DOI:
10.1039/C5RA26313A
(Communication)
RSC Adv., 2016,
6, 12093-12099
Facile growth of Bi2MoO6 nanosheet arrays on Ni foam as an electrode for electrochemical applications
Received
9th December 2015
, Accepted 18th January 2016
First published on 21st January 2016
Abstract
A facile hydrothermal reaction has been developed for the large-scale growth of Bi2MoO6 nanosheets on conductive substrates with robust adhesion as high performance electrodes for electrochemical capacitors. It was found that the nickel foam facilitates the formation of a uniform nanosheet array with fast electron and ion transportation, a large electroactive surface area and an excellent structural stability. As a result, it shows a specific capacitance of 37.3 F g−1 even at a current density of 2 A g−1 and a high cycling stability with 89% retention of its initial specific capacitance after 1000 cycles in 1 M KCl solution. These results provide a way of fabricating Bi2MoO6 nanosheet arrays as efficient electrodes for electrochemical capacitors.
1. Introduction
Supercapacitors with higher power densities and longer cycle lives have drawn considerable attention over the past few decades due to the increasing energy demands and environmental issues.1–3 Up to now, numerous nanomaterials4–8 with high specific surface areas have been investigated with improved charge accumulation and ion transportation for electrochemical utilization. For example, graphene,9,10 possessing a two dimensional structure, enables ultrahigh charging–discharging of an electric capacitor. Besides, the fabrication of interesting nanoarchitectures is also considered as an effective method to boost the electrochemical activities.
Recently, the rational design of nanosheet,11–13 nanowire14–17 and nanorod arrays18,19 directly on substrates has been confirmed as a great achievement in electrochemical (EC) research. It avoids the dead surface in a traditional slurry derived electrode and allows for more efficient charge exchange during the electrochemical process. In particular, the nanosheet array has attracted wide interest because of the large surface area and good electric transmission. Cheng and co-workers20 prepared Ni–Mo oxide nanosheet arrays on Ni foam with a high EC performance. Xiao et al.21 obtained NiO nanosheet networks supported on Ni foams as high rate supercapacitor electrodes. Liu et al. synthesized mesoporous MnO2 nanosheet arrays on Ni foam with improved capacitance.22
Bismuth molybdate (Bi2MoO6) with an Aurivillius type structure23 is a promising material that is widely used in photocatalytic applications due to its unique optical and electric properties. Moreover, it has been studied as a good electrode material for supercapacitors. As reported, Bi2MoO6 nanosheets24 yield a capacitance of 25 F g−1 at 5 mV s−1 in 1 M NaCl electrolyte. However, its EC properties need to be improved for practical applications. To the best of our knowledge, there is no report on the construction of Bi2MoO6 nanosheet arrays for electrochemical applications.
Herein, we report a Bi2MoO6 nanosheet array structure directly grown on Ni foam, which was successfully prepared by the facile hydrothermal reaction illustrated in Scheme 1. Furthermore, this synthesis method is also suitable for growing Bi2MoO6 nanostructures on carbon cloth. The results show that the Ni foam based samples exhibit a superior EC activity with a high specific capacitance of 37.3 F g−1 at a scan rate of 2 A g−1 in 1 M KCl electrolyte. The nanosheet array structures could not only provide a large surface area for a high rate supercapacitor, but also exhibit an excellent electron transport performance. This work provides an effective way to fabricate a Bi2MoO6 nanosheet array on a substrate with research into its electrochemical properties.
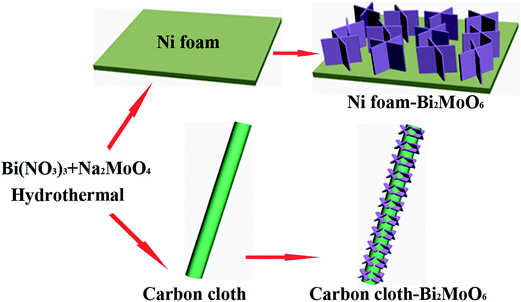 |
| Scheme 1 The schematic illustration of the Bi2MoO6 nanosheet array growth process on Ni foam and carbon cloth. | |
2. Experimental section
2.1 Synthesis of the Bi2MoO6 nanosheet array on Ni foam and carbon cloth
All the reagents were of analytical grade and were used without further purification. The nickel foam was acquired from Shanghai the d new materials co., Ltd with 320–380 g m−2, 1.8 mm thickness and an aperture of 400 μm. The carbon cloth was obtained from physical and chemical (Hong Kong) co., Ltd (W0S1002) with 125 g m−2 and 360 μm thickness. The Ni foam and carbon cloth (4 cm × 1 cm × 1 mm) were cleaned by sonication in acetone, and deionized water in sequence. In a typical synthesis, 0.84 g of Bi(NO3)3·5H2O and 0.2 g of Na2MoO4·7H2O were separately dissolved in 5 mL of ethylene glycol under magnetic stirring. Then, clear solutions formed and these two as-prepared solutions were mixed together in a second step. Finally, 25 mL of ethanol was added into the solution slowly with stirring for 10 min. The resulting clear solution (35 mL) was transferred into a 80 mL Teflon-lined stainless steel autoclave. The prepared Ni foam and carbon cloth were placed into the reaction solution, which was then maintained at 160 °C for 24 h. After cooling down to room temperature, the coated Ni foam and carbon cloth were washed with distilled water and dried at 60 °C. The substrate was cut to form a 2 cm × 1 cm × 1 mm shape for characterizing the electrochemical properties. The substrate was weighed before and after the reaction, and the masses of Bi2MoO6 were 9.8 mg and 10.2 mg grown on the Ni foam and carbon cloth substrates, respectively. For comparison, the electrochemical activities of pristine Ni foam (0.105 g) and carbon cloth (0.05 g) with 4 cm × 1 cm × 1 mm areas were measured in 1 M KCl aqueous electrolyte as well.
2.2 Characterization
SEM images were taken using a field-emission scanning electron microscope (JSM-6701F, JEOL) operated at an accelerating voltage of 5 kV. The X-ray diffraction (XRD) measurements were performed on a Rigaku RINT-2000 instrument using Cu Kα radiation (40 kV). The XRD patterns were recorded from 10° to 80° with a scanning rate of 0.067° s−1. The oxidation states of the product were investigated using an ESCALAB 250Xi X-ray photoelectron spectrometer (XPS) with non-monochromatized Mg Kα X-rays as the excitation source.
2.3 Electrochemical measurements
The electrochemical properties were tested on a CHI660 (Chenhua, Shanghai, China) with a three electrode system. All of the measurements were carried out in 1 M KCl aqueous electrolyte solution at room temperature. The as-obtained Bi2MoO6 nanosheet coated Ni foam and carbon cloth were used as the working electrode separately. A saturated calomel electrode (SCE) and platinum sheet were used as the reference and counter electrodes, respectively. Cyclic voltammetry (CV) curves were measured in a potential range from −0.4 V to −0.1 V at different scan rates, varying from 10 to 50 mV s−1. Galvanostatic charge–discharge (GCD) measurements were recorded at different current densities within the potential range from −0.4 V to −0.1 V. Electrochemical impedance spectroscopy (EIS) measurements were performed in the frequency range from 1 to 105 Hz.
3. Results and discussion
The morphologies and structures of the as-obtained Bi2MoO6 nanosheet arrays were characterized by scanning electron microscopy (SEM), as shown in Fig. 1. It is clear that the samples with a high density were uniformly distributed on the skeleton of the Ni foam (Fig. 1A). These nanosheets interconnect with each other and form an ordered array structure, as shown in Fig. 1B. Moreover, Fig. 1C reveals that the thickness of a single nanosheet was approximately 20 nm. Additionally, Fig. 1D–F display typical SEM images of the Bi2MoO6 nanosheet array grown on carbon cloth. The general view shows the uniform distribution of samples on each carbon fiber. Compared with the nanosheets on Ni foam, overlapping nanosheets with a smaller size are deposited on the carbon cloth. Notably, the nanosheet array structure provides convenient diffusion channels for the penetration of electrolytes, which could dramatically facilitate energy storage.
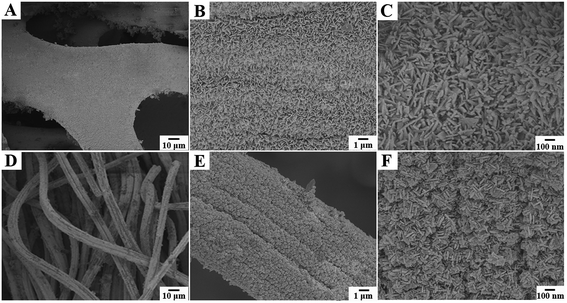 |
| Fig. 1 SEM images of the Bi2MoO6 nanosheet array grown on (A–C) Ni foam and (D–F) carbon cloth. | |
The X-ray diffraction (XRD) patterns of the Bi2MoO6 samples are shown in Fig. 2. Due to interference by the nickel foam, the Ni foam electrode displays weak diffraction peaks ascribed to γ-Bi2MoO6 (JCPDS #21-0102)25 and strong diffraction peaks for nickel (JCPDS #65-2865).24 For the carbon cloth electrode, the diffraction peaks of carbon and Bi2MoO6 are present in the pattern. Moreover, the element composition and oxidation state of the Bi2MoO6 grown on Ni foam were explored by X-ray photoelectron spectroscopy (XPS) (Fig. 2B–D). To reduce the interference caused by the nickel foam, the product was scratched from the nickel foam. As shown in Fig. 2B, the Bi 4f core level spectrum shows two peaks at 164.2 and 158.6 eV, corresponding to Bi 4f5/2 and Bi 4f7/2 of Bi3+. In the Mo 3d pattern (Fig. 2C), the binding energies at 231.9 eV and 235.3 eV can be assigned to Mo 3d5/2 and Mo 3d3/2 of Mo6+, respectively. The Bi–O bonds in Bi2MoO6 result in a peak at 530.9 eV in the O 1s pattern (Fig. 2D). This is consistent with the previous reports on γ-Bi2MoO6.26
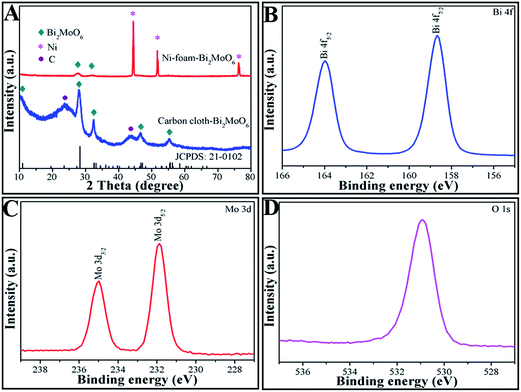 |
| Fig. 2 (A) XRD pattern of the Bi2MoO6 nanosheet arrays and XPS spectra of the Bi2MoO6 nanosheet scratched from the Ni foam substrate (B) Bi 4f core level (C) Mo 3d core level and (D) O 1s core level. | |
To evaluate the electrochemical properties of the Bi2MoO6 nanosheet arrays, their cyclic voltammogram (CV) curves (Fig. 3A and B) and galvanostatic charge–discharge (GCD) patterns (Fig. 3C and D) were investigated in 1 M KCl aqueous electrolyte. It is clear that all the CV curves exhibit an approximately rectangular shape with redox peaks, indicating the strong pseudocapacitive capability of the samples.27,28 Furthermore, the shapes of these curves do not significantly change with scan rate variation, revealing the good capacitive behavior and facilitated charge diffusion. More specifically, the Bi2MoO6 nanosheet array grown on Ni foam has a larger and more regular enclosed area than that of the array on carbon cloth, which is related to a more superior capacitance.29 The specific capacitance values (Cs) of the samples were calculated from the CV curves according to the equation: Cs = ∫IdV/νmΔV, where I (A) is the response current, ν (V s−1) is the scan rate, ΔV (V) is the potential window, and m (g) is the mass of the active electrode material. The maximum specific capacitance of the Ni foam electrode (33.8 F g−1) is higher than that of the carbon cloth electrode (26.6 F g−1) observed over this measurement range.30,31 Compared with carbon cloth, Ni foam is more favorable for the formation of an ordered nanosheet array with a high rate capacitance.
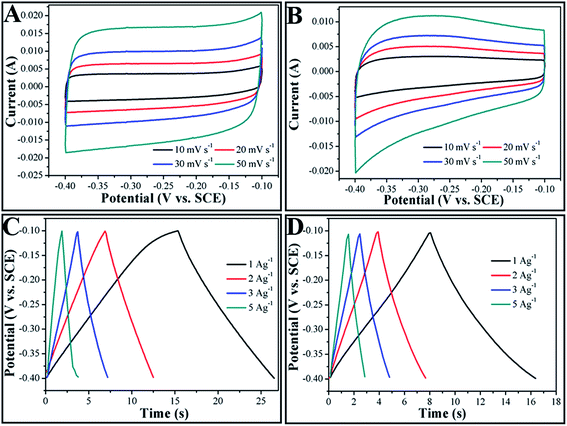 |
| Fig. 3 CV curves of the (A) Ni foam supported Bi2MoO6 nanosheet array and (B) carbon cloth supported Bi2MoO6 nanosheet array. Galvanostatic charge–discharge curves of the (C) Ni foam supported Bi2MoO6 nanosheet array and (D) carbon cloth supported Bi2MoO6 nanosheet array. | |
The GCD results of the samples obtained in a potential window between −0.4 and −0.1 V (vs. SCE) are shown in Fig. 3. The specific capacitance could be calculated based on the discharge data by the following equation: C = It/mΔV, where C (F g−1) is the specific capacitance, I (A) is the discharge current, t (s) is the discharge time, ΔV (V) is the potential window during the discharging process, and m (g) is the mass of the active material.32–34 Calculated from the equitation, the Ni foam supported Bi2MoO6 nanosheet array electrode exhibited a capacitance of 37.3 F g−1 at 2 A g−1, which is much higher than that of the carbon cloth supported electrode (25.1 F g−1) under the same conditions. Therefore, the rational fabrication of a nanosheet array on a good conductive substrate exhibits obvious advantages for pseudocapacitive behavior. The nanosheet array structure directly grown on a substrate not only enhances the connection between the material and substrate, but also provides a larger surface area of the active sites.
In addition, the typical cyclic voltammogram (CV) curves (Fig. 4A and B) and galvanostatic charge–discharge (GCD) patterns (Fig. 4C and D) reveal the capacitance performance of the substrates. Calculated from the CV curves, the specific capacitance values (Cs) of the Ni foam and C cloth are 6.7 × 10−3 and 4.52 × 10−3 F g−1, respectively, at 50 mV s−1, which are much lower than those of the Bi2MoO6 array electrodes (33.8 F g−1 for Bi2MoO6 supported on Ni foam and 26.6 F g−1 for Bi2MoO6 supported on carbon fiber). Moreover, based on the discharge data, the specific capacitance values are 5 × 10−3 and 4 × 10−3 F g−1 for the Ni foam and C cloth at 10−4 A g−1, respectively. It is clear that the substrates make little contribution to the capacitance of the electrodes.
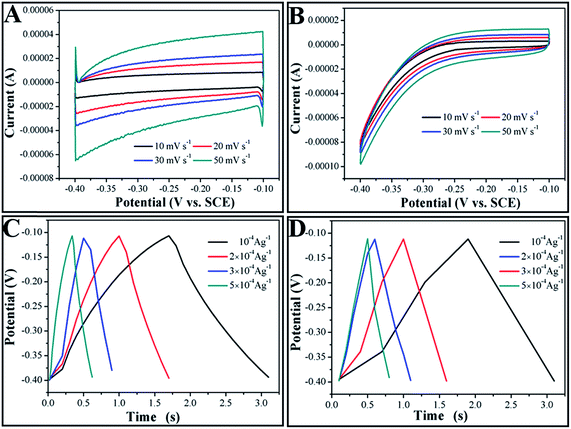 |
| Fig. 4 CV curves of the (A) Ni foam and (B) carbon cloth, and galvanostatic charge–discharge curves of the (C) Ni foam and (D) carbon cloth. | |
The normalized CV curves of the two kinds of Bi2MoO6 nanosheet array grown on different substrates at 20 mV s−1 give a review of the capacitance properties (Fig. 5A). Obviously, the Ni foam supported Bi2MoO6 exhibits a larger enclosed area than the other one, suggesting a larger areal capacitance. Furthermore, the capacitance values of the Ni foam based Bi2MoO6 are 38, 37.3, 34.7 and 33 F g−1 at current densities of 1, 2, 3 and 5 A g−1, respectively. However, the carbon cloth supported Bi2MoO6 possesses capacitance values of 27.7, 25.1, 23.4 and 21.7 F g−1 at current densities of 1, 2, 3 and 5 A g−1, respectively.
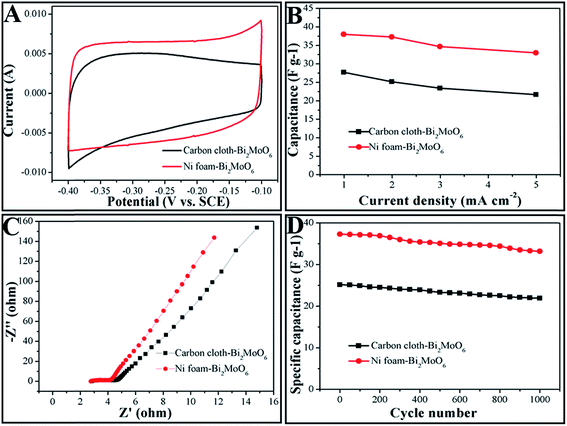 |
| Fig. 5 (A) Normalized CV curves of the Bi2MoO6 nanosheet arrays at 20 mV s−1, (B) capacitance of the samples versus current increase, (C) Nyquist plots of the Bi2MoO6 nanosheet arrays, and (D) cycling performance of the Bi2MoO6 nanosheet arrays at 2 A g−1. | |
In order to reveal the origin of the electrochemical behaviors of the electrodes, the electrochemical impedance spectra (EIS) were characterized and the Nyquist plots are presented in Fig. 5C. As can be seen, the curve includes two parts: the curve in the low frequency area and the semicircle in the high frequency area. The more vertical curve and the smaller semicircle correspond to the lower Warburg impedance (W) and charge-transfer resistance between the electrode and electrolyte, respectively. W represents the diffusive resistance of the electrolyte into the interior of the electrode and ion diffusion into the electrode.35–37 It is clear that the Ni foam supported Bi2MoO6 nanosheet array shows a better electron and ion transport performance than the carbon cloth supported Bi2MoO6 nanosheet array. Moreover, the cycling stability of the electrode is an important factor to investigate for the application of supercapacitors in energy storage. As shown in Fig. 5D, the Ni foam and carbon cloth supported Bi2MoO6 nanosheet arrays exhibit 89% and 87% retentions of the initial capacitance after 1000 cycles at 2 A g−1, respectively, demonstrating the excellent cycling stability at high current densities. The different stabilities of these two electrodes may result from the different densities of the Bi2MoO6 nanosheets on the substrates. With a higher density of Bi2MoO6 nanosheets on the carbon cloth, the electrode is unfavorable for the penetration of electrolytes.38 Above all, it is not hard to conclude that the Ni foam is more suitable as a substrate for the fabrication of the Bi2MoO6 nanosheet array.
4. Conclusion
In summary, Bi2MoO6 nanosheet arrays have been rationally designed on nickel foam and carbon cloth. The Ni foam supported electrode shows excellent electrochemical properties, which are superior to those of the carbon cloth based electrode. A high specific capacitance of 37.3 F g−1 at 2 A g−1 could be achieved with 11% capacitance loss after 1000 cycles. These enhanced electrochemical properties may result from the nanosheet array structure directly grown on the substrate, which provides a large surface area and excellent electric transport. The facile and rational strategy in this report affords an effective way to fabricate two-dimensional array nanostructures for the application of high performance supercapacitors.
References
- X. H. Xia, C. G. Zhu and J. S. Luo, Synthesis of free-standing metal sulfide nanoarrays via anion exchange reaction and their electrochemical energy storage application, Small, 2014, 10, 766–773 CrossRef CAS PubMed.
- C. Zhou, Y. W. Zhang, Y. Y. Li and J. P. Liu, Construction of high-capacitance 3D CoO@polypyrrole nanowire array electrode for aqueous asymmetric supercapacitor, Nano Lett., 2013, 13, 2078–2085 CrossRef CAS PubMed.
- S. Patrice and G. Yury, Materials for electrochemical capacitors, Nat. Mater., 2008, 7, 845–854 CrossRef PubMed.
- A. Adriano, S. Zdenek and P. Martin, Lithium intercalation compound dramatically influences the electrochemical properties of exfoliated MoS2, Small, 2015, 11, 605–612 CrossRef PubMed.
- X. M. Li, Q. G. Li, Y. Wu, M. C. Rui and H. B. Zeng, Two-dimensional, porous nickel–cobalt sulfide for high performance asymmetric supercapacitors, ACS Appl. Mater. Interfaces, 2015, 7, 19316–19323 CAS.
- X. H. Xia, J. P. Tu, Y. Q. Zhang, J. Chen, X. L. Wang, C. D. Gu, C. Guan, J. S. Luo and H. J. Fan, Porous hydroxide nanosheets on performed nanowires by electrodeposition: branched nanoarrays for electrochemical energy storage, Chem. Mater., 2012, 24, 3793–3799 CrossRef CAS.
- V. D. Nithya, R. K. Selvan and L. Vasylechko, Effect of carbon coating on the electrochemical properties of Bi2WO6 nanoparticles by PVP-assisted sonochemical method, J. Appl. Electrochem., 2015, 45, 473–485 CrossRef CAS.
- Y. J. Li, N. Yu and P. Yan, Fabrication of manganese dioxide nanoplates anchoring on biomass-derived cross-linked carbon nanosheets for high-performance asymmetric supercapacitors, J. Power Sources, 2015, 300, 309–317 CrossRef CAS.
- M. F. El-Kady, V. Strong and S. Dubin, Laser scribing of high-performance and flexible graphene – based electrochemical capacitors, Science, 2012, 335, 1326–1330 CrossRef CAS PubMed.
- D. W. Wang, F. Ling and J. P. Zhao, Fabrication of graphene/polyaniline composite paper via in situ anodic electropolymerization for high-performance flexible electrode, ACS Nano, 2009, 3, 1745–1752 CrossRef CAS PubMed.
- J. P. Liu, J. Jiang and C. W. Cheng, Co3O4 nanowire@MnO2 ultrathin nanosheet core/shell arrays: a new class of high-performance pseudocapacitive materials, Adv. Mater., 2011, 23, 2076–2081 CrossRef CAS PubMed.
- C. Z. Yuan, L. Yang, L. R. Hou, L. F. Shen, X. Q. Zhang and X. W. Lou, Growth of ultrathin mesoporous Co3O4 nanosheet arrays on Ni for high-performance electrochemical capacitors, Energy Environ. Sci., 2012, 5, 7883–7887 CAS.
- W. Chen, C. Xia and H. N. Alshareef, One-step electrodeposited nickel cobalt sulfide nanosheet arrays for high-performance asymmetric supercapacitors, ACS Nano, 2014, 8, 9531–9541 CrossRef CAS PubMed.
- Y. G. Li, B. Tan and Y. Y. Wu, Mesoporous Co3O4 nanowire arrays for lithium ion batteries with high capacity and rate capability, Nano Lett., 2008, 8, 265–270 CrossRef CAS PubMed.
- J. J. Xu, K. Wang, S. Z. Zu, B. H. Han and Z. X. Wei, Hierarchical nanocomposites of polyaniline nanowire arrays on graphene oxide sheets with synergistic effect for energy storage, ACS Nano, 2010, 4, 5019–5026 CrossRef CAS PubMed.
- X. H. Xia, G. M. Wang and T. Zhai, High-quality metal oxide core/shell nanowire arrays on conductive substrates for electrochemical energy storage, ACS Nano, 2012, 6, 5531–5538 CrossRef CAS PubMed.
- Q. Q. Hu, W. Q. Ma, G. Liang, H. H. Nan, X. T. Zheng and X. J. Zhang, Anion-exchange reaction synthesized CoNi2S4 nanowires for superior electrochemical performances, RSC Adv., 2015, 5, 84974–84979 RSC.
- J. P. Liu, Y. Y. Li, X. T. Huang, R. M. Ding, Y. Y. Hu, J. Jiang and L. Liao, Direct growth of SnO2 nanorod array electrodesfor
lithium ion batteries, J. Mater. Chem., 2009, 19, 1859–1864 RSC.
- X. H. Lu, D. Z. Zheng, T. Zhai, Z. Q. Liu, Y. Y. Huang, S. L. Xie and Y. X. Tong, Facile synthesis of large-area manganese oxide nanorod arrays as a high-performance electrochemical supercapacitor, Energy Environ. Sci., 2011, 4, 2915–2921 CAS.
- D. Cheng, Y. F. Yang, Y. B. Luo, C. J. Fang and J. Xiong, Growth of ultrathin mesoporous Ni–Mo oxide nanosheet arrays on Ni foam for high-performance supercapacitor electrodes, Electrochim. Acta, 2015, 176, 1343–1351 CrossRef CAS.
- L. Yang, L. Qian, X. Q. Tian, J. Li, J. Y. Dai, Y. Guo and D. Xiao, Hierarchically porous nickel oxide nanosheets grown on nickel foam prepared by one-step in situ anodization for high-performance supercapacitors, Chem.–Asian J., 2014, 9, 1579–1585 CrossRef CAS PubMed.
- M. Kundu and L. F. Liu, Direct growth of mesoporous MnO2 nanosheet arrays on nickel foam current collectors for high-performance pseudocapacitors, J. Power Sources, 2013, 243, 676–681 CrossRef CAS.
- J. L. Long, S. C. Wang, H. J. Chang, B. Z. Zhao, B. T. Liu, Y. G. Zhou, W. Wei, X. X. Wang, L. Huang and W. Huang, Bi2MoO6 Nanobelts for Crystal Facet-Enhanced Photocatalysis, Small, 2014, 10, 2791–2795 CrossRef CAS PubMed.
- B. Senthilkumar, R. K. Selvan, L. Vasylechko and M. Minakshi, Synthesis, crystal structure and pseudocapacitor electrode properties of gamma-Bi2MoO6 nanoplates, Solid State Sci., 2014, 35, 18–27 CrossRef CAS.
- G. H. Tian, Y. J. Chen, W. Zhou, K. Pan, Y. Z. Dong, C. G. Tian and H. G. Fu, Facile solvothermal synthesis of hierarchical flower-like Bi2MoO6 hollow spheres as high performance visible-light driven photocatalysts, J. Mater. Chem., 2011, 21, 887–892 RSC.
- M. Y. Zhang, C. L. Shao, J. B. Mu, Z. Y. Zhang, Z. C. Guo, P. Zhang and Y. C. Liu, Hierarchical heterostructures of Bi2MoO6 on carbon nanofibers: controllable solvothermal fabrication and enhanced visible photocatalytic properties, CrystEngComm, 2012, 14, 605–612 RSC.
- E. Frackowiak and F. Beguin, Carbon materials for the electrochemical storage of energy in capacitors, Carbon, 2001, 39, 937–950 CrossRef CAS.
- P. H. Yang, Y. Ding, Z. Y. Lin, Z. W. Chen, Y. Z. Li, P. F. Qiang, M. Ebrahimi, W. J. Mai, C. P. Wong and Z. L. Wang, Low-cost high-performance solid-state asymmetric supercapacitors based on MnO2 nanowires and Fe2O3 nanotubes, Nano Lett., 2014, 14, 731–736 CrossRef CAS PubMed.
- L. N. Wang, Y. Ma, M. Yang and Y. X. Qi, Hierarchical hollow MoS2 nanospheres with enhanced electrochemical properties used as an electrode in supercapacitor, Electrochim. Acta, 2015, 186, 391–396 CrossRef CAS.
- X. Zhang, X. Z. Zeng, M. Yang and Y. X. Qi, Investigation of a branchlike MoO3/polypyrrole hybrid with enhanced electrochemical performance used as an electrode in supercapacitors, ACS Appl. Mater. Interfaces, 2014, 6, 1125–1130 CAS.
- H. Wang, C. Wang, C. Qing, D. M. Sun, B. X. Wang, G. Qu, M. Sun and Y. W. Tang, Construction of carbon–nickel cobalt sulphide hetero-structured arrays on nickel foam for high performance asymmetric supercapacitors, Electrochim. Acta, 2015, 174, 1104–1112 CrossRef CAS.
- C. H. Wang, X. Zhang, C. Li, X. Z. Sun and Y. W. Ma, Facile fabrication of nanostructured NiCo2O4 supported on Ni foam for high performance electrochemical energy storage, RSC Adv., 2015, 5, 80620–80624 RSC.
- Y. Huang, J. Y. Tao, W. J. Meng, M. S. Zhu, Y. Huang, Y. Q. Fu, Y. H. Gao and C. Y. Zhi, Super-high rate stretchable polypyrrole-based supercapacitors with excellent cycling stability, Nano Energy, 2015, 11, 518–525 CrossRef CAS.
- V. H. Nguyen and J. J. Shim, Three-dimensional nickel foam/graphene/NiCo2O4 as high-performance electrodes for supercapacitors, J. Power Sources, 2015, 273, 110–117 CrossRef CAS.
- M. D. Stoller, S. J. Park, Y. W. Zhu, J. H. An and R. S. Ruoff, Graphene-based ultracapacitors, Nano Lett., 2008, 8, 3498–3502 CrossRef CAS PubMed.
- S. D. Min, C. J. Zhao, G. R. Chen and X. Z. Qian, One-pot hydrothermal synthesis of reduced graphene oxide/Ni(OH)(2) films on nickel foam for high performance supercapacitors, Electrochim. Acta, 2014, 115, 155–164 CrossRef CAS.
- C. Kim, B. T. N. Ngoc, K. S. Yang, M. Kojima, Y. A. Kim, Y. J. Kim, M. Endo and S. C. Yang, Self-sustained thin webs consisting of porous carbon nanofibers for supercapacitors via the electrospinning of polyacrylonitrile solutions containing zinc chloride, Adv. Mater., 2007, 19, 2341–2346 CrossRef CAS.
- F. X. Bao, Z. Q. Zhang, W. Guo and X. Liu, Facile synthesis of three dimensional NiCo2O4@MnO2 core–shell nanosheet arrays and its supercapacitive performance, Electrochim. Acta, 2015, 157, 31–40 CrossRef CAS.
|
This journal is © The Royal Society of Chemistry 2016 |