2009, 29, 4332 RSC.
- A. Qin, J. W. Y. Lam and B. Z. Tang, Prog. Polym. Sci., 2012, 37, 182 CrossRef CAS.
- A. Rananaware, R. S. Bhosale, H. Patil, M. A. Kobaisi, A. Abraham, R. Shukla, S. V. Bhosale and S. V. Bhosale, RSC Adv., 2014, 4, 59078 RSC.
- A. Rananaware, D. Duc La and S. V. Bhosale, RSC Adv., 2015, 5, 63130 RSC.
- A. Rananaware, D. Duc La and S. V. Bhosale, RSC Adv., 2015, 5, 56270 RSC.
- A. Rananaware, R. S. Bhosale, K. Ohkubo, H. Patil, L. A. Jones, S. L. Jackson, S. Fukuzumi, S. V. Bhosale and S. V. Bhosale, J. Org. Chem., 2015, 80, 3832 CrossRef CAS PubMed.
- S. Lim, B. An, S. D. Jung, M. Chung and S. Y. Park, Angew. Chem., Int. Ed., 2004, 43, 6346 CrossRef CAS PubMed.
- C. Li, W. Gong, Z. Hu, M. P. Aldred, G. Zhang, T. chen, Z. Huang and M. Zhu, RSC Adv., 2013, 3, 8967 RSC.
- A. Qin, L. Tang, J. W. Y. Lam, C. K. W. Jim, Y. Yu, H. Zhao, J. Sun and B. Z. Tang, Adv. Funct. Mater., 2009, 19, 1891 CrossRef CAS.
- H. Li, H. Wu, E. Zhao, J. Li, J. Sun, A. Qin and B. Z. Tang, Macromolecules, 2013, 46, 3907 CrossRef CAS.
- R. Hu, N. L. C. Leung and B. Z. Tang, Chem. Soc. Rev., 2014, 43, 4494 RSC.
Footnote |
† Electronic supplementary information (ESI) available. See DOI: 10.1039/c5ra27014f |
|
This journal is © The Royal Society of Chemistry 2016 |