DOI:
10.1039/C5RA26085J
(Paper)
RSC Adv., 2016,
6, 16645-16655
Human neutrophils ROS inhibition and protective effects of Myrtus communis leaves essential oils against intestinal ischemia/reperfusion injury
Received
7th December 2015
, Accepted 22nd January 2016
First published on 27th January 2016
Abstract
The aim of the present work was to investigate the mechanism implicated in the protective effects of Myrtus communis leaves essential oils (MCEO) on human neutrophils reactive oxygen species (ROS) production. We also studied its preventive effect against intestinal ischemia reperfusion (IIR)-induced oxidative stress in rat model. Essential oils were obtained from the plant leaves by hydrodistillation and analyzed by GC-MS. Neutrophils were isolated from whole human blood using ficoll–dextran method. ROS generation and H2O2 production were measured by luminol-amplified chemiluminescence. The cytochrome c reduction assay was used for superoxide anion determination and Western blotting analysis was to determine the neutrophils myeloperoxidase (MPO) expression. Rats were divided into four groups: control (C), intestinal IR (IIR), MCEO, and MCEO plus IIR. Animals were pretreated with MCEO (50 mg kg−1) during 7 days. IIR was produced by 75 min of intestinal ischemia followed by reperfusion for 120 min. The GC-MS analysis, allowed to the identification of twenty five bioactive compounds in MCEO. In vitro, we found that MCEO inhibited ROS and H2O2 production and attenuated the neutrophils MPO expression. In vivo, the MCEO administration counteracted IIR-induced small intestine, lung and liver lipid peroxidation as well as the depletion of antioxidant enzymes activities such as superoxide dismutase (SOD), catalase (CAT) and glutathione peroxidase (GPx). MCEO pretreatment also corrected IIR-induced non-enzymatic antioxidants levels depletion such as sulfhydryl groups (–SH) and reduced glutathione (GSH). More importantly, IIR was accompanied by H2O2, free iron and calcium increase while MCEO administration reversed all intracellular mediator perturbations. In conclusion, we suggest that MCEO had a potential protective role against intestinal IR injury, in part owing to its antioxidant potential and ROS scavenging activities.
1. Introduction
Ischemia can be defined as a cessation or an insufficient blood supply to a tissue or organ. This deficiency is not only about the oxygen supply, but also the substrates and products of metabolism. Anaerobic glycolysis then starts up, but its energy intake is inadequate.1 Intestinal ischemia/reperfusion (IIR) is a common situation for many diseases such as acute mesenteric ischemia, septic or traumatic shock, severe burns, or some surgical procedures including small bowel transplantation, abdominal aortic aneurysm, hemorrhagic, traumatic or septic shock, and even severe burns.2,3 During intestinal ischemia/reperfusion there is a cascade of events whose chain inexorably leads to cell death.4 The first consequence is the energy depletion by stopping the production of energy-rich compounds, along with the collapse of the intracellular level of ATP, degradation products: adenosine diphosphate (ADP), adenosine monophosphate (AMP), hypoxanthine, xanthine and uric acid, are released into the cells.5 Reoxygenation restores the energy charge of the cells, but causes the generation of toxic metabolites: reactive oxygen species (ROS) or free radicals, key metabolites of the pathogenesis of mesenteric reperfusion.3,6,7 Activated neutrophils and endothelial cells will play a key role in the pathogenesis of mesenteric reperfusion. They induce lesions in both cellular and tissue.8
Myrtus communis is a perennial shrub, evergreen, from 1.8 to 2.4 m high, with little foliage and deep fissured bark. It is native to southern Europe, North Africa and West Asia. It is distributed in South America, North-Western Himalayas and Australia and widespread in the mediterranean region.9 Terpenes and terpene alcohols are the major constituents of the total volatile compounds of the essential oils.10 The myrtle leaves are used as an antiseptic and anti-inflammatory agent, as well as a mouthwash for the treatment of candidiasis.11 Generally, the decoction of leaves is used orally for the treatment of stomach pain, hypoglycemia, disbiosis, constipation, and used for wound healing.12,13
The aim of the present study was to investigate the protective effect of Myrtus communis essential oils on neutrophils ROS production and intestinal ischemia/reperfusion-induced oxidative stress in rat. We also studied the involvement of intracellular mediators in such protection.
2. Materials and methods
2.1. Reagents and antibodies
PMA, fMLF and protease inhibitors were from Sigma-Aldrich (St Quentin Fallavier, France). The anti-MPO antibody was from Abcam. 5,5-Dithio bis(2-nitrobenzoic acid) (DTNB), trichloroacetic acid (TCA), acetylcholine iodide, S-butyrylcholine, butylhydroxytoluene (BHT), methanol, ether, bovine serum albumin (BSA), orthophosphoric acid and NaCl were purchased from Sigma-Aldrich Co. (Germany).
2.2. Plant collection
Myrtle (Myrtus communis L.) leaves were collected in March, 2015 from the locality of Ain-Draham (Northwestern of Tunisia) and identified by the laboratory of taxonomy in the Faculty of Sciences of Tunis (FST), Tunisia. The voucher specimens (No. MY01) have been deposited in the herbarium of the Higher Institute of Biotechnology of Beja and in the Department of Biological Sciences, Faculty of Science of Bizerte, Tunisia.
2.3. Essential oils preparation
The leaves of Myrtus communis were submitted to hydrodistillation for 3 hours using Clevenger type apparatus. Briefly, the plant was immersed in water and heated to boiling, after which the essential oils were evaporated together with water vapour and finally collected in a condenser. The distillate was isolated and dried over anhydrous sodium sulfate. The oils fractions were stored at 4 °C until use.14
2.4. Gas chromatography-mass spectrometry (GC-MS) analysis
The essential oils of myrtle leaves were subjected to GC-MS analysis using Trace GC ULTRA/Polaris Q (GC-MS, Thermo Electron). The column was a VB-5 (5% phenyl/95% dimethylpolysiloxane) with film thickness of 0.25 μm, a length of 30 m and an internal diameter of 0.25 μm helium was used as carrier gas. The GC oven temperature was kept at 50 °C for 5 min and programmed to 250 °C for 3 min at rate of 4 °C min−1 and programmed to 300 °C, at rate of 25 °C min−1. The injector temperature was set at 250 °C. Split flow was adjusted at 50 mL min−1. MS were taken at 70 eV. Mass range was from uma 20 to 350. A library search was carried out using the “Wiley GC/MS Library”, Nist and Pmw. 1 μL of the sample (dissolved in hexane as 1/50 v/v) was injected in to the system.14
2.5. Ethics
Neutrophils were isolated from venous blood of healthy volunteers managed in the hematology and immunology department of Bichat Hospital, Paris, France. All experiments were approved by the “Institut National de la Santé et de Recherche Médicale (INSERM)” institutional review board and ethics committee. Data collection and analyses were performed anonymously.
2.6. Human neutrophil preparation
Neutrophils were isolated from blood of healthy volunteers by dextran sedimentation and Ficoll centrifugation using phosphate-free buffer as described previously by El-Benna and Dang (2007).15 Erythrocytes were removed by hypotonic lysis. Following isolation, the cells were resuspended in Hank's balanced salt solution (HBSS).
2.7. Measurement of neutrophil ROS production by chemiluminescence
Luminol-amplified chemiluminescence was measured as previously described by Jabri et al. (2015).16 Briefly, neutrophil suspensions (5 × 105 cells) in 0.5 mL HBSS containing 10 μM luminol were preheated in the presence or absence of the MCEO to 37 °C in the luminometer thermostated chamber (Berthold-Biolumat LB937) and allowed to stabilize. After a baseline reading was established, cells were stimulated with 100 ng mL−1 PMA or 0.1 mM fMLF, and changes in chemiluminescence were monitored.
2.8. Superoxide anion production measurement
Isolated neutrophils were resuspended in HBSS at a concentration of 1 million per mL. The reaction mixture containing 1 mg mL−1 of cytochrome c in the presence or absence of the MCEO were preheated to 37 °C in the thermostatted chamber of a spectrophotometer (Uvikon) and allowed to stabilize. Afterwards, cells were stimulated with 0.1 mM fMLF or 100 ng mL−1 PMA. Changes in absorbance were measured at 550 nm over a 15 min period.
2.9. Measurement of H2O2 inhibition by chemiluminescence
The H2O2 inhibition was tested in a cell free system as described by Jabri et al. (2015).16 Briefly, the reaction mixture contained 10 mM luminol in the presence or absence of the MCEO. The reaction was started by the addition of 2.5 U mL−1 horseradish peroxidase (HRPO), and the lucigenin chemiluminescence was measured at 37 °C for 30 min in a luminometer (Berthold-Biolumat LB937).
2.10. Measurement of released MPO from neutrophils
Neutrophils were lysed by nitrogen cavitation and the granule fraction was purified by Percoll gradient centrifugation.17 MPO content was measured also by Western blotting analysis.16
2.11. Animals and treatment
Forty adult male Wistar rats (weighing 220–240 g, housed five per cage) were purchased from Pasteur Institute of Tunis and used in accordance with the local ethics committee of Tunis University for the use and care of animals and in accordance with the NIH recommendations.18 They were provided with standard food (BADER, Utique, TN) and water ad libitum, and maintained in an animal house under a controlled temperature (22 ± 2 °C) with a 12–12 h light–dark cycle. Rats were divided into four groups of 10 animals each. The animals were intraperitoneally (i.p.) injected for 7 days with bidistilled water (group I and III) or with 50 mg kg−1 body weight (bw) of MCEO (group II and IV). Twenty-four hours after the last injection, intestinal ischemia/reperfusion (IIR) was performed on group III (IIR) and IV (IIR + MCEO). Group I (control) and group II (MCEO).
2.12. Intestinal I/R experimental model
The rat mode of intestinal I/R (IIR) was performed as described by Gan et al. (2012).19 Before surgical procedures rats were fasted for 18 h with free access to water. The rats were anesthetized by an intraperitoneal injection of 10% chloral hydrate (3.5 mL kg−1). The abdominal region shaved and cleaned with antiseptic solution. Using sterile technique, the abdomen was opened with a middle incision. Intestines were exteriorized and the superior mesenteric artery (SMA) was dissected. After identifying the SMA, the small intestine was subjected to the ischemia by occluding the SMA with a nontraumatic microvascular clamp. The clamp was removed 75 min later and reperfusion occurred for 2 h. Reperfusion was confirmed by the restoration of pulsation and color prior to closing incision. Animals in the group I and II were submitted to the abdominal incision but not the IIR. At the end of reperfusion period, rats were sacrificed and the small intestine, liver and lungs were excised and gently rinsed with 0.9% NaCl.
2.13. Tissues preparation
The small intestine, liver and lungs were rapidly excised and homogenized in phosphate buffer saline (KH2PO4/K2HPO4, 50 mM, pH 7.4) with Potter–Elvehjem homogenizer. After centrifugation at 10
000g for 10 min at 4 °C, supernatant was used for the biochemical determination of protein, free iron, H2O2, calcium, –SH groups, GSH, MDA and antioxidant enzyme activities.
2.14. Biochemical estimations
2.14.1. Lipid peroxidation determination. The lipid peroxidation was determined by MDA measurement according to the double heating method.20 Briefly, aliquots from small intestine, liver and lung tissues homogenates were mixed with BHT–trichloroacetic acid (TCA) solution containing 1% BHT (w/v) dissolved in 20% TCA (w/v) and centrifuged at 1000 × g for 5 min at 4 °C. Supernatant was blended with solution containing (0.5 N HCl, 120 mM TBA buffered in 26 Mm Tris) and then heated at 80 °C for 10 min. After cooling, the absorbance of the resulting chromophore was determined at 532 nm. MDA levels were determined by using an extinction coefficient for MDA-TBA complex of 1.56 × 105 M−1 cm−1.
2.14.2. Non-enzymatic antioxidants measurement. The total concentration of thiol groups (–SH) was performed according to Ellman's method.21 Briefly, homogenates of tissues were mixed with 800 μL of 0.25 M phosphate buffer (pH 8.2) and 100 μL of 20 mM EDTA, and the optical density was measured at 412 nm (A1). Then, 100 μL of 10 mM DTNB were added, incubated during 15 min and the absorbance of the sample was quantified at 412 nm (A2). The thiol groups concentration was calculated from A1 to A0 subtraction using a molar extinction coefficient of 13.6 × 103 M−1 × cm−1. The results were expressed as μmo of thiol groups per mg of protein.GSH was estimated in small intestine, liver and lung tissues by the method of Sedlak and Lindsay.22 Briefly, 500 μL of tissue homogenate prepared in 20 mM EDTA, (pH 4.7) were mixed with 400 μL of cold distilled water and 100 μL of 50% TCA. The samples were shaken using vortex mixer and centrifuged at 1200 × g during 15 min. Following centrifugation, 2 mL of supernatant were mixed with 400 μL of 400 mM Tris–buffer (pH 8.9) and 10 μL of 10 mM DTNB. The absorbance was read at 412 nm against blank tube without homogenate.
2.14.3. Antioxidant enzymes activities assays. The activity of SOD was determined using modified epinephrine assays.23 At alkaline pH, superoxide anion O2˙− causes the autoxidation of epinephrine to adenochrome; while competing with this reaction, SOD decreased the adenochrome formation. One unit of SOD is defined as the amount of the extract that inhibits the rate of adenochrome formation by 50%. Enzyme extract was added to 2 mL reaction mixture containing 10 μL of bovine catalase (0.4 U μL−1), 20 μL of epinephrine (5 mg mL−1) and 62.5 mM of sodium carbonate/bicarbonate buffer pH 10.2. Changes in absorbance were recorded at 480 nm.CAT activity was assayed by measuring the initial rate of H2O2 disappearance at 240 nm.24 The reaction mixture contained 33 mM H2O2 in 50 mM phosphate buffer pH 7.0 and CAT activity was calculated using the extinction coefficient of 40 mM−1 cm−1 for H2O2.
The activity of GPx was quantified following the procedure of Flohé and Günzler.25 Briefly, 1 mL of reaction mixture containing 0.2 mL of small intestine, liver or lung supernatants, 0.2 mL of phosphate buffer 0.1 M pH 7.4, 0.2 mL of GSH (4 mM) and 0.4 mL of H2O2 (5 mM) was incubated at 37 °C for 1 min and the reaction was stopped by the addition of 0.5 mL TCA (5%, w/v). After centrifugation at 1500g for 5 min, aliquot (0.2 mL) from supernatant was combined with 0.5 mL of phosphate buffer 0.1 M pH 7.4 and 0.5 mL DTNB (10 mM) and absorbance was read at 412 nm. The activity of GPx was expressed as nmol of GSH consumed per min per mg protein.
2.14.4. H2O2 determination. The tissues H2O2 level was performed according to Dingeon et al.26 Briefly, in the presence of peroxidase, the hydrogen peroxide reacts with p-hydroxybenzoic acid and 4-aminoantipyrine leading to a quantitative formation of a quinoneimine which has a pink color detected at 505 nm.
2.14.5. Iron measurement. Tissues non haem iron were measured colorimetrically using ferrozine as described by Leardi et al.27 Briefly, the iron dissociated from transferrin–iron complex by a solution of guanidine acetate and reduced by ascorbic acid reacts with ferrozine to give a pink complex measured at 562 nm.
2.14.6. Calcium determination. Small intestine, liver and lung calcium levels was performed using colorimetric method according to Stern and Lewis.28 Briefly, at alkaline medium, calcium reacts with cresolphthalein leading to a coloured complex measurable at 570 nm.
2.14.7. Protein determination. Protein concentration was determined according to Hartree29 which is a slight modification of the Lowry method. Serum albumin was used as standard.
2.15. Statistical analysis
Data were analyzed by unpaired Student's t-test or one-way analysis of variance (ANOVA) and are expressed as means ± standard error of the mean (SEM). Data are representative of four independent experiments. All statistical tests were two-tailed, and a p value of 0.05 or less was considered significant.
3. Results
3.1. Essential-oils composition
GC/MS analyses allowed to the identification of 25 constituents, representing 98.9% of the total essential oils composition (Fig. 1). The main constituents presented in Table 1 are α-pinene (55.66%), o-cymene (1.10%), 1,8-cineole (30.05%), 4-carene (1.10%), linalool (3.15%), terpineol (1.55%) and geranyl acetate (1.07%).
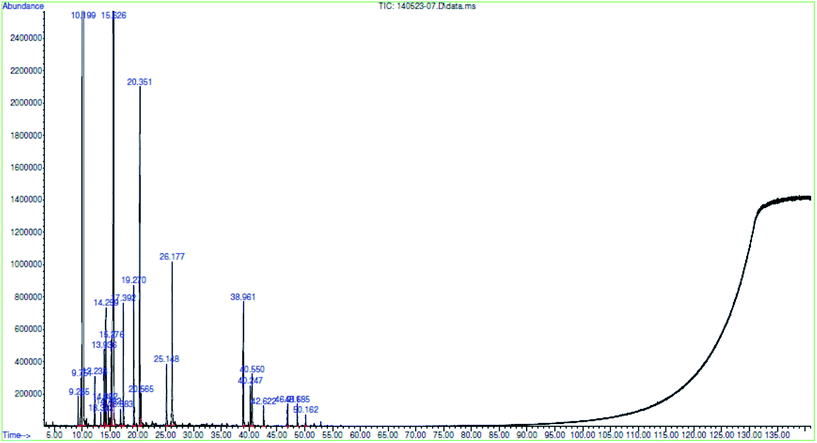 |
| Fig. 1 Representative GC/MS analysis Myrtus communis leaves essential oils (assignments of peaks are given in Table 1). | |
Table 1 GC/MS profile of Myrtus communis leaves essential oils
No. |
Phyto-components |
% peak area |
Retention time (min) |
1 |
Isobutyric acid |
0.18 |
9.25 |
2 |
Thujene |
0.51 |
9.74 |
3 |
α-Pinene |
55.66 |
10.20 |
4 |
β-Pinene |
0.35 |
12.23 |
5 |
β-Myrcene |
0.10 |
13.34 |
6 |
α-Phellandrene |
0.54 |
13.93 |
7 |
Butanoic acid |
0.17 |
14.09 |
8 |
3-Carene |
0.83 |
14.25 |
9 |
Terpinene |
0.16 |
14.70 |
10 |
o-Cymene |
1.10 |
15.27 |
11 |
1,8-Cineole |
30.05 |
15.62 |
12 |
E-β-Ocimene |
0.14 |
16.88 |
13 |
γ-Terpinene |
0.90 |
17.39 |
14 |
4-Carene |
1.10 |
19.26 |
15 |
Linalool |
3.15 |
20.34 |
16 |
Butyric acid |
0.28 |
20.56 |
17 |
L-Terpinen-4-ol |
0.59 |
25.14 |
18 |
Terpineol |
1.55 |
26.17 |
19 |
Geranyl acetate |
1.07 |
38.96 |
20 |
Methyleugenol |
0.39 |
40.24 |
21 |
Caryophyllene |
0.48 |
40.54 |
22 |
α-Caryophyllene |
0.18 |
42.62 |
23 |
Methylxanthine |
0.22 |
46.91 |
24 |
Germacrene B |
0.20 |
48.68 |
25 |
Caryophyllene oxide |
0.10 |
50.16 |
3.2. Effects of MCEO on reactive oxygen species production by neutrophils
As illustrated in Fig. 2A and B, PMA and fMLF induced a potent ROS production detected by chemiluminescence, while MCEO significantly decreased in a dose-dependent manner.
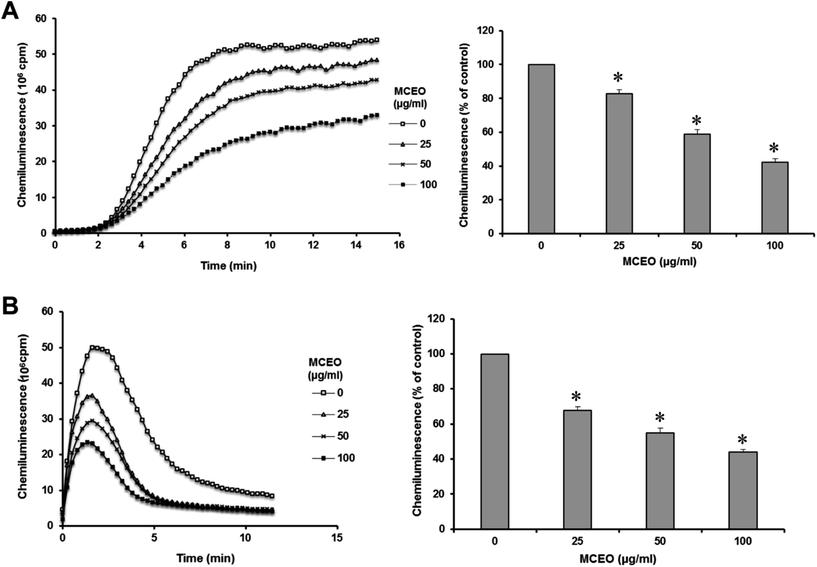 |
| Fig. 2 Effect of MCEO on 100 ng mL−1 PMA (A) and 0.1 mM fMLF (B) stimulated ROS production by human neutrophils (5 × 105 cells/0.5 mL HBSS). Neutrophils were incubated in Hanks buffer containing MCEO (50 mg mL−1) for 30 minutes; ROS production was measured with a chemiluminescence technique in the presence of 10 μM luminol. Total chemiluminescence in each condition was quantified and presented as the mean ± SEM of 5 experiments (*p < 0.05). The amounts of ROS production represented by the histograms are calculated by integration of the area under the curve obtained by chemiluminescence. | |
3.3. Effect of MCEO on H2O2 production in a cell free system
The effect of myrtle on H2O2 production has been studied and the results are presented in Fig. 3. We showed that all used dilutions of MCEO significantly inhibited luminol-amplified chemiluminescence stimulated with horseradish peroxidase (HRPO), indicating that MCEO are potent H2O2 scavenging activity.
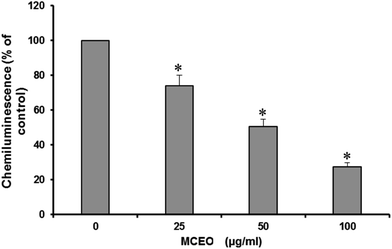 |
| Fig. 3 Effect of MCEO on luminol-amplified chemiluminescence of H2O2. H2O2 inhibition was measured during 30 min with a chemiluminescence technique in the presence of 10 μM luminol. The reaction mixture is stimulated by the addition of 2.5 U mL−1 horseradish peroxidase (HRPO), total chemiluminescence in each condition was quantified and presented as the mean ± SEM of 5 experiments (*p < 0.05). | |
3.4. Effects of MCEO on neutrophils superoxide anion production
As expected, PMA and fMLF have stimulated the superoxide anion production by neutrophils in control experiments performed without MCEO. However, pretreatment of neutrophils with different concentrations of MCEO had no effect in superoxide anion production (Fig. 4).
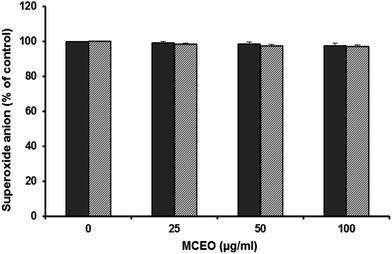 |
| Fig. 4 Effect of MCEO on PMA and fMLF stimulated superoxide anion production by human neutrophils. Neutrophils were incubated in the presence or not of MCEO and stimulated with PMA (100 ng mL−1) or fMLF (0.1 mM). Cytochrome c reduction was measured at 550 nm in a spectrophotometer for 10 min (data are mean plus or minus SEM of 5 experiments, *p < 0.05). | |
3.5. Effect of MCEO on PMA-induced MPO release from neutrophils
We further studied the effect of MCEO on PMA-induced MPO release. As shown in Fig. 5, PMA administration caused a potent increase of the MPO expression in neutrophils. However, MCEO treatment, significantly and dose-dependently protected against this perturbation of MPO expression.
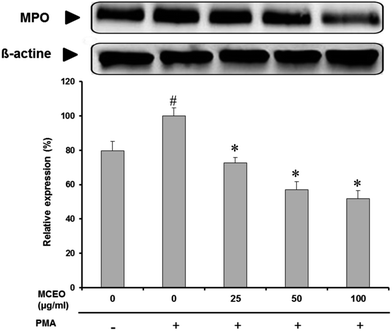 |
| Fig. 5 MPO expression in human neutrophils (2 × 106 cells per mL HBSS) treated or not with MCEO and stimulated with 100 ng mL−1 PMA. Cells were centrifuged and MPO protein expression was determined by immunoblotting with anti MPO antibody. Western blots from different experiments were scanned; MPO were quantified by densitometry (data are mean plus or minus SEM of 5 experiments, #: p < 0.05 compared to control group and *: p < 0.05 compared to PMA group). | |
3.6. Effects of MCEO on MDA levels in the small intestine, lung and liver tissues
As expected, MDA levels in the small intestine, lung and liver tissues of IIR group was significantly increased when compared to control group. Importantly, pre-treatment with MCEO, significantly reversed all IIR-induced tissue lipoperoxidation (Fig. 6).
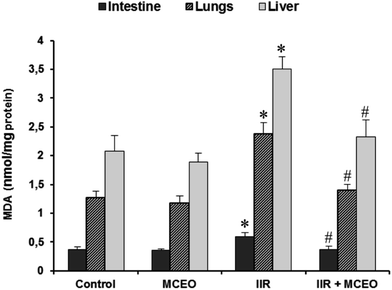 |
| Fig. 6 Effects of intestinal IR and Myrtus communis essential oils (MCEO) supplementation on tissues MDA levels. Animals were pretreated with MCEO (50 mg kg−1; i.p.) or vehicle (H2O) during 7 days before IIR induction. The data are expressed as mean ± SEM (n = 10). *: p < 0.05 compared to control group and #: p < 0.05 compared to IIR group. | |
3.7. Effects of MCEO on non-enzymatic antioxidant levels in the small intestine, lung and liver tissues
We also studied the effects of MCEO and IIR on non-enzymatic antioxidant levels (Fig. 7). IIR per se, induced a significant decrease of GSH and –SH groups levels in small intestine, lung and liver when compared to the control group. However, the essential oils administration attenuated this decrease of non-enzymatic antioxidant levels induced by IIR.
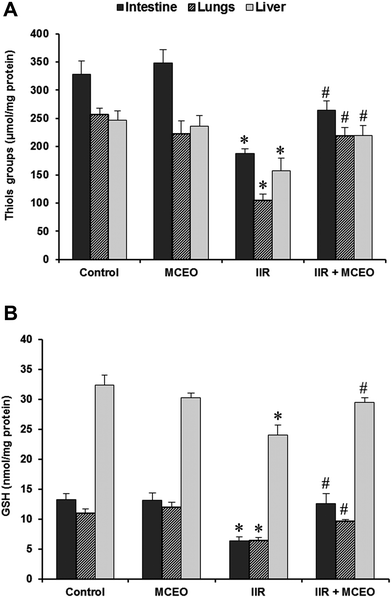 |
| Fig. 7 Effects of intestinal IR and Myrtus communis essential oils (MCEO) supplementation on tissues non-enzymatic antioxidants levels. Animals were pretreated with MCEO (50 mg kg−1; i.p.) or vehicle (H2O) during 7 days before IIR induction. The data are expressed as mean ± SEM (n = 10). *: p < 0.05 compared to control group and #: p < 0.05 compared to IIR group. | |
3.8. Effects of MCEO on antioxidant enzyme activities
On other hand we examined the effect of MCEO treatment and IIR on antioxidant enzymes activities. IIR significantly decreased small intestine, lung and liver tissues enzymes activities such as SOD (Fig. 8A), CAT (Fig. 8B), and GPx (Fig. 8C). Importantly, MCEO pretreatment markedly restored the antioxydant enzymes activities to near control levels.
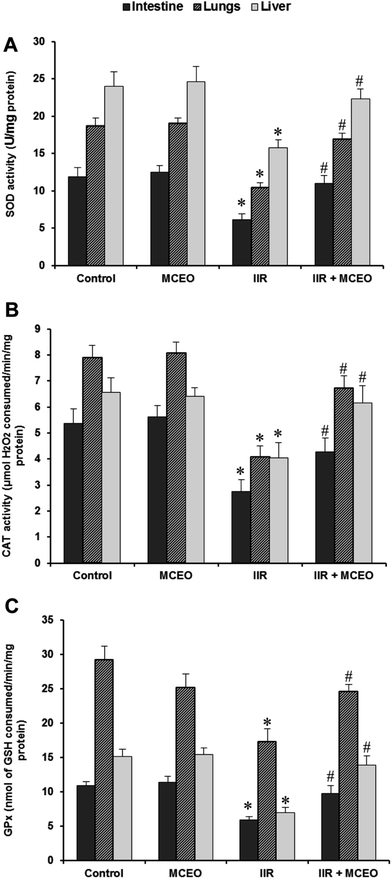 |
| Fig. 8 Effects of intestinal IR and Myrtus communis essential oils (MCEO) supplementation on tissues antioxidant enzymes activities animals were pretreated with MCEO (50 mg kg−1; i.p.) or vehicle (H2O) during 7 days before IIR induction. The data are expressed as mean ± SEM (n = 10). *: p < 0.05 compared to control group and #: p < 0.05 compared to IIR group. | |
3.9. Effect of MCEO on H2O2, free iron and calcium levels
We further sought to evaluate the degree of implication of intracellular mediators are in IIR injury as well as the putative protection offered by MCEO (Table 2). We showed that IIR clearly elevated H2O2, free iron and calcium levels compared to the control group. Whereas MCEO administration significantly reduced the IIR-induced intracellular mediators deregulation.
Table 2 Effects of intestinal IR and Myrtus communis essential oils (MCEO) supplementation on tissues H2O2, free iron and calcium levels (mean ± SD). *: p < 0.05 compared to control group and #: p < 0.05 compared to IIR group
Groups |
H2O2 (mmol per mg per protein) |
Free iron (μmol per mg per protein) |
Calcium (μmol per mg per protein) |
Intestine |
Lungs |
liver |
Intestine |
Lungs |
liver |
Intestine |
Lungs |
liver |
Control |
8.79 ± 0.57 |
15.87 ± 0.91 |
9.51 ± 0.91 |
81.12 ± 4.20 |
130.69 ± 8.54 |
217.8 ± 17.25 |
50.92 ± 4.00 |
33.46 ± 2.64 |
39.68 ± 5.51 |
MCEO |
8.07 ± 0.63 |
16.64 ± 0.90 |
9.32 ± 0.73 |
90.72 ± 8.49 |
142.76 ± 5.34 |
213.44 ± 13.90 |
43.75 ± 5.23 |
37.88 ± 2.64 |
36.73 ± 3.75 |
IIR |
18.14 ± 0.91* |
31.65 ± 1.52* |
16.69 ± 1.13* |
163.65 ± 9.61* |
234.56 ± 17.42* |
328.91 ± 20.43* |
93.83 ± 4.45* |
77.26 ± 3.14* |
79.11 ± 5.50* |
IIR + MCEO |
10.31 ± 0.78# |
18.60 ± 0.98# |
10.48 ± 0.64# |
110.31 ± 7.53# |
151.98 ± 15.14# |
247.83 ± 18.21# |
54.17 ± 6.83# |
44.42 ± 5.42# |
46.64 ± 3.38# |
4. Discussion
The present study outlines the involvement of the redox status as well as the role of intracellular mediators in the mode of action of Myrtus communis essential oils against acute intestinal ischemia/reperfusion-induced small intestine, lung and liver injury.
We firstly examined, the chemical composition of the essential oils obtained from myrtle leaves. The use of combined GC-MS analysis allowed us to identify 25 phyto-compounds, which most belong to monoterpenes hydrocarbons and oxygenated monoterpenes. Our results generally corroborate previous reports with some discrepancies. However, it was previously shown that that 1,8-cineole and α-pinene were the main constituents of different parts of Tunisian MCEO.30,31 Myrtenyl acetate was the major component of essential oils obtained from different myrtle parts from Portuguese32 and Croatia.33 In contrast, other compounds such as sabinene, z-carveol and nerol were not detected in our sample, but were found in previous report.31 The variability in chemical composition can be attributed in part to the climatic conditions as well as the mode of extraction.34 Indeed, this variability may also be due to the variety of myrtle as recently described by Messaoud and Boussaid35 for Tunisian myrtle essential oils. However myrtle leaf essential oils is very well known to be used as a powerful antioxidant.31,35
We also tested in the present investigation, the effect of MCEO on ROS production by human neutrophils. Reactive oxygen species production was assessed by using luminol-amplified chemiluminescence. We showed that neutrophils incubation with MCEO (25, 50 and 100 μg mL−1) during 15 min induced a strong inhibition of ROS production and H2O2 accumulation in the cell free system stimulated with horseradish peroxidase (HRPO). At the same concentrations, MCEO did not affect the neutrophils' viability, determined through trypan blue exclusion test (data not shown). Moreover, the myrtle essential oils also had no effect on fMLF or PMA-induced superoxide anion production, reflect of NADPH oxidase activity as previously described for myrtle berry seed aqueous extract.16 However myrtle leaf essential oils have been previously shown for their antioxidant properties.31,35 On the other hand, MCEO significantly and dose-dependently abrogated PMA-induced an increase in neutrophils MPO expression. Indeed, myeloperoxidase from activated neutrophils interact with the hydrogen peroxide and catalysis the hypochlorous acid ion formation which reacts with membrane primary amines to produce chlorine derivatives having a strong oxidizing power.36,37 In addition, reactive oxygen species are produced by neutrophils during the reduction of oxygen molecule into water. The transfer of an electron to oxygen generates the radical superoxide (O2˙−),38 transformed by superoxide dismutase into hydrogen peroxide.39 By adding new electrons, H2O2 is converted to the hydroxyl radical (OH˙). It allows oxidation of biological substances such as proteins, nucleic acids and unsaturated fatty acids in lipid membranes leading to the membrane dysfunction and cell disintegration.40,41 However, human neutrophils ROS production has been previously shown to be attenuated by many plants extracts such as Myrtus communis,16 Ceratonia siliqua42 and Punica granatum.43
Neutrophils are key cells in the pathophysiology of ischemia intestinal reperfusion. During the mesenteric reperfusion, activated neutrophils are probably a main vector between the intestine and target organs such as lung and liver.44 In this respect, we studied the effect of MCEO on intestinal ischemia/reperfusion induced-oxidative stress in rats. We showed, that IIR induced an oxidative stress status in rat small intestine, lung and liver as assessed by an increase of lipoperoxidation, depletion of non-enzymatic antioxidants such as –SH groups and GSH as well as deleterious effects on antioxidant enzyme activities such as SOD, CAT and GPx. Our results are in line with many previous reports indicating that IIR induced an oxidative stress damage in small intestine,45,46 lung47,48 and liver.49,50 However, ROS production starts during ischemia, then increases during reperfusion for 3 hours after reoxygenation.51 This increase in ROS production can be explained by transformation of a large part of oxygen directly to ROS. In fact, the accumulation of reduced equivalents during ischemia following the slowdown of the respiratory chain, the decline of the report adenosine diphosphate/oxygen during reperfusion as well as the reduction of ROS traps52 are responsible for direct transfer of electrons on oxygen and therefore an increase in free radical production, leading to increase of lipid peroxidation and exhaustion of all defense antioxidant systems in intestine,45 liver50 and lung.46 More importantly, MCEO pre-treatment protected against IIR-induced intestinal, lung and hepatic oxidative stress. These data fully corroborated all previously reported in vivo53 and in vitro31,54 antioxidant properties of myrtle leaves essential oils.
We also showed that IIR induced an increase in tissues calcium level, while the MCEO pretreatment has kept the calcium homeostasis. Indeed, during reperfusion, the poly(ribosomal DNA) polymerase (PRDP) activation and inactivation of some mitochondrial enzymes induce a depletion of intracellular adenosine triphosphate (ATP)55 and the increase of intracellular calcium level in the tissues. The same mechanism has been demonstrated for cerebral56 and heart57 tissues after ischemia/reperfusion injury.
Further, ischemia/reperfusion injury cause an iron and hydrogen peroxide overload in the tissues. In fact iron and H2O2 accumulation catalyzes the highly toxic hydroxyl radical (OH˙) production via the Fenton reaction. This radical is directly involved in most tissue damage of reperfusion, either by direct action or by activation of granulocytes. The use of a specific inhibitor of this radical such as allopurinol, oxypurinol, or pterin aldehyde, attenuate the intestinal hyperpermeability lesions observed during intestinal ischemia/reperfusion injury.58 MCEO attenuate the OH˙ production, by decreasing of H2O2 and free iron levels. The same mechanism for MCEO beneficial effect has been previously described of other organ system such as colon,16 stomach59 and brain.56
5. Conclusion
The findings of the current study provide evidence that MCEO protect against IIR-induced small intestine, lung and liver injury in rats. These beneficial effects of MCEO are partly related to their antioxidant and ROS scavenging activities as well as their opposite effects on intracellular metiators such as calcium, hydrogen peroxide and free iron.
Declaration of interest
The authors alone are responsible for the content of this paper.
Abbreviations
CAT | Catalase |
GPx | Glutathione peroxidase |
fMLF | N-Formyl-methionylleucyl-phenylalanine |
GSH | Reduced glutathione |
H2O2 | Hydrogen peroxide |
IIR | Intestinal ischemia/reperfusion |
MCEO | Myrtus communis essential oil |
MDA | Malondialdehyde |
MPO | Myeloperoxidase |
PMA | Phorbolmyristate acetate |
ROS | Reactive oxygen species |
–SH | Sulfhydryl groups |
SOD | Superoxide dismutase |
Acknowledgements
The authors would like to thank all members of U1149, “Center for Research on Inflammation” Paris France for assistance and helpful discussion. Financial support of INSERM and Tunisian Ministry of Higher Education and Scientific Research is gratefully acknowledged. Financial disclosures: none declared.
References
- W. Kübler and P. G. Spieckermann, Regulation of glycolysis in the ischemic and the anoxic myocardium, J. Mol. Cell. Cardiol., 1970, 1, 351–377 CrossRef.
- I. H. Mallick, W. Yang, M. C. Winslet and A. M. Seifalian, Ischemia-reperfusion injury of the intestine and protective strategies against injury, Dig. Dis. Sci., 2004, 49, 1359–1377 CrossRef CAS PubMed.
- A. E. Giakoustidis, D. E. Giakoustidis, S. Iliadis, G. Papageorgiou, K. Koliakou, N. Kontos, I. Taitzoglou, E. Botsoglou, V. Papanikolaou, K. Atmatzidis, D. Takoudas and A. Antoniadis, Attenuation of intestinal ischemia/reperfusion induced liver and lung injury by intraperitoneal administration of (−)-epigallocatechin-3-gallate, Free Radical Res., 2006, 40, 103–110 CrossRef CAS PubMed.
- A. Sizlan, A. Guven, B. Uysal, O. Yanarates, A. Atim, E. Oztas, A. Cosar and A. Korkmaz, Proanthocyanidin protects intestine and remote organs against mesenteric ischemia/reperfusion injury, World J. Surg., 2009, 33, 1384–1389 CrossRef PubMed.
- M. H. Schoenberg and H. C. Beger, Reperfusion injury after intestinal ischemia, Crit. Care Med., 1993, 21, 1376–1386 CrossRef CAS PubMed.
- P. A. Grace, Ischaemia-reperfusion injury, Br. J. Surg., 1994, 81, 637–647 CrossRef CAS PubMed.
- D. Giakoustidis, G. Papageorgiou, S. Iliadis, A. Giakoustidis, E. Kostopoulou, N. Kontos, E. Botsoglou, D. Tsantilas, V. Papanikolaou and D. Takoudas, The protective effect of alpha-tocopherol and GdCl3 against hepatic ischemia/reperfusion injury, Surg. Today, 2006, 36, 450–456 CrossRef CAS PubMed.
- J. K. Stechmiller, D. Treloar and N. Allen, Gut dysfunction in critically ill patients: a review of the literature, Am. J. Respir. Crit. Care Med., 1997, 6, 204–209 CAS.
- K. M. Nadkarni, Indian Materia Medica, Popular Prakashan Pvt, Ltd, Bombay, 3rd edn, 1989, vol. 1, p. 838 Search PubMed.
- M. Giacomo, Gas chromatographic-mass spectrometric investigation of the volatile components of myrtle berries (Myrtus communis L.), J. Chromatogr., 1983, 264, 304–311 CrossRef.
- O. Gortzi, S. Lalas, I. Chinou and J. Tsaknis, Reevaluation of bioactivity and antioxidant activity of Myrtus communis extract before and after encapsulation in liposomes, Eur. Food Res. Technol., 2008, 226, 583–590 CrossRef CAS.
- S. Serce, S. Ercisli, M. Sengul, K. Gunduz and E. Orhan, Antioxidant activities and fatty acid composition of wild grown myrtle (Myrtus communis L.) fruits, Pharmacogn. Mag., 2010, 6, 9–12 CrossRef CAS PubMed.
- C. Messaoud, A. Béjaoui and M. Boussaid, Fruit color, chemical and genetic diversity and structure of Myrtus communis L. var. italica Mill. morph populations, Biochem. Syst. Ecol., 2011, 39, 570–580 CrossRef CAS.
- H. Sebai, S. Selmi, K. Rtibi, A. Souli, N. Gharbi and M. Sakly, Lavender (Lavandula stoechas L.) essential oils attenuate hyperglycemia and protect against oxidative stress in alloxan-induced diabetic rats, Lipids Health Dis., 2013, 12, 1–9 CrossRef PubMed.
- J. El-Benna and P. M. Dang, Analysis of protein phosphorylation in human neutrophils, Methods Mol. Biol., 2007, 412, 85–96 CAS.
- M. A. Jabri, K. Rtibi, H. Tounsi, K. Hosni, A. Souli, J. El-Benna, L. Marzouki, M. Sakly and H. Sebai, Myrtle berries seeds aqueous extract inhibits in vitro human neutrophils myeloperoxidase and attenuates acetic acid-induced ulcerative colitis in rat, RSC Adv., 2015, 5, 64865–64877 RSC.
- L. Udby and N. Borregaard, Subcellular fractionation of human neutrophils and analysis of subcellular markers, Methods Mol. Biol., 2007, 412, 35–56 CAS.
- National Research Council, Guide for the care and the use of laboratory animals, National
Institute of Health, Bethesda, 1985, vol. 20, p. 85 Search PubMed.
- X. Gan, D. Liu, P. Huang, W. Gao, X. Chen and Z. Hei, Mast-cell-releasing tryptase triggers acute lung injury induced by small intestinal ischemia-reperfusion by activating PAR-2 in rats, Inflammation, 2012, 35, 1144–1153 CrossRef CAS PubMed.
- H. H. Draper and M. Hadley, Malondialdehyde determination as index of lipid peroxidation, Methods Enzymol., 1990, 186, 421–431 CAS.
- G. L. Ellman, Tissue sulfhydryl groups, Arch. Biochem. Biophys., 1959, 82, 70–77 CrossRef CAS PubMed.
- J. Sedlak and R. H. Lindsay, Estimation of total, protein bound, and non-protein sulfhydryl groups in tissue with Ellman's reagent, Anal. Biochem., 1968, 25, 192–205 CrossRef CAS PubMed.
- H. P. Misra and I. Fridovich, The role of superoxide anion in autoxidation of epinephrine and a simple assay for superoxide dismutase, J. Biol. Chem., 1972, 247, 3170–3175 CAS.
- H. Aebi, Catalase in vitro, Methods Enzymol., 1984, 105, 121–126 CAS.
- L. Flohé and W. A. Günzler, Assays of glutathione peroxidase, Methods Enzymol., 1984, 105, 114–121 Search PubMed.
- B. Dingeon, J. P. Ferry and A. Roullet, Automatic assay of blood sugar by Trinder's method, Ann. Biol. Clin., 1975, 33, 3–13 CAS.
- A. Leardi, M. Caraglia, C. Selleri, S. Pepe, C. Pizzi, R. Notaro, A. Fabbrocini, S. De Lorenzo, M. Musico, A. Abbruzzese, A. R. Bianco and P. Tagliaferri, Desferrioxamine increases iron depletion and apoptosis induced by ara-C of human myeloid leukaemic cells, Br. J. Haematol., 1998, 102, 746–752 CrossRef CAS PubMed.
- J. Stern and W. H. Lewis, The colorimetric estimation of calcium in serum with ocresolphthalein complexone, Clin. Chim. Acta, 1957, 2, 576–580 CrossRef CAS.
- E. F. Hartree, Determination of protein: a modification of the Lowry method that gives a linear photometric response, Anal. Biochem., 1972, 48, 422–427 CrossRef CAS PubMed.
- R. Gauthier, M. Gourai and J. Bellakhdar, A propos de l'huile essentielle de Myrtus communis L. var. italica récolté au Maroc. I. Rendements et compositions durant un cycle végétatif annuel, Biruniya, 1988, 4, 97–116 CAS.
- W. Aidi Wannes, B. Mhamdi, J. Sriti, M. Ben Jemia, O. Ouchikh, G. Hamdaoui, M. E. Kchouk and B. Marzouk, Antioxidant activities of the essential oils and methanol extracts from myrtle (Myrtus communis var. italica L.) leaf, stem and flower, Food Chem. Toxicol., 2010, 48, 1362–1370 CrossRef CAS PubMed.
- P. C. Pereira, M. J. Cebola and M. G. Bernardo-Gil, Evolution of the yields and composition of essential oil from Portuguese myrtle (Myrtus comunis L.) through the vegetative cycle, Molecules, 2009, 14, 3094–3105 CrossRef CAS PubMed.
- I. Jerkovic, A. Radonic and I. Borcic, Comparative study of leaf, fruit and flower essential oils from Croatian Myrtus communis L. during a one-year vegetative cycle, J. Essent. Oil Res., 2002, 14, 266–270 CrossRef CAS.
- M. Bocevska and H. Sovovà, Supercritical CO2 extraction of essential oil from yarrow, J. Supercrit. Fluids, 2007, 40, 360–367 CrossRef CAS.
- C. Messaoud and M. Boussaid, Myrtus communis Berry Color Morphs: A Comparative Analysis of Essential Oils, Fatty Acids, Phenolic Compounds, and Antioxidant Activities, Chem. Biodiversity, 2011, 8, 300–310 CAS.
- D. Yadegarinia, L. Gachkar, M. B. Rezaei, M. Taghizadeh, S. Alipoor-Astaneh and I. Rasooli, Biochemical activities of Iranian Mentha piperita L. and Myrtus communis L. essential oils, Phytochemistry, 2006, 67, 1249–1255 CrossRef CAS PubMed.
- M. Koelsch, R. Mallak, G. G. Graham, T. Kajer, M. K. Milligan, L. Q. Nguyen, D. W. Newsham, J. S. Keh, A. J. Kettle, K. F. Scott, J. B. Ziegler, D. I. Pattison, S. Fu, C. L. Hawkins, M. D. Rees and M. J. Davies, Acetaminophen (paracetamol) inhibits myeloperoxidase catalyze doxidant production and biological damage at therapeutically achievable concentrations, Biochem. Pharmacol., 2010, 79, 1156–1164 CrossRef CAS PubMed.
- J. Hill, T. Lindsay, C. R. Valeri, D. Shepro and H. B. Hechtman, A CD18 antibody prevents lung injury but not hypotension, J. Appl. Physiol., 1993, 74, 659–664 CrossRef CAS PubMed.
- B. M. Babior, NADPH oxidase: an update, Blood, 1999, 93, 1464–1476 CAS.
- J. M. Reimund, Oxidative stress in chronic inflammatory syndromes, Nutr. Clin. Metab., 2002, 16, 275–284 CrossRef CAS.
- B. M. Babior, Phagocytes and oxidative stress, Am. J. Med., 2000, 109, 33–44 CrossRef CAS PubMed.
- K. Rtibi, M. A. Jabri, S. Selmi, A. Souli, H. Sebai, J. El-Benna, M. Amri and L. Marzouki, Carob pods (Ceratonia siliqua L.) inhibit human neutrophils myeloperoxidase and in vitro ROS scavenging activity, RSC Adv., 2015, 5, 84207–84215 RSC.
- R. Bachoual, W. Talmoudi, T. Boussetta, F. Braut and J. El-Benna, An aqueous pomegranate peel extract inhibits neutrophil myeloperoxidase in vitro and attenuates lung inflammation in mice, Food Chem. Toxicol., 2011, 49, 1224–1228 CrossRef CAS PubMed.
- D. Carden, F. Xiao, C. Moak, B. H. Willis, S. Robinson-Jackson and S. Alexander, Neutrophil elastase promotes lung microvascular injury and proteolysis of endothelial cadherins, Am. J. Physiol., 1998, 275, 385–392 Search PubMed.
- Ö. Boybeyi, Y. D. Gunal, P. Atasoy, U. Kısa and M. K. Aslan, The effect of colchicine and low-dose methotrexate on intestinal ischemia/reperfusion injury in an experimental model, J. Clin. Pediatr. Surg., 2014, 49, 1471–1474 CrossRef PubMed.
- N. Okudan, M. Belviranlı, H. Gökbel, M. Oz and A. Kumak, Protective effects of curcumin supplementation on intestinal ischemia reperfusion injury, Phytomedicine, 2013, 20, 844–848 CrossRef CAS PubMed.
- W. Zhao, S. Zhou, W. Yao, X. Gan, G. Su, D. Yuan and Z. Hei, Propofol prevents lung injury after intestinal ischemia-reperfusion by inhibiting the interaction between mast cell activation and oxidative stress, Life Sci., 2014, 108, 80–87 CrossRef CAS PubMed.
- N. A. Bayomy, S. H. Elshafhey, R. H. ElBakary and E. Z. Abdelaziz, Protective effect of hesperidin against lung injury induced by intestinal ischemia/reperfusion in adult albino rats: histological, immunohistochemical and biochemical study, Tissue Cell, 2014, 46, 304–310 CrossRef CAS PubMed.
- Z. Fan, H. Jing, J. Yao, Y. Li, X. Hu, H. Shao, G. Shen, J. Pan, F. Luo and X. Tian, The protective effects of curcumin on experimental acute liver lesion induced by intestinal ischemia-reperfusion through inhibiting the pathway of NF-κB in a rat model, Oxid. Med. Cell. Longevity, 2014, 2014, 191624, DOI:org/10.1155/2014/191624.
- X. H. He, Q. W. Li, Y. L. Wang, Z. Z. Zhang, J. J. Ke, X. T. Yan and K. Chen, Transduced PEP-1-heme oxygenase-1 fusion protein reduces remote organ injury induced by intestinal ischemia/reperfusion, Med. Sci. Monit., 2015, 21, 1057–1065 CrossRef PubMed.
- R. Bolli, M. O. Jeroudi, B. S. Patel, O. I. Aruoma, B. Halliwell, E. K. Lai and P. B. McCay, Marked reduction of free radical generation and contractile dysfunction by antioxidant therapy begun at the time of reperfusion. Evidence that myocardial “stunning” is a manifestation of reperfusion injury, Circ. Res., 1989, 65, 607–622 CrossRef CAS PubMed.
- R. J. Korthuis and D. N. Granger, Reactive oxygen metabolites, neutrophils, and the pathogenesis of ischemic-tissue/reperfusion, Clin. Cardiol., 1993, 4(1), 19–26 CrossRef.
- A. Maxia, M. A. Frau, D. Falconieri, M. S. Karchuli and S. Kasture, Essential oil of Myrtus communis inhibits inflammation in rats by reducing serum IL-6 and TNF-alpha, Nat. Prod. Commun., 2011, 6, 1545–1548 CAS.
- N. Mimica-Dukić, D. Bugarin, S. Grbović, D. Mitić-Culafić, B. Vuković-Gacić, D. Orcić, E. Jovin and M. Couladis, Essential oil of Myrtusc ommunis L. as a potential antioxidant and antimutagenic agents, Molecules, 2010, 15, 2759–2770 CrossRef PubMed.
- S. Cuzzocrea, B. Zingarelli, G. Costantino, A. Szabó, A. L. Salzman, A. P. Caputi and C. Szabó, Beneficial effects of 3-aminobenzamide, an inhibitor of poly(ADP-ribose) synthetase in a rat model of splanchnic artery occlusion and reperfusion, Br. J. Pharmacol., 1997, 121, 1065–1074 CrossRef CAS PubMed.
- S. Kadri, S. Smine, M. Elayed, F. Limam, P. Cosette, M. Amri, E. Aouani and M. Mokni, Protective effect of grape seed and skin extract on cerebral ischemia in rat: implication of transition metals, Int. J. Stroke, 2015, 10, 415–424 CrossRef PubMed.
- A. Kimmoun, N. Ducrocq, N. Sennoun, K. Issa, C. Strub, J. M. Escanyé, S. Leclerc and B. Levy, Efficient extra- and intracellular alkalinization improves cardiovascular functions in severe lactic acidosis induced by hemorrhagic shock, Anesthesiology, 2014, 120, 926–934 CrossRef PubMed.
- P. E. Cañas, The role of xanthine oxidase and the effects of antioxidants in ischemia reperfusion cell injury, Acta Physiol., Pharmacol. Ther. Latinoam., 1999, 49, 13–20 Search PubMed.
- H. Sebai, M. A. Jabri, A. Souli, K. Hosni, S. Selmi, H. Tounsi, O. Tebourbi, S. Boubaker, J. El-Benna and M. Sakly, Protective effect of Artemisia campestris extract against aspirin-induced gastric lesions and oxidative stress in rat, RSC Adv., 2014, 4, 49831–49841 RSC.
|
This journal is © The Royal Society of Chemistry 2016 |
Click here to see how this site uses Cookies. View our privacy policy here.