DOI:
10.1039/C5RA25210E
(Paper)
RSC Adv., 2016,
6, 21187-21192
Significantly enhanced mechanical and electrical properties of epoxy nanocomposites reinforced with low loading of polyaniline nanoparticles
Received
27th November 2015
, Accepted 12th February 2016
First published on 12th February 2016
Abstract
The polyaniline (PANI)/epoxy nanocomposites with enhanced mechanical and electrical properties were prepared by three different techniques. Fourier transform infrared (FT-IR) spectroscopy and scanning electron microscopy (SEM) were used to study the chemical structure and surface morphology of the PANI nanoparticles, which were synthesized by the oxidation polymerization method. The effects of PANI loading and preparation method on the mechanical and electrical properties of PANI/epoxy nanocomposites were comparatively studied. The SEM images of the PANI/epoxy nanocomposites after doing the tensile test were used to study the dispersion of PANI nanoparticles in the epoxy matrix. The tensile strength of 5.0 wt% PANI/epoxy nanocomposites (107.27 MPa) was much higher than that of our previous PANI/epoxy nanocomposites (about 60.0 MPa) with the same PANI loading. The volume resistivity of the PANI/epoxy nanocomposites was also decreased compared to the reported literature. The toughness and Young's modulus of the PANI/epoxy nanocomposites were also studied and presented in this paper.
1. Introduction
Epoxy, considered as one of the most important engineered polymers, possesses a variety of high-technological applications, such as structural materials, flame retardant additives and anti-corrosion coatings due to its high tensile strength, Young's modulus, good thermal and chemical resistance, and so on.1–3 To make insulating epoxy (about 7.8 × 1013 ohm cm) conductive, different conductive nanofillers including carbon nanofibers, iron nanoparticles and carbon coated iron (Fe@C) nanoparticles were added into the epoxy matrix to widen the applications of epoxy in other fields.4–8 These nanofillers could improve the electrical conductivity of epoxy, however, these nanofillers would damage the other properties of epoxy. For example, adding the conductive metal nanoparticles could improve the electrical conductivity, but it increases the weight of final epoxy finishing, which will challenge the requirement of the device and structure miniaturization.5,9 The easy agglomeration of carbon nanofibers would reduce the mechanical property of the epoxy, and the surface treatment of the carbon nanofibers to improve the dispersion quality in the epoxy matrix would increase the cost for the fabrication of epoxy nanocomposites.10,11
Conductive polymers have attracted great attention due to their low cost, easy synthesis, flexibility, high capacitance and good environmental stability.12 Among all the conductive polymers, polyaniline (PANI) is one of the most studied one because of its high conductivity, good stability, low cost, light weight, easy doping and de-doping, etc.5,13 PANI could be applied in different fields including supercapacitors, environmental remediation and coupling agent between epoxy and nanofillers.13,14 In our previous work, the chemical bonding between PANI and epoxy has been formed and improved the mechanical property. After adding the PANI nanoparticles, the electrical conductivity of epoxy was increased without reducing other properties.5,15 The dispersion quality of the nanoparticles in the epoxy matrix is a great challenge for enhancing the properties of the epoxy nanocomposites.5 In order to reach high quality dispersion, the nanofillers were mixed with epon resin under stirring, and the curing agent was added into the mixture for curing the epoxy nanocomposites. However, the fabrication procedure effect on the properties of cured epoxy nanocomposites has not been reported.
In this work, the PANI nanoparticles synthesized with an oxidation polymerization method were used as nanofillers to prepare epoxy nanocomposites with three different methods. The Fourier transform infrared spectroscopy (FT-IR), X-ray diffraction (XRD), and scanning electron microscope (SEM) were used to characterize the chemical structure, crystallization, and morphology of the synthesized PANI nanoparticles. The effects of fabrication procedure and PANI loading on the mechanical property and electrical conductivity were studied. To study the dispersion of PANI nanoparticles in the epoxy matrix, the fracture surface of the PANI/epoxy nanocomposites after doing the tensile test was studied by the SEM images as well.
2. Experimental part
2.1 Materials
The epon 862 (bisphenol F epoxy) was provided by Miller-stephenson Chemical Company, Inc. Hexahydro-4-methylphthalic anhydride (98%) and acetone were obtained from Fisher Scientific. 2-Ethyl-4-methylimidazole (EMI), aniline (C6H7N), ammonium persulfate (APS, (NH4)2S2O8) and p-toluene sulfonic acid (PTSA, C7H8O3S, ≥98.5%) were purchased from Sigma Aldrich. All the chemicals were used as-received without any further treatment.
2.2 Preparation of PANI nanoparticles
PANI nanoparticles were synthesized by oxidation polymerization method. Briefly, the molar ratio was aniline
:
APS
:
PTSA = 6
:
3
:
5. For solution 1, PTSA (30 mmol) and APS (18 mmol) were dissolved in deionized water (200 mL) in a beaker, which was treated by sonication (Branson 8510) in the ice water bath for 1 hour mechanical stirring (SCILO-GEX OS20-Pro LCD Digital Overhead Stirrer 300 rpm). Solution 2 was aniline (36 mmol) dissolved in deionized water (50 mL). Solution 2 was then added into solution 1, and the mixture was sonicated for an additional 1.5 hour mechanical stirring (300 rpm) in the ice water bath for polymerization of aniline. Finally, the product was vacuum filtered and washed with deionized water and massive acetone for 3 times.
2.3 Preparation of PANI/epoxy nanocomposites with three different methods
In this project, we prepared the PANI/epoxy nanocomposites with three different methods to study the processing effect on mechanical and electrical properties of the epoxy nanocomposites. The following are details for the three methods to prepare PANI/epoxy nanocomposites.
2.3.1 “PANI/epoxy-1” method. After washed with acetone, the PANI product (0.4545 g, or 2.3684 g) was soaked with the hexahydro-4-methylphthalic anhydride (19.75 g) in a beaker. In order to remove acetone, the solution was put into the vacuum oven for 1 hour at 40 °C, then the PANI nanoparticles were immersed in hexahydro-4-methylphthalic anhydride without any disturbance overnight at room temperature. The solution was treated by sonication and mechanical stirring at 600 rpm for 1 hour at room temperature. Then the beaker was mechanically stirred at 200 rpm in a hot water bath at 80 °C. The epon monomer (25 g) and 2-ethyl-4-methylimidazole (0.25 g) were added into the beaker. Finally, after about 0.5 hour stirring, the solution was poured into the silicone rubber molds and cured at 100 °C for 3 hours, 140 °C for 4 hours and then cooled naturally to room temperature. The weight ratio was epon resin
:
hexahydro-4-methylphthalic anhydride
:
2-ethyl-4-methylimidazole = 25 g
:
19.75 g
:
0.25 g. The PANI/epoxy nanocomposites with 1.0 and 5.0 wt% PANI nanoparticles were synthesized by this method. The samples were named as 1.0 wt% PANI/epoxy-1 and 5.0 wt% PANI/epoxy-1, respectively.
2.3.2 “PANI/epoxy-2” method. After washed with acetone, the PANI product (0.4545 g, or 2.3684 g) was soaked with epon (25 g) in a beaker. To remove acetone, the beaker was put into the vacuum oven for 1 hour at 40 °C. The PANI nanoparticles were immersed in epon without any disturbance overnight at room temperature. The solution was treated by sonication and mechanical stirring 600 rpm for 1 hour at room temperature. The solution was then heated to 80 °C in a hot water bath under mechanical stirring 200 rpm. Hexahydro-4-methylphthalic anhydride (19.75 g) and 2-ethyl-4-methylimidazole (0.25 g) were added and stirred for about 0.5 hour. The solution was then poured into the silicone rubber molds and cured at 100 °C for 3 hours, 140 °C for 4 hours and then cooled naturally to room temperature. The PANI/epoxy nanocomposites with 1.0 and 5.0 wt% PANI nanoparticles were synthesized, respectively. The samples were named as 1.0 wt% PANI/epoxy-2, and 5.0 wt% PANI/epoxy-2, respectively.
2.3.3 “PANI/epoxy-3” method. After washed with acetone, the PANI product (0.4545 g, 0.918 g, 2.3684 g or 3.9130 g) was soaked with epon resin (25 g) in a beaker. To remove acetone, the beaker was put into the vacuum oven for 1 hour at 40 °C. The PANI nanoparticles were immersed in epon resin without any disturbance overnight at room temperature. Hexahydro-4-methylphthalic anhydride (19.75 g) was added into the solution. The solution was treated by sonication and mechanical stirring 600 rpm for 1 hour at room temperature. Then the solution was heated to 80 °C in a hot water bath under mechanical stirring 200 rpm. When the temperature was 80 °C, 2-ethyl-4-methylimidazole (0.25 g) was added into the solution and stirred for about 0.5 hour. The mixture was poured into the silicone rubber molds and cured at 100 °C for 3 hours, 140 °C for 4 hours and then cooled naturally to room temperature. The PANI/epoxy nanocomposites with 1.0, 2.0, 5.0 and 8.0 wt% PANI nanoparticles were synthesized by this method. The samples were named as 1.0 wt% PANI/epoxy-3, 2.0 wt% PANI/epoxy-3, 5.0 wt% PANI/epoxy-3 and 8.0 wt% PANI/epoxy-3, respectively. The cured pure epoxy was also synthesized with the same method without adding the PANI nanoparticles.
2.4 FT-IR, XRD and SEM characterizations
The chemical structure of the synthesized PANI nanoparticles was analyzed by a Fourier transform infrared spectroscopy (FT-IR), coupled with an ATR accessory (Bruker Vector 22) in the range of 500–4000 cm−1 with a resolution of 4 cm−1. The XRD was measured by D/max-rB wide-angle X-ray diffractometer at a Cu Kα wavelength of 0.154 nm. The morphologies of the synthesized PANI nanoparticles and the fracture surface of the cured pure epoxy and PANI/epoxy nanocomposites were examined by a Hitachi S4300 scanning electron microscopy (SEM). All the samples were sputter coated a thin layer of gold (about 5 nm) to ensure good imaging.
2.5 Mechanical properties
Tensile test was carried out following ASTM, D412-98a in a unidirectional tensile test machine (ADMET tensile strength testing system 2610). The parameters (displacement and force) were controlled by a digital controller (MTESTQuattro) with MTESTQuattro Materials Testing Software. The samples (dog-bone shaped) were designed according to the ASTM standard requirement and prepared as described for the epoxy nanocomposites in the molds. A crosshead speed of 1 mm min−1 was used and the strain (mm mm−1) was calculated by dividing the jogging displacement by the original gauge length. For each loading sample, we made 5 dog-bone, and did the tensile test to get the average data.
2.6 Volume resistivity
The volume resistivity, which is the inverse of electrical conductivity, was measured by testing the DC resistance along the disc samples with a diameter of about 60 mm with an Agilent 4339B high resistance meter (with the resistivity measurement up to 1016 Ω cm) and by a four-probe technique (C4S 4-Point Probe Head Cascade Microtech., the probe tips were made of tungsten carbide). For each loading sample, we measured the volume resistivity 5 times, then get the average data.
3. Results and discussion
Fig. 1(a) shows the FT-IR spectrum of PANI nanoparticles. The peaks at 1556 and 1470 cm−1 correspond to the C
C stretching vibration of the quinoid and benzenoid rings of the PANI polymer backbone, respectively.16 The peak at 1285 cm−1 is due to the C–N stretching vibration of the benzenoid unit, and the peak at around 1232 cm−1 is assigned to the C–H stretching vibration of the quinoid rings.17 The peak at 789 cm−1 corresponds to the out-of-plane bending of C–H in the substituted benzenoid ring. These prove that the PANI nanoparticles are the emeraldine salt form.12 The two broad diffraction peaks at 2θ = 20.1 and 25.3°, Fig. 1(b), correspond to the (100) and (110) crystallographic plane of the partially crystalline PANI nanoparticles.17 Fig. 1(c) shows the SEM image of the PANI nanoparticles. The PANI nanoparticles have a rough surface with an average diameter of 110 nm measured by the nano measurer software. All the results indicate that the PANI nanoparticles have been successfully synthesized.
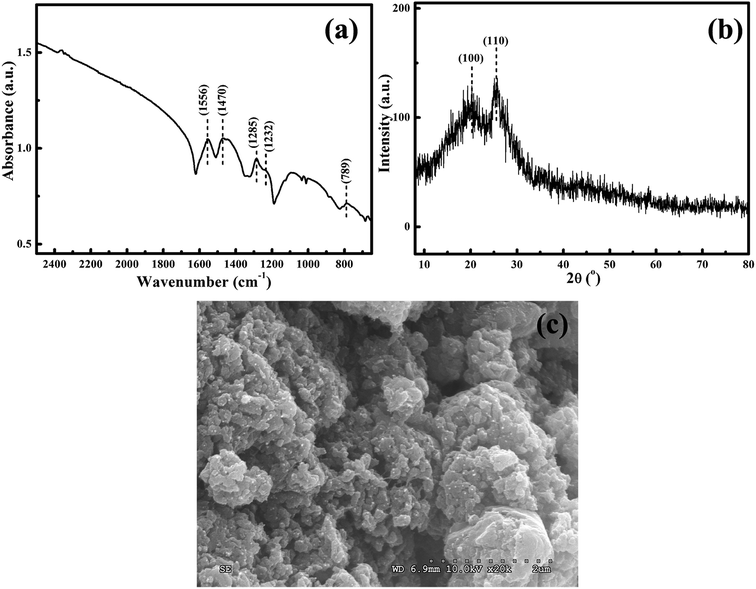 |
| Fig. 1 (a) FT-IR spectrum, (b) XRD pattern and (c) SEM microstructures of the synthesized PANI nanoparticles. | |
Fig. 2 shows the curve of tensile stress as a function of tensile strain for the cured pure epoxy and PANI/epoxy nanocomposites with different fabrication methods. The tensile strength of cured pure epoxy is 80.87 MPa, a little lower than that of pure epoxy with curing agent Epicure W.2 The PANI/epoxy nanocomposites with a PANI loading of 5.0 wt% show an enhanced tensile strength compared with that of cured pure epoxy, which is due to the covalent bondings formed between PANI nanoparticles and epoxy matrix.15,18 And the PANI/epoxy-3 nanocomposites show much higher tensile strength (107.27 MPa) than PANI/epoxy-2 (96.35 MPa) and PANI/epoxy-1 (88.81 MPa).
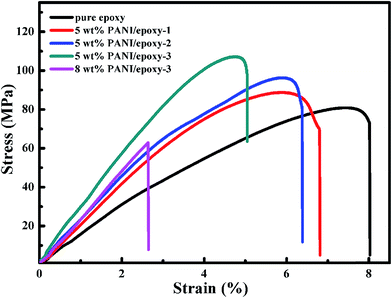 |
| Fig. 2 Stress–strain curves of cured pure epoxy, 5.0 wt% PANI/epoxy-1, 5.0 wt% PANI/epoxy-2, 5.0 wt% PANI/epoxy-3, and 8.0 wt% PANI/epoxy-3, respectively. | |
However, the tensile strength of 8.0 wt% PANI/epoxy-3 nanocomposites was decreased to 62.93 MPa, which is induced by the agglomeration of the PANI nanoparticles.5 The Young's modulus is the slope of the stress–strain curve at elastic portion. And the toughness can be determined by integrating the stress–strain curve. The Young's modules and toughness of pure epoxy and PANI/epoxy nanocomposites are summarized in Table 1. Adding the PANI nanoparticles into the epoxy matrix would reduce the toughness of epoxy but enhance the Young's modulus of the epoxy. These results indicate that the stiffness is improved by sacrificing the toughness, which is consistent with the results in magnetic epoxy nanocomposites and layered silicates/epoxy nanocomposites.19,20
Table 1 Young's modules and toughness of pure epoxy and PANI/epoxy nanocomposites
|
Toughness (J m−3 104) |
Young's modulus (GPa) |
Pure epoxy |
406.0 |
1.6 |
5.0 wt% PANI/epoxy-1 |
395.0 |
2.2 |
5.0 wt% PANI/epoxy-2 |
387.0 |
2.3 |
5.0 wt% PANI/epoxy-3 |
331.0 |
3.1 |
8.0 wt% PANI/epoxy-3 |
83.0 |
2.6 |
Fig. 3 shows the SEM images of the fracture surface of the cured pure epoxy and PANI/epoxy nanocomposites with different synthesized methods and PANI loadings. A relatively smooth fracture surface with “river-like” patterns is observed in the SEM images of pure epoxy, Fig. 3(a), indicating a brittle failure because of the rapid crack propagation.21 Adding the PANI nanoparticles into the epoxy matrix, the fracture surface of the PANI/epoxy nanocomposites becomes much rougher, Fig. 3(b–e). However, compared with 5.0 wt% PANI/epoxy-1 and 5.0 wt% PANI/epoxy-2 nanocomposites, Fig. 3(b and c), the fracture surface of 5.0 wt% PANI/epoxy-3 nanocomposites is relatively smoother, indicating that the PANI nanoparticles have a good dispersion in the epoxy matrix, Fig. 3(d). Due to the good nanoparticle dispersion in the epoxy matrix and the covalent bondings formed between PANI nanoparticles and epoxy matrix,18 the tensile strength (107.27 MPa) of 5.0 wt% PANI/epoxy-3 nanocomposites is much higher than previously reported PANI/epoxy nanocomposites (about 60.0 MPa) at the same PANI loading.5 The agglomeration of the PANI nanoparticles is observed in the fracture surface of 8.0 wt% PANI/epoxy-3 nanocomposites, Fig. 3(e), and leads to a decreased tensile strength (62.93 MPa). However, the 8.0 wt% PANI/epoxy-3 nanocomposites still show higher tensile strength (62.93 MPa) than previously reported PANI nanocomposites with 10.0 wt% PANI loading (about 55 MPa).5
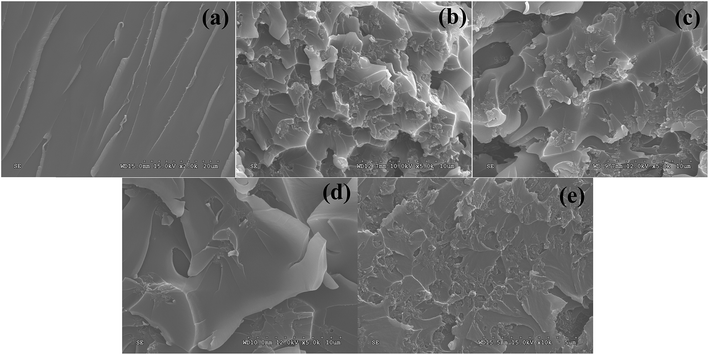 |
| Fig. 3 SEM microstructures of the fracture surface of (a) cured pure epoxy, 5.0 wt% PANI/epoxy nanocomposites synthesized by (b) “5.0 wt% PANI/epoxy-1”, (c) “5.0 wt% PANI/epoxy-2”, and (d) “5.0 wt% PANI/epoxy-3”, and (e) 8.0 wt% PANI/epoxy-3, respectively. | |
Fig. 4 depicts the volume resistivity of the cured pure epoxy and its PANI nanocomposites with different fabrication methods. The volume resistivity of cured pure epoxy is about 1.1629 × 1016 Ω cm. The volume resistivity is decreased by adding the conductive PANI nanoparticles into the epoxy matrix. When the PANI loading is 5.0 wt%, the volume resistivity of the nanocomposites is decreased to 2.06785 × 1012 Ω cm for PANI/epoxy-1 nanocomposites, 2.3913 × 1012 Ω cm for PANI/epoxy-2 nanocomposites. However, for the 5.0 wt% PANI/epoxy-3 nanocomposites, the volume resistivity is decreased to 3.0928 × 1011 Ω cm, indicating that the PANI nanoparticles are well dispersed in the epoxy matrix, which is consistent with the SEM observation of the fracture surface of PANI/epoxy nanocomposites. When increasing the PANI loading to 8.0 wt%, the volume resistivity of the PANI/epoxy-3 nanocomposites was decreased to 3.8017 × 1010 Ω cm, even lower than that of our previously reported PANI/epoxy nanocomposites with 10.0 wt% PANI nanoparticles (about 1.0 × 1011 Ω cm).5 Fig. 5 depicts the volume resistivity of PANI/epoxy-3 nanocomposites with different PANI nanoparticle loadings. When 1.0 and 2.0 wt% PANI nanoparticles were added into the epoxy matrix, the volume resistivity was decreased to 1.5576 × 1014, and 1.31943 × 1014 Ω cm for 1.0 wt% PANI/epoxy-3, and 2.0 wt% PANI/epoxy-3 nanocomposites, respectively. However, when the PANI loading was increased to 5.0 wt%, the volume resistivity of 5.0 wt% PANI/epoxy-3 nanocomposites was decreased significantly, almost 4 orders of magnitude lower than that of the cured pure epoxy (1.1629 × 1016 Ω cm). The variation of volume resistivity of the PANI/epoxy-3 nanocomposites with different loadings of PANI nanoparticles indicated the formation of percolated network. At lower PANI loading, the PANI nanoparticles rarely contact each other, which makes the electron hopping more difficult because of the large spacing between particles.22 When the particle loading was increased to higher percent, the percolation network was formed by nanoparticles in the epoxy matrix at a certain nanoparticle loading, making the electron hopping much easier between neighboring nanoparticles.23 Similar phenomenon is also observed the epoxy nanocomposites with iron-core–carbon-shell nanoparticles.4
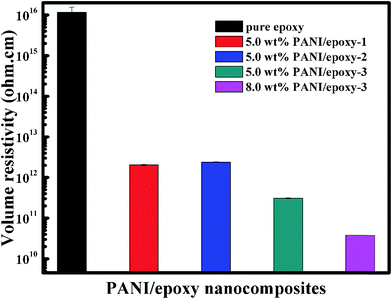 |
| Fig. 4 Volume resistivity of pure epoxy, 5.0 wt% PANI/epoxy-1, 5.0 wt% PANI/epoxy-2, 5.0 wt% PANI/epoxy-3, and 8.0 wt% PANI/epoxy-3, respectively. | |
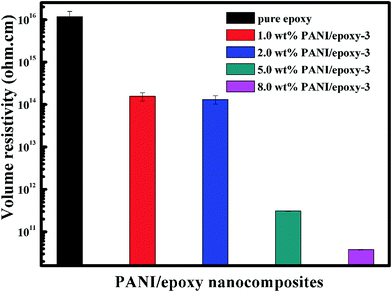 |
| Fig. 5 Volume resistivity of pure epoxy, 1.0 wt% PANI/epoxy-3, 2.0 wt% PANI/epoxy-3, 5.0 wt% PANI/epoxy-3, and 8.0 wt% PANI/epoxy-3, respectively. | |
4. Conclusions
The PANI nanoparticles prepared with oxidation polymerization method have been successfully prepared as fillers for epoxy nanocomposites with three different approaches. The PANI nanoparticles have a good dispersion in the epoxy matrix, which is observed in the SEM result of fracture surface of the PANI/epoxy nanocomposites after doing the tensile test. Compared with PANI/epoxy-1 and PANI/epoxy-2 nanocomposites, the PANI/epoxy-3 nanocomposites showed better electrical conductivity (3.0928 × 1011 Ω cm) and mechanical property (107.27 MPa), indicating that the synthesized process had a significant effect on the properties of PANI/epoxy nanocomposites. Both electrical conductivity and mechanical property (tensile strength) were significantly enhanced at 5.0 wt% PANI loading, compared with pure epoxy (1.1629 × 1016 Ω cm and 80.87 MPa). And 8.0 wt% PANI/epoxy-3 nanocomposites showed better electrical conductivity (3.8017 × 1010 Ω cm) and mechanical property (tensile strength 62.93 MPa) than previously reported PANI/epoxy nanocomposites with 10.0 wt% PANI loading (1.0 × 1011 Ω cm and 55.0 MPa). Adding the PANI nanoparticles into the epoxy matrix could increase the Young's modulus of the epoxy, but decrease the toughness of the epoxy.
Acknowledgements
This project is financially supported by the start-up funds of University of Tennessee, Knoxville.
Notes and references
- X. Zhang, O. Alloul, Q. He, J. Zhu, M. J. Verde, Y. Li, S. Wei and Z. Guo, Polymer, 2013, 54, 3594–3604 CrossRef CAS.
- J. Guo, X. Zhang, H. Gu, Y. Wang, X. Yan, D. Ding, J. Long, S. Tadakamalla, Q. Wang, M. A. Khan, J. Liu, X. Zhang, B. L. Weeks, L. Sun, D. P. Young, S. Wei and Z. Guo, RSC Adv., 2014, 4, 36560–36572 RSC.
- H. Gu, J. Guo, H. Wei, S. Guo, J. Liu, Y. Huang, M. A. Khan, X. Wang, D. P. Young, S. Wei and Z. Guo, Adv. Mater., 2015, 27, 6277–6282 CrossRef CAS PubMed.
- X. Zhang, O. Alloul, J. Zhu, Q. He, Z. Luo, H. A. Colorado, N. Haldolaarachchige, D. P. Young, T. D. Shen, S. Wei and Z. Guo, RSC Adv., 2013, 3, 9453–9464 RSC.
- X. Zhang, Q. He, H. Gu, H. A. Colorado, S. Wei and Z. Guo, ACS Appl. Mater. Interfaces, 2012, 5, 898–910 Search PubMed.
- M. Amjadi, A. Pichitpajongkit, S. Lee, S. Ryu and I. Park, ACS Nano, 2014, 8, 5154–5163 CrossRef CAS PubMed.
- L. J. Lee, C. Zeng, X. Cao, X. Han, J. Shen and G. Xu, Compos. Sci. Technol., 2005, 65, 2344–2363 CrossRef CAS.
- R. Rahimi, M. Ochoa, W. Yu and B. Ziaie, ACS Appl. Mater. Interfaces, 2015, 7, 4463–4470 CAS.
- T. R. Hendricks, J. Lu, L. T. Drzal and I. Lee, Adv. Mater., 2008, 20, 2008–2012 CrossRef CAS.
- J. K. W. Sandler, J. E. Kirk, I. A. Kinloch, M. S. P. Shaffer and A. H. Windle, Polymer, 2003, 44, 5893–5899 CrossRef CAS.
- J. Zhu, J. Kim, H. Peng, J. L. Margrave, V. N. Khabashesku and E. V. Barrera, Nano Lett., 2003, 3, 1107–1113 CrossRef CAS.
- H. Gu, H. Wei, J. Guo, N. Haldolaarachige, D. P. Young, S. Wei and Z. Guo, Polymer, 2013, 54, 5974–5985 CrossRef CAS.
- H. Gu, J. Guo, X. Zhang, Q. He, Y. Huang, H. A. Colorado, N. Haldolaarachchige, H. Xin, D. P. Young, S. Wei and Z. Guo, J. Phys. Chem. C, 2013, 117, 6426–6436 CAS.
- H. Gu, J. Guo, H. Wei, Y. Huang, C. Zhao, Y. Li, Q. Wu, N. Haldolaarachchige, D. P. Young, S. Wei and Z. Guo, Phys. Chem. Chem. Phys., 2013, 15, 10866–10875 RSC.
- H. Gu, S. Tadakamalla, Y. Huang, H. A. Colorado, Z. Luo, N. Haldolaarachchige, D. P. Young, S. Wei and Z. Guo, ACS Appl. Mater. Interfaces, 2012, 4, 5613–5624 CAS.
- H. Gu, J. Guo, Q. He, S. Tadakamalla, X. Zhang, X. Yan, Y. Huang, H. A. Colorado, S. Wei and Z. Guo, Ind. Eng. Chem. Res., 2013, 52, 7718–7728 CrossRef CAS.
- H. Gu, Y. Huang, X. Zhang, Q. Wang, J. Zhu, L. Shao, N. Haldolaarachchige, D. P. Young, S. Wei and Z. Guo, Polymer, 2012, 53, 801–809 CrossRef CAS.
- X. Zhang, Q. He, H. Gu, S. Wei and Z. Guo, J. Mater. Chem. C, 2013, 1, 2886–2899 RSC.
- J. Zhu, S. Wei, J. Ryu, L. Sun, Z. Luo and Z. Guo, ACS Appl. Mater. Interfaces, 2010, 2, 2100–2107 CAS.
- C. Zilg, R. Mülhaupt and J. Finter, Macromol. Chem. Phys., 1999, 200, 661–670 CrossRef CAS.
- S. Wang, Z. Liang, P. Gonnet, Y. H. Liao, B. Wang and C. Zhang, Adv. Funct. Mater., 2007, 17, 87–92 CrossRef CAS.
- J. Zhu, S. Wei, Y. Li, L. Sun, N. Haldolaarachchige, D. P. Young, C. Southworth, A. Khasanov, Z. Luo and Z. Guo, Macromolecules, 2011, 44, 4382–4391 CrossRef CAS.
- L. I. Trakhtenberg, E. Axelrod, G. N. Gerasimov, E. V. Nikolaeva and E. I. Smirnova, J. Non-Cryst. Solids, 2002, 305, 190–196 CrossRef CAS.
|
This journal is © The Royal Society of Chemistry 2016 |