DOI:
10.1039/C5RA23401H
(Paper)
RSC Adv., 2016,
6, 12519-12524
Identification of a new curcumin synthase from ginger and construction of a curcuminoid-producing unnatural fusion protein diketide-CoA synthase::curcumin synthase†
Received
6th November 2015
, Accepted 18th January 2016
First published on 22nd January 2016
Abstract
The biosynthesis and metabolic engineering of curcuminoids received considerable attention for their important pharmaceutical properties. In the present study, a new curcumin synthase (ZoCURS) was identified from ginger (Zingiber officinale). Notably, ZoCURS efficiently accepts 3-(4-hydroxyphenyl)propionyl-CoA to produce tetrahydrobisdemethoxycurcumin, which would be meaningful to further understand the biosynthesis of curcuminoids in ginger. In addition, a curcuminoid-producing unnatural fusion protein diketide-CoA synthase::curcumin synthase (DCS::CURS) was constructed. Comparing to ZoCURS, DCS::CURS indicated similar substrate specificities and catalytic potentials to catalyze the formation of various curcuminoids, however, the yield of curcuminoids produced by DCS::CURS was obviously increased, which would be useful for metabolic engineering of pharmaceutically important curcuminoid analogs, particularly including asymmetric dihydrocurcuminoids such as 3-(4-hydroxyphenyl)propionyl-feruloylmethane, 3-(4-hydroxyphenyl)propionyl-p-coumaroylmethane, and 3-(4-hydroxyphenyl)propionyl-cinnamoylmethane.
1. Introduction
Curcumin, the major component of Curcuma longa, is widely used as traditional medicine and food additive for its promising bioactivities, unique coloring and aromatic properties.1,2 Due to its important pharmaceutical properties such as anti-inflammatory, antitumor, and anti-oxidation activities, the biosynthesis of curcumin has attracted much attention.3–6 The recent characterization of two novel type III polyketide synthases from C. longa termed as diketide-CoA synthase (DCS) and curcumin synthase (CURS) well established the biosynthesis pathway of curcumin in C. longa.7,8 Firstly, DCS catalyzes the condensation of feruloyl-CoA and malonyl-CoA to produce feruloyldiketide-CoA. And then, CURS catalyzes the conversion of feruloyldiketide-CoA to β-keto acid and sequentially condenses with another molecule of feruloyl-CoA to produce curcumin (Scheme 1A).7,8 Besides, a novel type III polyketide synthase (curcuminoid synthase, CUS) from rice plant Oryza sativa catalyzes one-pot formation of bisdemethoxycurcumin from the condensation of two molecules of p-coumaroyl-CoA and one molecule of malonyl-CoA (Scheme 1B).9–11 Although curcuminoids have never been isolated from O. sativa, the identification of CUS in O. sativa is still interesting and its adaptive function is expecting to be found. In contrast, ginger (Zingiber officinale) as another major source of curcuminoids, enzymes involved in the biosynthesis of curcuminoids in ginger have not been characterized. Thus, characterization of functionally related enzymes from ginger would be advantage to further understand the biosynthesis of curcuminoids in this plant.
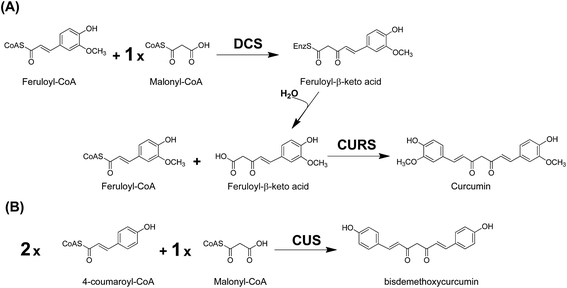 |
| Scheme 1 Proposed mechanisms of curcuminoids formation. (A) Curcumin from two molecules of feruloyl-CoA and one molecule of malonyl-CoA by DCS and CURS; (B) bisdemethoxycurcumin from two molecules of 4-coumaroyl-CoA and one molecule of malonyl-CoA by CUS. | |
On the other hand, advances in biogenetic engineering technologies facilitate to manipulate and partially/entirely reconstruct a biosynthesis pathway in heterologous microorganisms for the production of specific metabolites with high-yields for medicine.12–15 Among of the approaches used in the biosynthetic-process manipulation and reconstruction, generation of unnatural fusion protein by insertion of artificial linkers between sequential biosynthetic enzymes, which mimics the natural co-localization of proteins in cells and/or the physical channeling of reactive intermediates between enzyme active sites, is of great promising to simplify the biosynthetic process and increase the metabolic efficiency.16–18 Given the important pharmaceutical properties of curcuminoids, reconstruction of the biosynthesis pathway in heterologous microorganisms to produce curcuminoids is getting increasingly attractive. However, comparing to DCS and CURS synergically catalyzed the formation of curcuminoids, CUS from O. sativa possessing the remarkable function of one-pot formation of curcuminoids is more convenient and thus preferred to be used in the construction of metabolically engineered microorganisms for curcuminoids production.19–22 We propose that construction of an unnatural fusion protein DCS::CURS possibly achieve the synergistic function of DCS and CURS, and the fusion protein would be convenient to be used in metabolic engineering curcuminoids production in microorganisms.
Herein, we report the identification of a new curcumin synthase (ZoCURS) from ginger, and functional analysis of DCS::CURS, a curcuminoid-producing unnatural fusion protein constructed by inserting of an artificial linker between a known diketide-CoA synthase (DCS) from C. longa and the newly identified ZoCURS.
2. Materials and methods
2.1. Chemicals
Cinnamoyl-CoA, p-coumaroyl-CoA, feruloyl-CoA, and 3-(4-hydroxyphenyl)propionyl-CoA were enzymatically synthesized according to reported methods.23 Malonyl-CoA, curcumin (1a), bisdemethoxycurcumin (2a), 3-methoxy-4-hydroxybenzalacetone (dehydrozingerone) (1b), 4-hydroxybenzalacetone (2b), and 4-hydroxybenzylacetone (raspberry ketone) (4b) were purchased from Sigama (St Louis, MO, USA). Dicinnamoylmethane (3a), tetrahydrobisdemethoxycurcumin (THBDC) (4a) were synthesized according to reported methods.24,25
2.2. cDNA cloning and expression
Total RNA samples were prepared from 100 mg of young fresh rhizomes of C. longa and leaves of Z. officinale, respectively, using Plant RNA kit (Omega) and reverse-transcribed using Reverscript (Wakon) and oligo-dT primer (RACE 32 = 5′-GGC CAC GCG TCG ACT AGT ACT TTT TTT TTT TTT TTT T-3′). The full-length cDNA of DCS and ZoCURS were amplified by PCR using N- and C-terminal primers synthesized according to the sequence registered (NCBI accession numbers: AB495006 for DCS, and DQ486012 for ZoCURS) (primer sequences could be found in ESI, S1†). The amplified DNA was cloned between the BamHI and HindIII sites of pET28a (Novagen) to obtain the constructed plasmids pET28a-DCS and pET28a-ZoCURS. To construct the DCS::CURS fusion gene, the stop codon of DCS was removed and a three amino acid linker (Gly-Ser-Gly) was introduced between the open reading frames of DCS and ZoCURS by site directed-mutagenesis through two rounds of PCR (primer sequences could be found in ESI, S1†). It resulted in a 2.349 kb fused gene encoding DCS, the tripeptide linker, and ZoCURS. Finally, the fusion gene was inserted into the BamHI/HindIII site of pET28a (Novagen) to obtain the constructed plasmid pET28a-DCS::CURS. After confirmation of the sequences, the plasmid of pET28a-DCS, pET28a-ZoCURS and pET28a-DCS::CURS was transformed into E. coli BL21(DE3), respectively. The cells harboring the corresponding plasmid were cultured to an A600 of 0.6 in LB medium containing 50 μg mL−1 kanamycin and induced by 0.6 mM IPTG at 23 °C for 16 h.
2.3. Enzyme purification
The E. coli cells were harvested by centrifugation and resuspended in 40 mM potassium phosphate buffer (KPB) (pH = 7.9) containing 100 mM NaCl, 5 mM imidazole. Cell lysis was carried out by sonication and centrifuged at 12
000g at 4 °C for 30 min. The supernatant containing crude recombinant protein was collected and passed through a column of Ni-NTA His-Bind™ Resin (CWBIO, Beijing, China) containing Ni2+ as an affinity ligand. After washing with 20 mM KPB (pH = 7.9) containing 500 mM NaCl and 40 mM imidazole, the recombinant enzyme was finally eluted with 15 mM KPB (pH = 7.8) containing 10% glycerol and 500 mM imidazole. After desalted and concentrated by Amicon Ultra-15 centrifugal concentrators (Millipore, MI, USA), the recombinant protein was finally kept in 20 mM potassium phosphate buffer (pH = 8.0) containing 1 mM EDTA and 10% glycerol. The determination of protein concentration was carried out according to BCA method (Protein Assay, BIOMIGA) using bovine serum albumin as standard. The expression yield of DCS, ZoCURS, and DCS::CURS is 1.0 mg L−1, 1.6 mg L−1, and 1.5 mg L−1, respectively.
2.4. Enzyme reaction
The standard reaction mixture contained 100 μM starter substrate (cinnamoyl-CoA, p-coumaroyl-CoA, feruloyl-CoA, and 3-(4-hydroxyphenyl)propionyl-CoA), 100 μM extender substrate (malonyl-CoA), 100 mM KPB (pH = 7.5), and 166 nM of purified recombinant enzyme in a total volume of 500 μL. And reactions were incubated at 37 °C for 8 h before being quenched with 40 μL 20% HCl. The products were extracted twice with 800 μL ethyl acetate, and the organic layer was evaporated to dryness. The residual materials were dissolved in 100 μL of methanol for LCMS-IT-TOF (Shimadzu, Japan) analysis. The HPLC was equipped with an Agilent Eclipse XDB C18 Column (250 × 4.6 mm I.D. 5 μm) and eluted with a linear acetonitrile gradient (30–100% over 30 min) in water at a flow rate of 1.0 mL min−1. The enzymatic products were identified and quantified using corresponding authentic compounds.
2.5. Time course of DCS::CURS catalyzing the production of curcumin (1a) and 3-methoxy-4-hydroxybenzalactone (1b)
100 μM feruloyl-CoA, 100 μM malonyl-CoA, 100 mM KPB (pH = 7.5), and 83.4 nM of purified recombinant enzyme in a total volume of 500 μL was incubated at 30 °C for 5–360 min. The reaction mixture was processed according to the method mentioned in Section 2.4, and the enzymatic products were quantified using standard compounds.
3. Results and discussion
3.1. cDNA cloning and enzyme purification
The full length cDNA of DCS was directly amplified from C. longa according to the known sequence of DCS (NCBI accession numbers AB495006). Considering the extensive reports of curcumin synthases from C. longa (CURS1, CURS2, and CURS3),7,26 we have no attempt to screen and clone CURS from C. longa. However, enzymes involved in the biosynthesis of curcuminoids in ginger have not been characterized before. Although a type III polyketide synthase named ZoPKS has been proposed to be involved in the biosynthesis of phenylbutanone derivatives in ginger. Its unambiguous activity is currently unknown.27 Therefore, we cloned and expressed ZoPKS (NCBI accession numbers DQ486012) based on its known full length. With a series of in vitro functional analysis, recombinant protein ZoPKS was identified to be a new curcumin synthase, named ZoCURS. As the construction of the fusion protein DCS::CURS, the stop codon of DCS was removed and a three amino acid linker (Gly-Ser-Gly) was introduced between the open reading frames of DCS and ZoCURS by site directed-mutagenesis through two rounds of PCR.
DCS, ZoCURS, and the unnatural fusion protein DCS::CURS His-tagged at their N terminus were produced in E. coli BL21 (DE3) and purified by a nickel chelated affinity column to obtain three recombinant proteins of 42.04 kDa, 43.24 kDa, and 85.47 kDa, respectively (SDS-page could be found in ESI, S2†).
3.2. Analysis of the reactions catalyzed by ZoCURS
In the absence of DCS, only a trace amount of curcumin (1a) was detectable by LC-MS in the reaction of ZoCURS with feruloyl-CoA (1) and malonyl-CoA. However, the yield of curcumin (1a) was dramatically increased by co-incubation DCS and ZoCURS with feruloyl-CoA and malonyl-CoA (Scheme 2A and Fig. 1A). Further analysis of the substrate specificity revealed that ZoCURS, in the presence of DCS, could also accept p-coumaroyl-CoA (2) and cinnamoyl-CoA (3) to produce bidemethoxycurcumin (2a) and dicinnamoylmethane (3a), respectively (Scheme 2A and Fig. 1B–C). In addition, C6–C4 scaffolds of 3-methoxy-4-hydroxybenzalacetone (dehydrozingerone) (1b) and 4-hydroxybenzalacetone (2b) could also be produced by ZoCURS (co-incubated with DCS) as by-product from feruloyl-CoA and p-coumaroyl-CoA, respectively. Very interestingly, when DCS and ZoCURS were co-incubated in the presence of 3-(4-hydroxyphenyl)propionyl-CoA (4) and malonyl-CoA, tetrahydrobisdemethoxycurcumin (THBDC) (4a) and 4-hydroxybenzylacetone (4b) were produced (Scheme 2B and Fig. 1D). All structures of the enzymatic products were unambiguously characterized by comparing their UV and MS data as well as retention time (Rt) on HPLC chromatogram with those of the authentic compounds (Fig. 1, and S3–S4†). It is generally accepted that the biosynthesis of THBDC (4a) and gingerol-related compounds in ginger involved in a NAD(P)H-dependent reductase to selectively reduce the unsaturated double bonds.28 However, ZoCURS from ginger efficiently accepts 3-(4-hydroxyphenyl)propionyl-CoA as a starter to produce diarylheptanoid scaffold of THBDC (4a), which suggests that THBDC (4a) and gingerol-related compounds in Z. officinale are possible to be directly synthesized from 3-(4-hydroxyphenyl)propionic acid, excepting for the possibility of that from p-coumaric acid. In addition, it should be notable that 4-hydroxybenzylacetone (raspberry ketone) (4b) was previously reported to be biogenetically synthesized from 4-hydroxybenzalacetone which was formed by benzalacetone synthase (BAS) from the condensation of p-coumaroyl-CoA and one molecule of malonyl-CoA.29–32 Enzymes directly involved in the synthesis of 4-hydroxybenzylacetone (4b) from 3-(4-hydroxyphenyl)propionyl-CoA has never been reported. The C6–C4 scaffolds of 1b, 2b, and 4b should be non-enzymatic hydrolysis and subsequent decarboxylation of diketide-CoA intermediates produced by DCS.
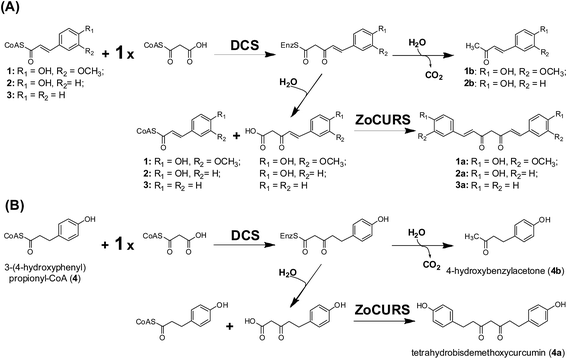 |
| Scheme 2 Proposed mechanisms of curcuminoids formation catalyzed by DCS::CURS. | |
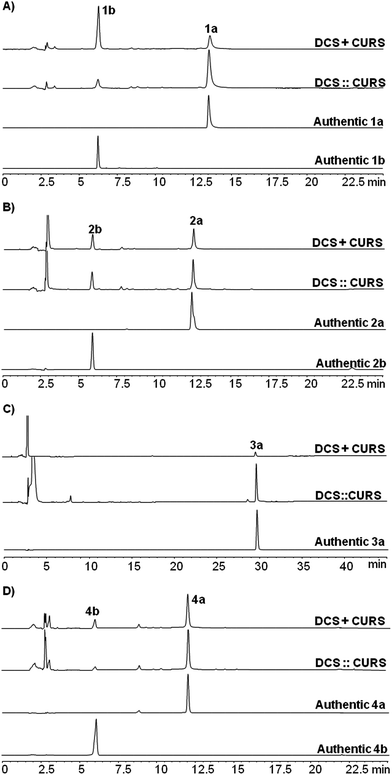 |
| Fig. 1 HPLC chromatograms of the reaction products produced by ZoCURS and DCS::CURS from malonyl-CoA and varied substrates (A) feruloyl-CoA (A360 nm), (B) 4-coumaroyl-CoA (A360 nm), (C) cinnamoyl-CoA (A360 nm), and (D) 3-(4-hydroxyphenyl)propionyl-CoA (A280 nm). | |
Although curcuminoids have been extensively reported from ginger, ZoCURS is the first polyketide synthase functionally identified to be involved in the biosynthesis of curcuminoids in ginger. Particularly, enzymatic formation of THBDC (4a) from 3-(4-hydroxyphenyl)propionyl-CoA by wild plant polyketide synthases has not been previously reported. Sequence alignment reveals that ZoCURS shares 81.03% amino acid sequence identity with CURS1 from C. longa, the highly conserved Cys-His-Asn catalytic triad in other plant polyketide synthases is also observed in ZoCURS. Additionally, ZoCURS shares almost the same amino residues lining around the catalytic cavity in CURS1, including Phe215, Phe265, Phe267, Gln338, and Met137. It was proposed that a hydrophobic cavity surrounded by the side chains of Phe215, Phe265, Phe267, and Gly211 in CURS1 was very important for CURS1 to use a β-keto acid as an extender substrate.8 The observation of Phe215, Phe265, and Phe267 in ZoCURS suggests that ZoCURS probably utilizes similar catalytic machinery to that of CURS1.
3.3. Analysis of the reactions catalyzed by DCS::CURS
Comprehensive investigations on catalytic functions of the fusion protein DCS::CURS revealed that DCS::CURS showed similar substrate specificities and catalytic potentials to those of ZoCURS (co-incubate with DCS). The fusion protein DCS::CURS could also efficiently accept feruloyl-CoA, p-coumaroyl-CoA, cinnamoyl-CoA, and 3-(4-hydroxyphenyl)propionyl-CoA to produce various diarylheptanoid scaffolds of curcumin (1a), bisdemethoxycurcumin (2a), dicinnamoylmethane (3a), and THBDC (4a), respectively (Fig. 1). Comparing to the reactions catalyzed by DCS and ZoCURS co-incubation, the yield of curcumin, bisdemethoxycurcumin, dicinnamoylmethane, and THBDC produced by DCS::CURS was dramatically increased (Fig. 2). In contrast, the yields of the by-products of 1b, 2b, and 4b decreased (Fig. 2). The fusion protein DCS::CURS showed the optimal pH and temperature at 7.5 and 30 °C, respectively (ESI, S5†). Investigation on the time course of the unnatural fusion protein DCS::CURS using feruloyl-CoA and malonyl-CoA as substrates revealed that the production rate of the major product curcumin (1a) and the minor product 3-methoxy-4-hydroxybenzalacetone (1b) varied at different time points. In the first 60 min after the reaction initiation, the velocity of curcumin synthesis was very slow, and sharply increased in the following 3 h. In contrast, the accumulation of the minor product 3-methoxy-4-hydroxybenzalacetone (1b) in the reaction mixture kept increasing in the first 60 min, and then reached saturation (ESI, S6†). The results allowed us to propose that DCS::CURS catalyzing the formation of curcumin (1a) most likely involved in the formation and release of an intermediate diketide-CoA. At the initial stage, the low concentration of diketide-CoA in the reaction mixture result in the formation of curcumin (1a) in low velocity, with the concentration of diketide-CoA rising up, the velocity of curcumin (1a) formation increased. However, long time incubation would cause degradation of curcumin (1a). Notably, when a 3
:
1 mixture of feruloyl-CoA and malonyl-CoA was incubated with DCS::CURS, the reaction exclusively produced a single product of curcumin (1a). Presumably, the excess feruloyl-CoA completely exhausts the intermediate diketide-CoA to avoid the formation of the by-product 3-methoxy-4-hydroxy-3-methoxy-4-hydroxybenzalacetone (1b). In order to further probe the catalytic potentials of the fusion protein, a mixture of feruloyl-CoA and p-coumaroyl-CoA was incubated with malonyl-CoA and the fusion protein. An asymmetric diarylheptanoid of p-coumaroyl-feruloylmethane was unexpectedly produced as major product, excepting the formation of curcumin and a minor product bidemethoxycurcumin (2a) (HPLC chromatograms could be found in ESI, S7A†). Likewise, various asymmetric curcuminoids such as p-coumaroyl-cinnamoylmethane, feruloyl-cinnamoylmethane could also be formed by DCS::CURS (HPLC chromatograms could be found in ESI, S7B and S7C†). Notably, DCS::CURS catalyzed the formation of asymmetric curcuminoids such as 3-(4-hydroxyphenyl)propionyl-cinnamoylmethane, 3-(4-hydroxyphenyl)propionyl-feruloylmethane, and 3-(4-hydroxyphenyl)propionyl-p-coumaroylmethane would be useful to regioselectively synthesize this type of dihydrocurcuminoids (HPLC chromatograms could be found in ESI, S8†). In contrast, although CUS from O. sativa is preferred to be used for heterologous production of curcuminoids, wild CUS could not accept 3-(4-hydroxyphenyl)propionyl-CoA as a substrate preventing the smoothly utilization of CUS in heterologous production of dihydrocurcuminoids.10 The unnatural fusion protein DCS::CURS with broader substrate tolerance would thus be meaningful for synthesis of structurally more diverse curcuminoids.
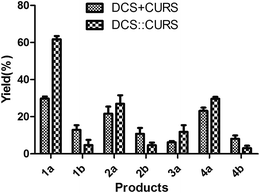 |
| Fig. 2 Increased production of curcuminoids (1a–4a) and decreased formation of benzalacetones/benzylacetone (1b, 2b, and 4b) by DCS::CURS. Results are presented as mean ± S.E. (n = 3). | |
In summary, we identified a new curcumin synthase (ZoCURS) from ginger and constructed a curcuminoid-producing unnatural fusion protein DCS::CURS. These would be not only contributable to further understand the biosynthesis of curcuminoids in ginger but also be advantage to further manipulate the biosynthesis of curcuminoid analogs, particularly including THBDC and various dihydrocurcuminoid derivatives in microorganisms.
Acknowledgements
We are thankful to the financial supports by Beijing Natural Science Foundation (No. 5132022) and the Program for New Century Excellent Talents in University (No. NCET-11-0604) and graduate independent project in Beijing University of Chinese Medicine (No. 2015-JYB-XS060).
References
- R. A. Sharma, A. J. Gescher and W. P. Steward, Eur. J. Cancer, 2005, 41, 1955 CrossRef CAS PubMed.
- R. F. Tayyem, D. D. Heath, W. K. Al-Delaimy and C. L. Rock, Nutr. Cancer, 2013, 55, 126 CrossRef PubMed.
- P. J. Roughley and D. A. Whiting, J. Chem. Soc., Perkin Trans. 1, 1973, 1, 2379 RSC.
- P. Denniff, l. Macleod and D. A. Whiting, J. Chem. Soc., Perkin Trans. 1, 1980, 1, 2637 RSC.
- P. Denniff and D. A. Whiting, J. Chem. Soc., Chem. Commun., 1976, 18, 711 RSC.
- M. d. C. Ramirez-Ahumada, B. N. Timmermann and D. R. Gang, Phytochemistry, 2006, 67, 2017 CrossRef CAS PubMed.
- Y. Katsuyama, T. Kita, N. Funa and S. Horinouchi, J. Biol. Chem., 2009, 284, 11160 CrossRef CAS PubMed.
- Y. Katsuyama, K. Miyazono, M. Tanokura, Y. Ohnishi and S. Horinouchi, J. Biol. Chem., 2011, 286, 6659 CrossRef CAS PubMed.
- Y. Katsuyama, M. Matsuzawa, N. Funa and S. Horinouchi, J. Biol. Chem., 2007, 282, 37702 CrossRef CAS PubMed.
- H. Morita, K. Wanibuchi, H. Nii, R. Kato, S. Sugio and I. Abe, Proc. Natl. Acad. Sci. U. S. A., 2010, 107, 19778 CrossRef CAS PubMed.
- I. Abe and H. Morita, Nat. Prod. Rep., 2010, 27, 809 RSC.
- W. Runguphan, X. Qu and S. E. O'Connor, Nature, 2010, 468, 461 CrossRef CAS.
- P. K. Ajikumar, W. H. Xiao, K. E. J. Tyo, Y. Wang, F. Simeon, E. Leonard, O. Mucha, T. H. Phon, B. Pfeifer and G. Stephanopoulos, Science, 2010, 330, 70 CrossRef CAS.
- J. D. Newman, J. Marshall, M. Chang, F. Nowroozi, E. Paradise, D. Pitera, K. L. Newman and J. D. Keasling, Biotechnol. Bioeng., 2006, 95, 684 CrossRef CAS PubMed.
- S. Galanie, K. Thodey, I. J. Trenchard, M. F. Interrante and C. D. Smolke, Science, 2015, 349, 1095 CrossRef CAS PubMed.
- Y. Li and P. C. Cirino, Biotechnol. Bioeng., 2014, 111, 1273 CrossRef CAS PubMed.
- Y. S. Zhang, S. Z. Li, J. Li, X. Q. Pan, R. E. Cahoon, J. G. Jaworski, X. M. Wang, J. M. Jez, F. Chen and O. Yu, J. Am. Chem. Soc., 2006, 128, 13030 CrossRef CAS PubMed.
- Y. Wang, H. Yi, M. Wang, O. Yu and J. M. Jez, J. Am. Chem. Soc., 2011, 133, 20684 CrossRef CAS PubMed.
- Y. Katsuyama, M. Matsuzawa, N. Funa and S. Horinouchi, Microbiology, 2008, 154, 2620 CrossRef CAS PubMed.
- Y. Katsuyama, Y. Hirose, N. Funa, Y. Ohnishi and S. Horinouchi, Biosci., Biotechnol., Biochem., 2010, 74, 641 CrossRef CAS PubMed.
- Y. Katsuyama, Y. Ohnishi and S. Horinouchi, ChemBioChem, 2010, 11, 2034 CrossRef CAS PubMed.
- J. L. Rodrigues, R. G. Araújo, K. L. Prather, L. D. Kluskens and L. R. Rodrigues, Biotechnol. J., 2015, 10, 599 CrossRef CAS PubMed.
- T. Mo, J. Wang, B. W. Gao, L. Zhang, X. Liu, X. H. Wang, P. F. Tu and S. P. Shi, Chin. J. Org. Chem., 2015, 35, 1052 CrossRef CAS.
- A. Nurfina, M. Reksohadiprodjo, H. Timmerman, U. Jenie, D. Sugiyanto and H. Van der Goot, Eur. J. Med. Chem., 1997, 32, 321 CrossRef CAS.
- W. M. Weber, L. A. Hunsaker, S. F. Abcouwer, L. M. Deck and D. L. Vander Jagt, Bioorg. Med. Chem., 2005, 13, 3811 CrossRef CAS PubMed.
- Y. Katsuyama, T. Kita and S. Horinouchi, FEBS Lett., 2009, 583, 2799 CrossRef CAS PubMed.
- E. K. Radhakrishnan, K. C. Sivakumar and E. V. Soniya, J. Proteomics Bioinf., 2009, 2, 310 CrossRef CAS.
- H. J. Koo, E. T. McDowell, X. Q. Ma, K. A. Greer, J. Kapteyn, Z. Z. Xie, A. Descour, H. Kim, Y. Yu, D. Kudrna, R. A. Wing, C. A. Soderlund and D. R. Gang, BMC Plant Biol., 2013, 13, 27 CrossRef CAS PubMed.
- S. Pedapudi, C. K. Chin and H. Pedersen, Biotechnol. Prog., 2000, 16, 346 CrossRef CAS PubMed.
- T. Abe, H. Morita, H. Noma, T. Kohno, H. Noguchi and I. Abe, Bioorg. Med. Chem. Lett., 2007, 17, 3161 CrossRef CAS.
- I. Abe, Y. Sano, Y. Takahashi and H. Noguchi, J. Biol. Chem., 2003, 278, 25218 CrossRef CAS PubMed.
- H. Morita, Y. Shimokawa, M. Tanio, R. Kato, H. Noguchi, S. Sugio, T. Kohno and I. Abe, Proc. Natl. Acad. Sci. U. S. A., 2010, 107, 669 CrossRef CAS PubMed.
Footnote |
† Electronic supplementary information (ESI) available. See DOI: 10.1039/c5ra23401h |
|
This journal is © The Royal Society of Chemistry 2016 |