DOI:
10.1039/C5RA22402K
(Paper)
RSC Adv., 2016,
6, 7999-8005
Synthesis and properties of pteridine-2,4-dione-functionalised oligothiophenes†
Received
26th October 2015
, Accepted 5th January 2016
First published on 19th January 2016
Abstract
The synthesis of acceptor-functionalised oligothiophene derivatives (t3, t6, t7, t8) is described, where the pteridine-2,4-dione acceptor units are arranged symmetrically in derivatives t6–t8. The symmetrical arrangement of acceptor units stems from the ability to selectively brominate the conjugated thiophene moiety of building block t3. Upon increasing the conjugation by going from t3 to t8, a significant increase in absorption toward the near-infrared region and a simultaneous narrowing of the HOMO/LUMO gap occurs, which may promote their future application as optoelectronic materials.
Introduction
Acceptor-functionalised short-chain linear oligothiophenes1 have received considerable attention over recent years, and have been utilised as components in organic photovoltaic (OPV) cells, organic field effect transistors (OFETs), and organic light emitting diodes (OLEDs).2 The juxtaposition of the thiophene donor (D) units with the acceptor (A) component affords conjugated D–A systems which typically promotes better charge carrier mobility and decreases the band gap.3 Therefore, the synthesis of new acceptor-appended short oligothiophenes are attractive targets for the development of promising new materials with a range of optoelectronic applications.
The pteridine-2,4-dione moiety occurs widely in biological systems, and in particular, is found in flavin-based redox cofactors.4 The fascinating redox properties of the latter have ensured that synthetic flavin derivatives have become important building blocks for systems with molecular device and optoelectronics applications.5 Here, we report a class of functionalised oligothiphene featuring pteridine-2,4-dione acceptor units which are constructed from building block t3 (Scheme 1). An important feature of t3 is that it is possible to selectively brominate the α-position of one of the thiophene units thereby allowing the synthesis of oligothiophenes t6–t8 with symmetrically appended acceptor units.
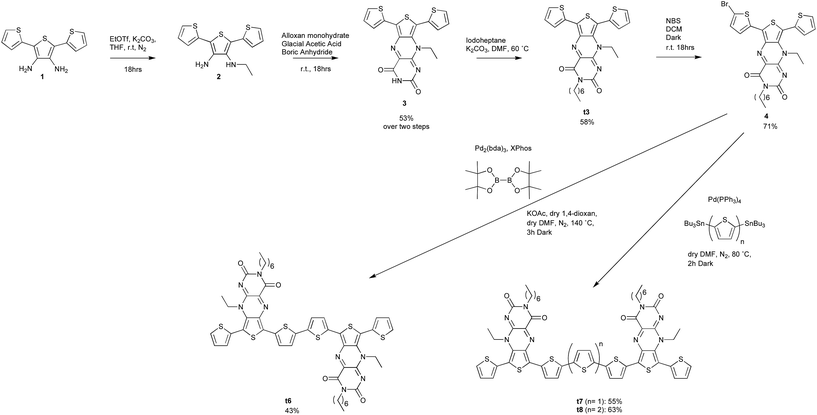 |
| Scheme 1 Synthesis of t3, t6–t8. | |
Results and discussion
Synthesis
The synthesis of the acceptor-functionalised oligothiophene derivatives are shown in Scheme 1. The synthesis of the key building block t3 was achieved from compound 1.6 N-Alkylation of 3 with iodoheptane was then undertaken to confer good solubility on t3 and its resulting oligomers. The X-ray crystal structure of the t3 clearly shows that one of the thiophene residues is almost coplanar to the pteridine core, whereas the other thiophene is non-planar and therefore non-conjugated to the core acceptor moiety (Fig. 1a). Bromination of t3 furnished compound 4 in 71% yield following purification by column chromatography. 1H NMR spectroscopy performed on compound 4 indicated that the bromination was selective, as evidence of other brominated species was not observed (see ESI†). X-ray crystallography confirmed that bromination occurs at the α-position of the more conjugated thiophene moiety (Fig. 1b). Compound 4 was then readily self-coupled (via the in situ preparation of its boronate ester) using a one-pot Suzuki–Miyaura reaction.7 Compound 4 could also be readily converted to oligothiophenes t7 and t8 using Stille methodology and the corresponding bis(tributylstannyl)thiophene.
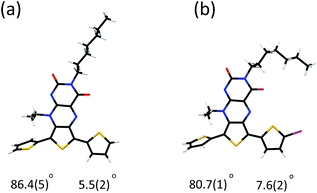 |
| Fig. 1 X-ray crystal structures of derivatives (a) t3 and (b) 4. Colour scheme: C, black; H, grey; Br, pink; N, blue; O, red; S, yellow. Torsion angles with respect to central thiophene ring are provided. | |
Photophysical and electrochemical properties
UV-vis spectroscopy performed on compound t3 indicated that the longest wavelength absorption band shows solvatochromism, which is consistent with an intramolecular charge-transfer (ICT) band (see ESI†). Increasing the oligothiophene chain length from t3 to t8 shifts the ICT absorption towards longer wavelength (547 nm for t3 to 673 nm for t8 in DCM) (Fig. 2). This chain length dependency in absorption properties suggests that the thiophene units are conjugated in the higher oligomers. The electrochemical properties of t3–t8 were determined using cyclic and square wave voltammetry (ESI† and Fig. 3). The electrochemical and optical properties are summarised in Table 1. The electrochemical data indicate that the oxidation potentials vary significantly whilst the reduction potentials remain fairly constant for the series. Accordingly, the calculated Efund (ref. 8) on going from t3 to t8 significantly decreases, which mainly results from a lowering of the ionization potentials (IPs) due to a concomitant increase in conjugation within the thiophene backbone. However, the electron affinities (EAs) remain relatively unchanged, indicating that increasing the length of thiophene backbone has a limited influence on the acceptor properties of the pteridine units.
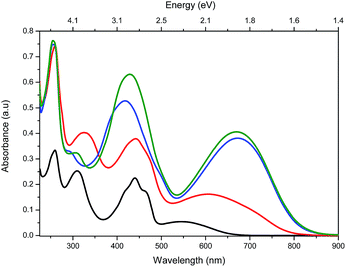 |
| Fig. 2 UV-vis absorption spectra of t3 (black), t6 (red), t7 (blue) and t8 (green) (1 × 10−5 M in DCM). | |
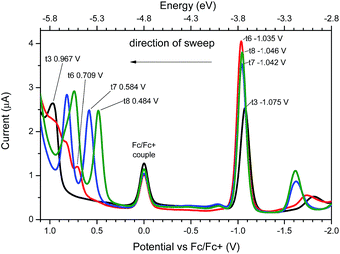 |
| Fig. 3 Square wave voltammetry of t3 (black), t6 (red), t7 (blue) and t8 (green) (1 × 10−5 M in DCM). | |
Table 1 Summary of electrochemical and optical properties of t3, t6, t8, t9. Efund (fundamental gap) = IP – EA. Eopt (optical gap) = 1240/λonset
|
Electrochemical properties |
Optical properties |
E(ox) (V) |
E(red) (V) |
IP (eV) |
EA (eV) |
Efund (eV) |
λmax (nm) |
λonset (nm) |
Eopt (eV) |
t3 |
0.97 |
−1.08 |
−5.8 |
−3.7 |
2.0 |
547 |
652 |
1.9 |
t6 |
0.71 |
−1.03 |
−5.5 |
−3.8 |
1.7 |
606 |
789 |
1.6 |
t7 |
0.58 |
−1.04 |
−5.4 |
−3.8 |
1.6 |
671 |
820 |
1.5 |
t8 |
0.48 |
−1.05 |
−5.3 |
−3.8 |
1.5 |
673 |
820 |
1.5 |
DFT calculations
DFT calculations were undertaken to probe the structure and electronic properties of t3–t8. The calculations for the oligomers are in accordance with the X-ray data for t3, and show that the outer thiophene units are out of conjugation with the acceptor core, whereas the conjugated thiophene units are essentially coplanar. The HOMOs of t3–t8 are mainly located on the conjugated thiophene backbone whereas the LUMOs are largely localised over the pteridine acceptor cores and their conjugated thiophene units (Fig. 4 and ESI†). The calculated HOMO/LUMO energy trends are broadly in accordance with the electrochemically determined IP and EA energies (t3 HOMO −5.8 eV, LUMO −3.1 eV; t6 HOMO −5.4 eV, LUMO −3.3 eV; t7 HOMO −5.2 eV, LUMO −3.3 eV; t8 HOMO −5.2 eV, LUMO −3.2 eV), and further indicate that on going from t3 to t8 it is the HOMO/IP that is most affected (ΔHOMO = 0.6 eV/ΔLUMO = 0.2 eV).
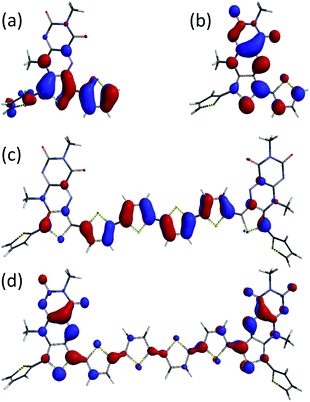 |
| Fig. 4 DFT-calculated structures of t3 and t8 (alkyl groups replaced with methyl units) showing the HOMO ((a) and (c)) and LUMO ((b) and (d)) maps. | |
Spectroelectrochemistry
Spectroelectrochemical measurements were carried out on compounds t3 (Fig. 5) and t8 (Fig. 6). Application of increasing negative potential to the working electrode produced a profound change in their UV-vis spectra, and the applied potentials of which generally coincide with the onset of the reduction waves shown in Fig. 3. The UV-vis spectra of compound t3 undergoes a significant change around −0.8 V with the peak at 439 nm collapsing and a new absorption at 471 nm forming. In accordance with spectroelectrochemical data recorded for related flavin derivatives, we attribute this spectral change due to the formation of pteridine radical anion species.9 As further negative potential is applied to the cell up to −1.2 V, the spectra pass through an isosbestic point at around 420 nm, whilst the absorption at 471 nm collapses and a shoulder at around 387 nm forms. This second feature is presumably due to the onset of the second reduced state (as indicated in the square wave voltammetry data). Interestingly, although the spectroelectrochemistry of t8 is qualitatively similar to that of t3 when a voltage of around −0.8 V is applied, in that a longer wavelength absorption at 445 nm and a shoulder around 480 nm form, however, a significantly longer wavelength absorption at 667 nm also forms for t8, which collapses with increasing negative potential. This new feature is likely a consequence of the addition of the second pteridine unit and the increased conjugation that occurs upon going from t3 to t8, which significantly reduces the onset voltage required to generate the second reduced state. For both compounds the original spectra recover upon returning the applied potential to 0 V.
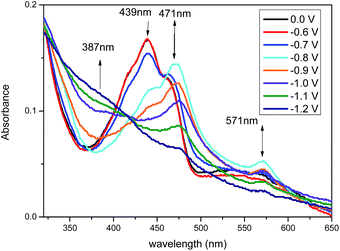 |
| Fig. 5 Spectroelectrochemistry of t3 recorded in DCM (1 × 10−4 M) using a platinum gauze working electrode, platinum wire counter electrode, silver wire pseudo-reference electrode and TBAPF6 as electrolyte (0.1 M). | |
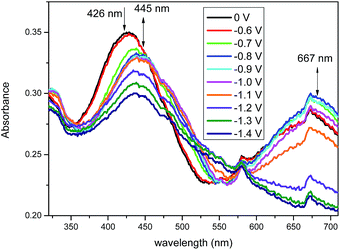 |
| Fig. 6 Spectroelectrochemistry of t8 recorded in DCM (1 × 10−4 M) using a platinum gauze working electrode, platinum wire counter electrode, silver wire pseudo-reference electrode and TBAPF6 as electrolyte (0.1 M). | |
Fluorescence
The solution fluorescence of t3 to t8 were investigated, and experiments revealed that these materials were only weakly fluorescent, presumably a consequence of their donor–acceptor architecture. We next investigated the ability of t3 and t8 to quench the fluorescence of the electron rich polymer poly(3-hexylthiophene-2,5-diyl) (P3HT). Fig. 7 shows the photoluminescent quantum yield (PLQY) of thin film blends of these materials prepared from chlorobenzene solutions. Increasing the concentration of t3 and t8 to as little as 1% causes a significant (50%) quenching of the fluorescence of P3HT, thereby indicating efficient electron transfer from the donor polymer to acceptors t3 and t8.10 At higher concentration of t3 and t8 (3%), no further quenching was observed indicating that significant aggregation of the t3 and t8 acceptor units may occur.
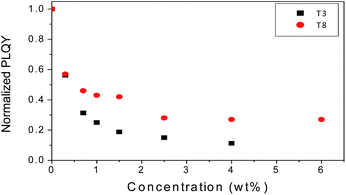 |
| Fig. 7 The PLQY of P3HT as a function of increasing concentrations of t3 and t8. | |
Experimental
All chemicals were purchased from commercial sources and used without further purification. (Sigma-Aldrich, TCI, Fisher, Acros). TLC was performed using aluminium sheets coated with silica gel 60 F254 (Merck). Dry solvents were obtained using Innovative Technology inc. Pure Solv 400-5-MD solvent purification system (activated alumina columns). IR spectra were recorded using a Perkin Elmer FT-IR instrument, νmax is given in wavenumbers (cm−1). Mass spectrometry was performed using a JEOL M STATION spectrometer. Melting points were measured using a SMP-10 Stuart Scientific melting point apparatus and are uncorrected. NMR spectroscopy was performed on either Bruker Avance 500 or a Bruker Avance 400 spectrometer. Spectra were processed using Mestrelab research iNMR© package (for MacOS X®). UV-vis and electrochemical data was processed using OriginLab©; Origin v. 8.5. Compound 1 was synthesised from 2,5-dibromothiophene.6
Synthesis
Compound 3. Compound 1 (0.8 g, 2.9 mmol) and K2CO3 (0.48 g, 3.5 mmol) were dissolved in dry THF (5 mL) under N2 atmosphere. Ethyl trifluoromethanesulfonate (0.39 mL, 3.0 mmol) was then added slowly and the reaction was left to stir overnight under N2. Water (100 mL) was added to the reaction mixture and the crude product was extracted into DCM (2 × 20 mL) and the organic extracts were dried over MgSO4. The drying agent was then removed by filtration and the solvents were removed under vacuum to yield terthiophene 2 as a dark brown solid. This was used without further purification and dissolved in glacial acetic acid (50 mL) with boric anhydride (0.47 g, 6.8 mmol) and alloxan monohydrate (0.56 g, 3.5 mmol). The reaction mixture was left to stir at room temperature overnight and the solvents were then removed under reduced pressure. The crude product was then purified by flash column chromatography (THF
:
hexane; 1
:
3) to yield 3 a red-greenish solid (0.63 g, 53%); mp > 300 °C; νmax/cm−1 (solid state) 3159 (N–H), 1673 (C
O); δH (500 MHz, CDCl3) 1.22 (3H, t, J 7.0, C
3), 4.43 (2H, q, J 7.0, C
2), 7.17–7.20 (2H, m, C
), 7.34 (1H, dd, J 3.5, 1.2, C
), 7.60 (1H, dd, J 5.3, 1.2, C
), 7.63 (1H, dd, J 5.1, 1.0, C
), 7.83 (1H, dd, J 3.8, 1.0, C
), 8.48 (1H, s, N
); δC (125 MHz, CDCl3) 12.7 (
H3), 41.4 (
H2), 106.1 (
), 107.4 (
), 127.4 (
), 127.5 (
H), 128.17 (
H), 129.0 (
H), 129.8 (
H), 130.6 (
), 131.6 (
H), 132.1 (
), 132.6 (
H), 133.9 (
), 135.1 (
), 142.3 (![[C with combining low line]](https://www.rsc.org/images/entities/char_0043_0332.gif)
O), 150.3 (![[C with combining low line]](https://www.rsc.org/images/entities/char_0043_0332.gif)
O); m/z (FAB+) 413.0202 [M + H]+ (C18H13N4O2S3 requires 413.0201).
Compound t3. Iodoheptane (0.16 mL, 1.0 mmol) was added to a solution of compound 3 (0.15 g, 0.36 mmol) and potassium carbonate (0.19 g, 1.4 mmol) in acetone (20 mL). The reaction was stirred under reflux overnight. The solvent was then evaporated and the reaction quenched with water (20 mL). The crude product was extracted with chloroform (3 × 15 mL), washed with water (2 × 25 mL) and brine (2 × 25 mL). The combined organic extracts were then dried over MgSO4, filtered and the solvent was removed under reduced pressure. The crude product was then purified by flash column chromatography (hexane/ethyl acetate; 3
:
1) to yield compound t3 as a red solid (106 mg, 58%); mp 189–191 °C; νmax/cm−1 (solid state) 2951–2855, 1659, 1585, 1558, 1524, 1435; δH (500 MHz, CDCl3) 0.87 (3H, t, J 6.8, C
3), 1.18 (3H, t, J 7.0, C
3), 1.25–1.42 (8H, m, C
2), 1.72 (2H, dt, J 14.9, 7.5, C
2), 4.06 (2H, t, J 7.5, C
2), 4.37 (2H, q, J 7.0, C
2), 7.14 (2H, ddd, J 5.1, 3.7, 1.1, C
), 7.31 (1H, dd, J 3.7, 1.1, C
), 7.56 (2H, ddd, J 5.1, 3.7, 1.1, C
), 7.78 (1H, dd, J 3.7, 1.1, C
); δC (125 MHz, CDCl3) 12.9 (
H3), 14.2 (
H3), 22.7 (
H2), 27.1 (
H2), 28.0 (
H2), 29.2 (
H2), 31.9 (
H2), 40.9 (
H2), 42.3 (
H2), 107.0 (
), 127.5 (
H), 127.6 (
), 128.1 (
H), 128.8 (
H), 129.7 (
H), 131.0 (
), 131.3 (
H), 132.4 (
), 132.6 (
H), 133.8 (
), 135.2 (
), 141.6 (
), 148.9 (
), 155.9 (![[C with combining low line]](https://www.rsc.org/images/entities/char_0043_0332.gif)
O), 159.6 (![[C with combining low line]](https://www.rsc.org/images/entities/char_0043_0332.gif)
O); m/z (FAB +) 511.1295 [M + H]+ (C25H27N4O2S3 requires 511.1296).
Compound 4. Compound t3 (170 mg, 0.32 mmol) was dissolved in DCM (10 mL) and the flask was covered with aluminium foil. N-Bromosuccinamide (64 mg, 0.36 mmol) was then added and the reaction mixture was left to stir overnight. The reaction was then quenched with water (100 mL) and the crude product extracted into DCM (3 × 50 mL). The combined organic extracts were then dried over MgSO4, filtered and the solvent was removed under reduced pressure. The crude product was then purified by flash column chromatography (DCM 100%) to yield compound 4 as a green solid (158 mg, 81%); mp 242–244 °C; δH (500 MHz, CDCl3) 0.88 (3H, t, J 6.9, C
3), 1.18 (3H, t, J 7.0, C
3), 1.26–1.43 (8H, m, C
2), 1.69–1.75 (2H, m, C
2), 4.06 (2H, t, J 7.7, C
2), 4.37 (2H, q, J 7.0, C
2), 7.10 (1H, d, J 4.0, C
), 7.15 (1H, dd, J 5.3, 3.5, C
), 7.31 (1H, dd, J 3.5, 1.2, C
), 7.43 (1H, d, J 4.0, C
), 7.57 (1H, dd, J 5.3, 1.2, C
); δC (125 MHz, CDCl3) 12.9 (
H3), 14.3 (
H3), 22.8 (
H2), 27.2 (
H2), 28.0 (
H2), 29.2 (
H2), 31.9 (
H2), 40.9 (
H2), 42.4 (
H2), 107.3 (
), 120.3 (
Br), 127.5 (
), 127.6 (
H), 128.2 (
H), 129.9 (
H), 130.6 (
H), 130.8 (
), 132.7 (
H), 133.8 (
), 133.9 (
), 135.3 (
), 140.4 (
), 149.0 (
) 155.8 (![[C with combining low line]](https://www.rsc.org/images/entities/char_0043_0332.gif)
O), 159.5 (![[C with combining low line]](https://www.rsc.org/images/entities/char_0043_0332.gif)
O); m/z (ES +) 611.0196 [M + Na]+ (C25H2579BrN4NaO2S3 requires 611.0215).
Compound t6. Compound 4 (120 mg, 0.2 mmol), tris(dibenzylideneacetone) dipalladium(0) (3.7 mg, 4.0 μmol), bis-(pinacolato)diboron (105 mg, 0.4 mmol), K2OAc (40 mg, 0.4 mmol) and X-phos (40 mg, 8.0 μmol) were added to a Schlenk tube, which was then evacuated and backfilled with N2 gas three times. A mixture of dry 1,4-dioxane (15 mL) and dry DMF (5 mL) was added under N2 and the tube was then sealed, protected from light and placed in an oil bath at 140 °C for 3 h. DCM (100 mL) was added and the organic layer was washed with water. The organic layer was dried over MgSO4, filtered and the solvent evaporated under vacuum. The product was purified by column chromatography (100% DCM) to yield compound t6 as a green solid (45 mg, 43%); mp 295–297 °C; νmax/cm−1 (film) 2929, 2857, 1705, 1655, 1585, 1559, 1523, 1475; δH (500 MHz, CDCl3) 0.86 (6H, t, J 6.9, C
3), 1.16 (6H, t, J 6.9, C
3), 1.41–1.21 (16H, m, C
2), 1.60–1.67 (4H, m, C
2), 4.03 (4H, t, J 8.0, C
2), 4.35 (4H, q, J 6.9, C
2), 7.13 (2H, dd, J 5.3, 3.6, C
), 7.27 (2H, d, J 4.0, C
), 7.28 (2H, dd, J 3.6, 1.2, C
), 7.55 (2H, dd, J 5.3, 1.2, C
), 7.64 (2H, d, J 4.0, C
); δC (125 MHz, CDCl3) 12.9 (
H3), 14.2 (
H3), 22.8 (
H2), 27.2 (
H2), 28.0 (
H2), 29.2 (
H2), 31.9 (
H2), 40.9 (
H2), 42.4 (
H2), 107.8 (
), 127.6 (
H), 127.6 (
), 129.0 (
H), 129.9 (
H), 130.8 (
), 132.6 (
H), 133.6 (
H), 134.1 (
), 135.5 (
), 135.9 (
), 140.3 (
), 140.9 (![[C with combining low line]](https://www.rsc.org/images/entities/char_0043_0332.gif)
N), 148.9 (![[C with combining low line]](https://www.rsc.org/images/entities/char_0043_0332.gif)
N), 155.8 (![[C with combining low line]](https://www.rsc.org/images/entities/char_0043_0332.gif)
O), 159.5 (![[C with combining low line]](https://www.rsc.org/images/entities/char_0043_0332.gif)
O); m/z (EI+) 1041.2123 [M + Na]+ (C50H50N8O4S6Na requires 1041.2171).
Compound t7. Compound 4 (110 mg, 0.2 mmol), 2,5-bis-(tributylstannyl)thiophene (55 μL, 0.1 mmol) and tetrakis(triphenylphosphine)palladium(0) (6 mg, 5.0 μmol) were added, under N2 gas, to dry DMF (2 mL). The reaction was protected from light and stirred at 80 °C for 2 h. The reaction was terminated by diluting with DCM (75 mL) and then washed with water (3 × 75 mL), dried over MgSO4 and filtered. The solvent was evaporated under vacuum and the product was purified by column chromatography (DCM 100%) to yield compound t7 as a green solid (60 mg, 55%); mp > 300 °C; νmax/cm−1 (film) 2929, 2857, 1704, 1653, 1584, 1557, 1510; δH (500 MHz, CDCl3) 0.85 (6H, t, J 6.8, C
3), 1.14 (6H, t, J 7.0, C
3), 1.42–1.19 (16H, m, C
2), 1.70–1.76 (4H, m, C
2), 4.03 (4H, t, J 8.0, C
2), 4.33 (4H, q, J 7.0, C
2), 7.13 (2H, dd, J 5.0, 3.5, C
), 7.16 (2H, d, J 4.0, C
), 7.23 (2H, s, C
), 7.30 (2H, dd, J 3.4, 0.9, C
), 7.54 (2H, dd, J 5.3, 0.9, C
), 7.66 (2H, d, J 4.0, C
); δC (125 MHz, CDCl3) 12.91 (
H3), 14.25 (
H3), 22.76 (
H2), 27.18 (
H2), 28.07 (
H2), 29.23 (
H2), 31.93 (
H2), 40.87 (
H2), 42.34 (
H2), 106.88 (
), 124.85 (
H), 126.32 (
H), 127.57 (
H), 127.61 (
), 129.81 (
H), 129.89 (
H), 130.94 (
), 131.55 (
), 132.66 (
H), 133.32 (
), 135.49 (
), 136.81 (
), 140.99 (
), 142.77 (![[C with combining low line]](https://www.rsc.org/images/entities/char_0043_0332.gif)
N), 148.97 (![[C with combining low line]](https://www.rsc.org/images/entities/char_0043_0332.gif)
N), 155.87 (![[C with combining low line]](https://www.rsc.org/images/entities/char_0043_0332.gif)
O), 159.60 (![[C with combining low line]](https://www.rsc.org/images/entities/char_0043_0332.gif)
O); m/z (EI+) 1123.1996 [M + Na]+ (C54H52N8O4S7Na requires 1123.2049).
Compound t8. Compound 4 (210 mg, 0.41 mmol), 5,5′-bis-(tributylstannyl)-2,2′-bithiophene (0.12 mL, 0.2 mmol) and tetrakis(triphenylphosphine) palladium(0) (12 mg, 0.01 mmol) were added, under N2 gas, to dry DMF (4 mL). The reaction was protected from light and stirred at 80 °C for 2 h. DCM (100 mL) was added and the organic layer was washed with distilled water (3 × 100 mL), dried over MgSO4 and filtered. The solvent was evaporated under vacuum and the product purified by column chromatography (DCM 100%) to yield compound t8 as a green solid (150 mg, 63%); mp > 300 °C); νmax/cm−1 (film) 2923, 2853, 1704, 1651, 1582, 1556, 1530, 1509; δH (500 MHz, C2D2Cl4) 0.91 (6H, t, J 6.8, C
3), 1.19 (6H, t, J 7.0, C
3), 1.46–1.24 (16H, m, C
2), 1.67–1.71 (4H, m, C
2), 4.00–4.03 (4H, m, C
2), 4.34 (4H, q, J 6.8, C
2), 7.17–7.19 (4H, m, C
), 7.22 (2H, d, J 3.9, C
), 7.30 (2H, d, J 4.0, C
), 7.34 (2H, dd, J 3.4, 1.0, C
), 7.61 (2H, dd, J 5.3, 1.0, C
), 7.73 (2H, d, J 4.0, C
); δC (125 MHz, C2D2Cl4) 12.6 (
H3), 14.1 (
H3), 22.6 (
H2), 26.9 (
H2), 27.9 (
H2), 29.0 (
H2), 31.7 (
H2), 40.8 (
H2), 41.9 (
H2), 106.9 (
), 124.7 (
H), 125.1 (
H), 126.1 (
H), 127.2 (
), 127.4 (
H), 129.7 (
H), 130.0 (
H), 130.3 (
), 131.0 (
), 132.5 (
H), 133.0 (
), 135.2 (
), 135.5 (
), 137.0 (
), 140.7 (
), 142.4 (![[C with combining low line]](https://www.rsc.org/images/entities/char_0043_0332.gif)
N), 148.6 (![[C with combining low line]](https://www.rsc.org/images/entities/char_0043_0332.gif)
N), 155.3 (![[C with combining low line]](https://www.rsc.org/images/entities/char_0043_0332.gif)
O), 159.4 (![[C with combining low line]](https://www.rsc.org/images/entities/char_0043_0332.gif)
O); m/z (EI+) 1205.1895 [M + Na]+ (C58H54N8O4S8Na requires 1205.1931).
Spectroscopic and voltammetry measurements
UV-vis spectra were recorded on a Shimadzu UV-3600 spectrometer using a 10 mm path length quartz cuvette. Cyclic voltammetry and square wave voltammetry was recorded with a CH Instruments Inc. 440A potentiostat. The voltammograms were recorded in solutions of tetrabutylammonium hexafluorophosphate (TBAPF6) in dry-DCM (0.1 M). Measurements were performed with degassed solutions under inert atmosphere, using a platinum working electrode, a silver wire pseudo-reference electrode and a platinum wire counter electrode. The redox potentials were determined relative to the ferrocene/ferrocenium redox couple (4.8 eV), and IPs and EAs were estimated from these values.
UV-vis spectroelectrochemistry
Spectroelectrochemical measurements were carried out using the ALS Co. Ltd. SEC-C 0.5 mm spectroelectrochemical cell featuring a platinum gauze working electrode and silver wire pseudo-reference electrode and a platinum wire counter electrode. All experiments were carried out with 1 × 10−4 M solutions of the analyte in DCM with TBAPF6 (0.1 M) as the supporting electrolyte. UV-vis spectra were recorded on a Shimadzu UV-3600 spectrometer, and potential was applied using a CH Instruments Inc. 440A potentiostat. A base line spectrum of the cell and electrolyte was subtracted from the recorded spectra and the spectra were re-zeroed.
Fluoresence quenching experiments
A solution of P3HT (from Rieke metals) was prepared by dissolving 20 mg mL−1 in chlorobenzene. Solutions of t3 and t8 were prepared by dissolving 1 mg mL−1 in chlorobenzene. Both solutions were mixed by appropriate volumes to yield the desired ratio of P3HT and t3 and t8 with the concentration given as the fraction of total mass. The solutions were then spin coated in a nitrogen filled glovebox at 1000 rpm. Quenching studies were performed by measuring the photoluminescence quantum yield with a Hamamatsu U6039-05 integrating sphere. At the excitation wavelength of 500 nm the total absorption was more than 60% and the emission was collected in a range from 650 to 900 nm. PLQY was determined by the instrument and is given by the number of photons emitted divided by the number of photons absorbed.
DFT calculations
Calculations were performed using a Spartan '14 (64-bit) software suite.11 Molecular geometries were first optimised semi-empirically (AM1) and then re-optimised using DFT (B3LYP/6-31G*). Alkyl chains are substituted with methyl groups in order to diminish calculation time. The resulting structures were local minima, as none of the vibrational frequencies generated imaginary frequencies.
X-ray structure determination
Crystal data for t3: C26H28Cl2N4O2S3, Mr = 595.60, monoclinic system, space group P21/c, a = 14.3594(3), b = 22.1059(5), c = 8.8264(2) Å, β = 104.710(2), V = 2709.91(10) Å3. Z = 4, ρcalcd = 1.460 g cm−3; MoKα radiation, λ = 0.71073 Å, μ = 0.504 mm−1, T = 150 K. 21011 data (5140 unique, Rint = 0.0421, θ < 25.7°) were collected on an Oxford Gemini A Ultra CCD diffractometer and were corrected for absorption (transmission 0.851–0.880). The structure was solved by direct methods and refined by full-matrix least-squares on F2 to give wR2 = 0.1775, R1 = 0.0576, S = 1.038 for 362 parameters. Residual electron density extrema were 0.72 and −0.86 e Å−3.
Crystal data for 4: C25H25BrN4O2S3, Mr = 589.58, monoclinic system, space group P21/c, a = 14.7336(8), b = 18.2084(11), c = 9.4057(6) Å, β = 94.715(3), V = 2514.8(3) Å3. Z = 4, ρcalcd = 1.557 g cm−3; MoKα radiation, λ = 0.71073 Å, μ = 1.915 mm−1, T = 150 K. 19655 data (4922 unique, Rint = 0.0406, θ < 26°) were collected on a Bruker Apex II Quasar CCD diffractometer and were corrected for absorption (transmission 0.846–0.945). The structure was solved by direct methods and refined by full-matrix least-squares on F2 to give wR2 = 0.1037, R1 = 0.0396, S = 1.051 for 318 parameters. Residual electron density extrema were 0.96 and −0.56 e Å−3.
The crystallographic data for compound t3 and 4 have been deposited with the Cambridge Crystallographic Data Centre with deposition number CCDC 1053866-1053867.
Conclusions
In conclusion, we report the synthesis of the symmetrically functionalised oligothiophene derivatives t6, t7 and t8 from building block t3. The increased conjugation that occurs on going from t3 to t8 results in a significant bathochromic shift in the maximum wavelength absorption and an Efund as low as 1.5 eV. PLQY experiments reveal that these units have the propensity to act as efficient electron acceptors for the electron donor polymer P3HT.12 It is anticipated that these, and related derivatives, will have interesting optoelectronics applications (e.g. photovoltaic properties).13 Our investigations will be reported in due course.
Acknowledgements
GC and IDWS thank the EPSRC for funding (EP/I00243X/1). VR thanks the NSF (CHE-1307021). IDWS also acknowledges support from a Royal Society Wolfson Research Merit Award.
Notes and references
-
(a) L. Zhang, N. S. Colella, B. P. Cherniawski, S. C. B. Mannsfeld and A. L. Briseno, ACS Appl. Mater. Interfaces, 2014, 6, 5327–5343 CrossRef CAS PubMed;
(b) A. Mishra, C.-Q. Ma and P. Bäuerle, Chem. Rev., 2009, 109, 1141–1276 CrossRef CAS PubMed.
- For illustrative examples see:
(a) J. J. Chen, T. L. Chen, B. Kim, D. A. Poulsen, J. L. Mynar and J. M. Fréchet, ACS Appl. Mater. Interfaces, 2010, 2, 2679–2686 CrossRef CAS PubMed;
(b) Y. Liu, X. Wan, B. Yin, J. Zhou, G. Long, S. Yin and Y. Chen, J. Mater. Chem., 2010, 20, 2464–2468 RSC;
(c) G. C. Welch, L. A. Perez, C. V. Hoven, Y. Zhang, X.-D. Dang, A. Sharenko, M. F. Toney, E. J. Kramer, T.-Q. Nguyen and G. C. Bazan, J. Mater. Chem., 2011, 21, 12700–12709 RSC;
(d) Z. Li, G. He, X. Wan, Y. Liu, J. Zhou, G. Long, Y. Zuo, M. Zhang and Y. Chen, Adv. Energy Mater., 2012, 2, 74–77 CrossRef CAS;
(e) Y. Liu, X. Wan, F. Wang, J. Zhou, G. Long, J. Tian and Y. Chen, Adv. Mater., 2011, 23, 5387–5391 CrossRef CAS PubMed;
(f) J. Zhou, X. Wan, Y. Liu, G. Long, F. Wang, Z. Li, Y. Zuo, C. Li and Y. Chen, J. Mater. Chem., 2011, 23, 4666–4668 CrossRef CAS;
(g) Y. Liu, X. Wan, F. Wang, J. Zhou, G. Long, J. Tian, J. You, Y. Yang and Y. Chen, Adv. Energy Mater., 2011, 1, 771–775 CrossRef CAS;
(h) G. He, Z. Li, X. Wan, Y. Liu, J. Zhou, G. Long, M. Zhang and Y. Chen, J. Mater. Chem., 2012, 22, 9173–9180 RSC;
(i) G. He, Z. Li, X. Wan, J. Zhou, G. Long, S. Zhang, M. Zhang and Y. Chen, J. Mater. Chem., 2013, 1, 1801–1809 RSC;
(j) G. Long, X. Wan, B. Kan, Y. Liu, G. He, Z. Li, Y. Zhang, Y. Zhang, Q. Zhang, M. Zhang and Y. Chen, Adv. Energy Mater., 2013, 3, 639–646 CrossRef CAS;
(k) X. Wan, Y. Liu, F. Wang, J. Zhou, G. Long and Y. Chen, Org. Electron., 2013, 14, 1562–1569 CrossRef CAS;
(l) T. S. van der Poll, J. A. Love, T.-Q. Nguyen and G. C. Bazan, Adv. Mater., 2012, 24, 3646–3649 CrossRef CAS PubMed;
(m) J. Zhou, Y. Zuo, X. Wan, G. Long, Q. Zhang, W. Ni, Y. Liu, Z. Li, G. He, C. Li, B. Kan, M. Li and Y. Chen, J. Am. Chem. Soc., 2013, 135, 8484–8487 CrossRef CAS PubMed;
(n) J. Zhou, X. Wan, Y. Liu, Y. Zuo, Z. Li, G. He, G. Long, W. Ni, C. Li, X. Su and Y. Chen, J. Am. Chem. Soc., 2012, 134, 16345–16351 CrossRef CAS PubMed;
(o) F. Baert, C. Cabanetos, A. Leliège, E. Kirchner, O. Segut, O. Alévêque, M. Allain, G. Seo, S. Jung, D. Tondelier, B. Geffroy, J. Roncali, P. Lerichea and P. Blanchard, J. Mater. Chem. C, 2015, 3, 390–398 RSC;
(p) P. J. Skabara, R. Berridge, I. M. Serebryakov, A. L. Kanibolotsky, L. Kanibolotskaya, S. Gordeyev, I. F. Perepichka, N. S. Sariciftci and C. Winder, J. Mater. Chem., 2007, 17, 1055–1062 RSC;
(q) W. Zhang, S. C. Tse, J. Lu, Y. Tao and M. Shing Wong, J. Mater. Chem., 2010, 20, 2182–2189 RSC;
(r) A. Riaño, P. Mayorga Burrezo, M. J. Mancheño, A. Timalsina, J. Smith, A. Facchetti, T. J. Marks, J. T. López Navarrete, J. L. Segura, J. Casado and R. Ponce Ortiz, J. Mater. Chem. C, 2014, 2, 6376–6386 RSC;
(s) T. Noda and Y. Shirota, J. Am. Chem. Soc., 1998, 120, 9714–9715 CrossRef CAS.
- E. Ahmed, G. Ren, F. S. Kim, E. C. Hollenbeck and S. A. Jenekhe, Chem. Mater., 2011, 23, 4563–4577 CrossRef CAS.
- S. O. Mansoorabadi, C. J. Thibodeaux and H.-W. Liu, J. Org. Chem., 2007, 72, 6329–6342 CrossRef CAS PubMed.
-
(a) A. Niemz and V. M. Rotello, Acc. Chem. Res., 1999, 32, 44–52 CrossRef CAS;
(b) V. Nandwana, I. Samuel, G. Cooke and V. M. Rotello, Acc. Chem. Res., 2013, 46, 1000–1009 CrossRef CAS PubMed.
- X. Yangjun, J. Luo, X. Deng, X. Li, D. Li, X. Zhu, W. Yang and Y. Cao, Macromol. Chem. Phys., 2006, 207, 511–520 CrossRef.
- F. Brouwer, J. Alma, H. Valkenier, T. P. Voortman, J. Hillebrand, R. C. Chiechi and J. C. Hummelen, J. Mater. Chem., 2011, 21, 1582–1592 RSC.
- J.-L. Bredas, Mater. Horiz., 2014, 1, 17–19 RSC.
- A. Niemz, J. Imbriglio and V. M. Rotello, J. Am. Chem. Soc., 1997, 119, 887–892 CrossRef CAS.
- P. E. Schwenn, K. Gui, A. M. Nardes, K. B. Krueger, K. H. Lee, K. Mutkins, H. Rubinstein-Dunlop, P. E. Shaw, N. Kopidakis, P. L. Burn and P. Meredith, Adv. Energy Mater., 2011, 1, 73–81 CrossRef CAS.
- Wavefunction Inc., 18401 Von Karman Ave., Suite 370, Irvine, CA 92612, USA.
- A. F. Eftaiha, J.-P. Sun, I. G. Hill and G. C. Welch, J. Mater. Chem. A, 2014, 2, 1201–1213 CAS.
- L. Yuan, Y. Zhao, K. Lu, D. Deng, W. Yan and Z. Wei, J. Mater. Chem. C, 2014, 2, 5842–5849 RSC.
|
This journal is © The Royal Society of Chemistry 2016 |