DOI:
10.1039/C5RA21495E
(Paper)
RSC Adv., 2016,
6, 44-56
A new and facile strategy for the one-pot fabrication of luminescent gold nanoclusters and their prospective application†
Received
15th October 2015
, Accepted 8th December 2015
First published on 9th December 2015
Abstract
A simple, novel, green, economic, environment friendly and convenient one-pot fabrication of luminescent gold nanoclusters, incorporating an aqueous extract of the peels of Allium cepa L., otherwise considered as a common household waste material, has been described. The optimal experimental conditions including concentrations of Allium cepa L. extract and different reaction temperatures have been investigated in detail. The nanoclusters were characterized by various spectroscopic techniques, such as ultra-violet spectroscopy (UV-vis), photoluminescence spectroscopy (PL), Transmission Electron Microscopy (TEM), Selected Area Electron Diffraction pattern (SAED), Fourier-transform infrared spectra (FTIR), X-ray diffraction spectroscopy (XRD) and scanning electron microscopy with energy dispersive X-ray (SEM-EDX). spectroscopic results revealed the formation of clusters with an average diameter in the range of 4–9 nm and depicted a fluorescence emission spectrum at 640 nm. Furthermore, the EDX pattern also revealed the formation of gold nanoclusters. The prepared nanoclusters were effectively utilized for the remediation of two carcinogenic and lethal textile dyes, rose bengal and methylene blue from aqueous solution. Approximately, 98.9 and 95.1% of rose bengal and methylene blue dye were degraded within 180 and 145 min using the synthesized gold nanoclusters, respectively. Additionally, the exhausted nanoclusters were regenerated and their photo catalytic capability was evaluated for three continuous rounds of cycling. The exhausted nanoclusters and the intermediates of the degradation process were respectively analysed using TEM, SAED and LC-MS techniques. The catalytic efficiency of the fabricated nanoclusters was also evaluated by conversion of 4-nitro phenol to 4-amino phenol in the presence of sodium borohydride in an aqueous medium. It was observed that about 97.3% of 4-nitro phenol was reduced within 70 min. Furthermore, the fabricated clusters were also found to inhibit the growth of a common human pathogenic bacterium, Escherichia coli. Hence, the present study has unfastened a pioneering way for synthesizing Au NCs and their applicability for the remediation of hazardous compounds and their potential as antibacterial agents.
1. Introduction
During the last few decades, the fabrication of luminescent metal nanoclusters (NCs) especially gold nanoclusters (Au NCs) has continued to evolve as one of the thirstiest research areas in the field of nanoscience and nanotechnology owing to their innumerable advantages, fascinating properties and their ease of fabrication, water solublization, non-hazardous nature and more biocompatibility.1,2 Actually the term NCs is restricted to entities having a diameter from sub-nanometer to 10 nanometers.3,4
Until now, numerous fabrication techniques have been formulated for the synthesis of Au NCs, but despite their advances, all these techniques are not entirely acceptable owing to limitations such as requirement of complex reagents, longer reaction time, harsh reaction conditions and poor yield.5,6 Consequently, the development of a stable and functionalized luminescent Au NCs employing green, facile, rapid and environmental friendly method with high quantum yield and good biocompatibility is an urgent necessitate of the scientific fraternities.
Therefore, in this pursuit, we endeavored to exploit a common household waste material, outer peel extracts of Allium cepa L., considered as useless as well as an environmental threat for the rapid fabrication of Au NCs by controlling different reaction parameters such as temperature and concentration. It is perceived that the compositional abundance of flavonoids such as quercetin and amino acid cysteine and its derivatives such as S-substituted cysteine sulphoxides and γ-glutamyl peptides may be envisaged for the effective reduction and stabilization of the Au NCs.7 Moreover, now with the increase of urbanization, industrialization and modernization, the environmental toxicity in the form of air and water pollution has been enhanced and a major portion is constituted by various hazardous organic compounds such as dyes, nitro phenol and its derivatives.
Dyes find several applications in our daily life and are extensively used in various industries and are recognized as the primary contaminant in industrial waste water.8 Dyes are carcinogenic and toxic in nature and are a considerable source of non-aesthetic pollution and eutrophication that generate harmful byproducts by further oxidation, hydrolysis or other chemical reactions in the waste water.9 Apart from these, the presence of dyes in water can reduce the light penetration resulting in less photosynthetic activity, thus making oxygen unavailable for biodegradation of microorganisms in the water.10 Hence, it possesses a threat to both human begins and marine ecosystems.11 Therefore, their complete dislodgment is a mandatory and a cumbersome assignment owing to their complicated structure and high stability.
In this article, two dyes namely, Rose Bengal (RB) and Methylene Blue (MB) have been selected. RB is a xanthene class of dye and is extensively used as a dyeing material, insecticides, and in printing industries. The dissipation of RB causes several harmful diseases to the liver and stomach of human beings. While MB is water soluble heterocyclic aromatic chemical compound and is used as a colorant and as a pharmaceutical drug. It is lethal and causes dermatological, gastrointestinal, cardiovascular, genitor urinal and hematological problems. Consequently, RB and MB are health hazardous and are threat to all living organisms and their abatement is necessary and photocatalytic degradation in presence of suitable nanocatalyst is found to be the most effective method for their remediation.
Apart from dyes, nitro-phenol and its derivatives are also menace to environment. Para-nitro phenol (4-NP) is known to be toxic, anthropogenic and inhibitory in nature.12 4-NP is widely used in the production of various fungicides, pesticides, insecticides, pharmaceuticals and dyes. Their exposure causes headaches, nausea, drowsiness, cyanosis and damage to central nervous system, kidney, liver and both human and animal blood. Furthermore, 4-NP is highly soluble and stable in water. Hence, their complete dislodgement is mandatory on account of environmental concern. Scientific fraternities have developed various techniques for their remediation likewise microbial degradation, traditional water purification method, adsorption, photocatalytic degradation, microwave-assisted catalytic oxidation, electro coagulation, electro-Fenton method and electrochemical treatment.13 But all these methodologies employ organic solvents and are energy consuming. Hence, it is obligatory to develop a technique or reaction which can be easily studied or developed and is trustworthy, convincing and requires simple experimental set up. So, far only one reaction which stands on a strong footing and has been qualified is the catalytic reduction of 4-NP to 4-AP (para-aminophenol) in aqueous media in presence of sodium borohydride (NaBH4) using Au NCs as catalyst. Hence, we exploited the fabricated Au NCs for the remediation of hazardous organic compound, namely; RB, MB and 4-NP.
Additionally, the coinage NPs exhibited potential antimicrobial activities and find applications in various fields such as water treatment, medical devices, instruments, and food processing due to their smaller size, high surface area to volume ratio which allows stronger interaction with microbial membranes and is not due to the liberation of metal ions in solutions.14
Hence, increased environmental pollution has evoked our research attention to utilize materials or to design processes that are friendly to both environment and human health. Thus, in this perspective, the present work addresses a green, facile, environment friendly and cost-effective method for the fabrications of Au NCs employing a common household waste material (peels of Allium cepa L.) and their utility as a catalyst for the abatement of hazardous compounds (RB, MB and 4-NP). The antibacterial activities of the fabricated Au NCs were also explored. The synthesized NCs were characterized using various techniques, such as UV-visible spectroscopy (UV–vis spectroscopy), photoluminescence spectroscopy (PL spectroscopy), Fourier transformer infrared spectroscopy (FTIR spectroscopy), transmission electron spectroscopy (TEM), Selected Area Electron Diffraction (SAED) pattern and energy dispersive X-ray spectroscopy (EDAX).
2. Experimental
2.1 Materials
Hydrogen tetrachloroaurate(III) trihydrate (HAuCl4·3H2O), Rose Bengal (RB), Methylene Blue (MB), para-nitrophenol (4-NP), sodium borohydride (NaBH4) of AR grade was procured from Sigma-Aldrich and used as received. Double distilled water was used in all the experiments. Allium cepa L. peels was collected from the local household and was washed carefully with double distilled water and then dried in oven at 60 °C until steady weight. The dry biomass was then grounded in a stainless steel grinder and sieved.
2.2 Preparation of aqueous extract of peels of Allium cepa L.
Fine powdered peels of Allium cepa L. (AcL) (10 g) was placed in a 500 ml Erlenmeyer flask containing 450 ml distilled water, and then heated at 70 °C for 20 minutes (min). This was followed by centrifugation at 4000 rpm for 20 min and the supernatant was then filtered. The extract was stored in a refrigerator at 4 °C for further use. The prepared extract was used within one week of preparation.
2.3 Synthesis of Au NCs
An aliquot (20 ml) of extract peels of AcL of various concentrations (10%, 20%, 30% and 40%) were added separately to 0.1 M (20 ml) HAuCl4·3H2O and heated at 70 °C for 20 min followed by slow cooling at room temperature. Another set of Au NCs were prepared by varying the reaction temperatures (40 °C, 60 °C, 80 °C and 100 °C) by heating a mixture of 0.1 M (20 ml) HAuCl4·3H2O with 10% (20 ml) AcL peel extract solution for 20 min. These solutions were then allowed to stabilize for some time. After stabilization, the solution with red sediment was formed at the bottom of the container indicating the formation of Au NCs. These were then centrifuged, filtered and the residues were washed several times with double distilled water to remove unbound polymers to yield Au NCs.
2.4 Evaluation of photo catalytic activity
Photodegradation of RB and MB dyes was evaluated by dispersing 10 mg of Au NCs in 200 ml of 10−4 M aqueous solutions of these dyes. To attain the adsorption–desorption equilibrium of dye on the surface of the NPs, the suspended solution was allowed to stand for 1 h in the dark before solar irradiation. These dyes were then exposed to sunlight. The experiments were carried out on a sunny day at Silchar city between 10 a.m. to 3 p.m. (atmospheric temperature 32–36 °C). At regular intervals of time, 4 ml of suspensions were withdrawn and immediately centrifuged. The progress of the reaction was monitored using UV-vis spectroscopy at regular intervals of time.
2.5 Catalytic activity of the fabricated Au NCs
To evaluate the efficacy of the fabricated Au NCs as catalyst, the conversion of 4-NP to 4-AP in aqueous medium in presence of NaBH4 was carried out at room temperature. In a standard quartz cuvette having 1 cm path length, 2.6 ml of water and 60 μl of 6.07 × 10−3 M 4-NP were taken separately and the absorbance was recorded using the UV-visible spectrometer. To this 4-NP solution, 350 μl of aqueous NaBH4 (0.1 M) was added and the absorbance was noted. Thereafter, 150 μl of 0.01 g of Au NCs was added to that mixture and the absorbance was until the peak due to the 4-NP was no longer observed.
2.6 Antibacterial activity of the synthesized Au NCs
The antibacterial activity of the synthesized Au NCs was evaluated via agar well diffusion method against a gram negative bacterium, Escherichia coli. In a nutrient broth the bacteria was grown at 37 °C for 24 hours. By dissolving 3.9 g of solid Mueller Hinton agar in 100 ml of distilled water, the Mueller-Hinton agar was prepared. Each Mueller Hinton agar (25 ml) was poured into each Petri dish and was allowed to solidify. Then they were cut and the turbidity of the bacterial inoculums was adjusted and was swabbed over the entire surface of the agar plate. The Au NCs were impregnated in the wells and standard antibiotic tetracycline was employed as a positive control and wells were filled with sample. The zones of inhibition were recorded at 24 hours after incubation at 37 °C.
2.7 Characterization of the synthesized Au NCs
The UV-vis absorption spectra of the synthesized NCs were recorded on Cary 100 Bio spectrophotometer (λmax in nm) equipped with 1 cm quartz cell. The photoluminescence measurements were carried out using Perkin Elmer LS 45 spectrofluorometer. The TEM, HR-TEM images, and SAED pattern were recorded using JEOL-JEM 2100 transmission electron microscope operated at an accelerating voltage of 200 kV. The TEM samples of the NCs were prepared by placing the solution drops over the carbon coated copper grids and allowing the solvent to evaporate at room temperature. An energy dispersive X-ray spectroscopy (EDX) analyzer was attached to the TEM operating mode and was used to analyze the components in the NCs. The FTIR spectra were measured using Bruker Hyperion 3000 FTIR spectrometer, using thin, transparent KBr pellets prepared by pressing a mechanically homogenized mixture of dried sample with dehydrated KBr. The XRD pattern was recorded using a Phillips X'Pert Pro diffractometer with Cu Ka radiation of wavelength 1.5418 Å.
3. Results and discussion
3.1 UV-vis spectroscopy
The optical properties of Au NCs are largely dependent on their size, template, surface ligand and the surrounding medium.15 Previous studies have shown that NCs with dimension lesser than 3 nm exhibits molecule like properties and do not possess apparent SPR in the visible range.16 However, for NCs greater than 3 nm up to 10 nm, a SPR band may be observed owing to the interband transition of the Au core 5d electrons.2,16–18. (Fig. 1(a) and (b)) respectively displayed the absorption spectra of the fabricated Au NCs using different concentrations of AcL extracts (10%, 20%, 30% and 40%) and at different heating temperatures (40 °C, 60 °C, 80 °C and 100 °C). Both the spectra revealed peak at around ∼540 nm which increased monotonically with enhancement of both concentration and temperature indicating that the absorption arose due to the interband transition of the Au core 5d electrons.16–18
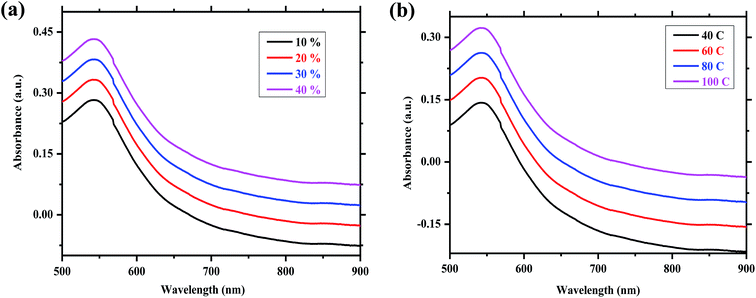 |
| Fig. 1 ((a) and (b)) depicted the UV-vis spectra of the fabricated Au NCs using different concentrations of AcL extracts (10%, 20%, 30% and 40%) and at different heating temperatures (40 °C, 60 °C, 80 °C and 100 °C) respectively. | |
3.2 Photoluminescence spectroscopy
(Fig. 2(a) and (b)) respectively depict the fluorescence spectra of the synthesized Au NCs at different concentrations of AcL extracts (10%, 20%, 30% and 40%) and at different reaction temperatures (40 °C, 60 °C, 80 °C and 100 °C). A fixed excitation wavelength (λexcit) of 350 nm was employed. The growth of the NCs as a function of concentration of extract and heating temperature were studied. Both the spectra revealed that at initial stages, no emission spectra were observed. Moreover, with the increase in concentration (Fig. 2(a)) as well as with the enhancement of reaction temperature (Fig. 2(b)), an emission band centered at ∼640 nm owing to Au–S charge transfer transition of Au NCs were found which exhibited red shift. This red shift was attributed to the slow growth of the Au NCs.
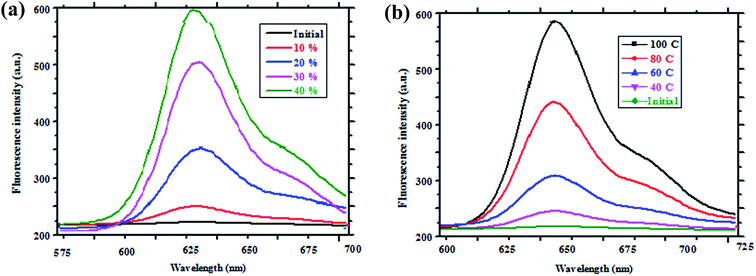 |
| Fig. 2 ((a) and (b)) respectively displayed the fluorescence spectra of the synthesized Au NCs at different concentrations of AcL extracts (10%, 20%, 30% and 40%) and at different reaction temperatures (40 °C, 60 °C, 80 °C and 100 °C). | |
Hence, the above results confirmed that the fluorescence emission spectrum (λexcit = 350 nm) of the materials exhibited a maximum emission wavelength of 640 nm with enhanced intensity of the band depicting that the absorption of the band depended on both the concentration and temperature. The fabricated Au NCs were found to be quite stable.
3.3 TEM and SAED studies
TEM images of the Au NCs formed using different concentrations of AcL extract (10% and 40%) are presented in (Fig. 3(a) and (d)), respectively. (Fig. 3(b) and (e)) represented the HRTEM micrographs and (Fig. 3(c) and (f)) depicted the SAED pattern of the Au NCs formed using 10% and 40% of the extract respectively. The TEM micrographs (Fig. 3(a) and (d)) revealed that the fabricated Au NCs are well dispersed with an average diameter of 4–6 nm and 7–9 nm (shown in the inset of Fig. 3(a) and (d)) indicating that the cluster size increased with increased concentration of the extract. The lattice spacing determined from the HRTEM was observed to be 0.24 nm (Fig. 3(b) and (e)) and were found to be in consistent with the (111) plane of Au crystal lattice.19
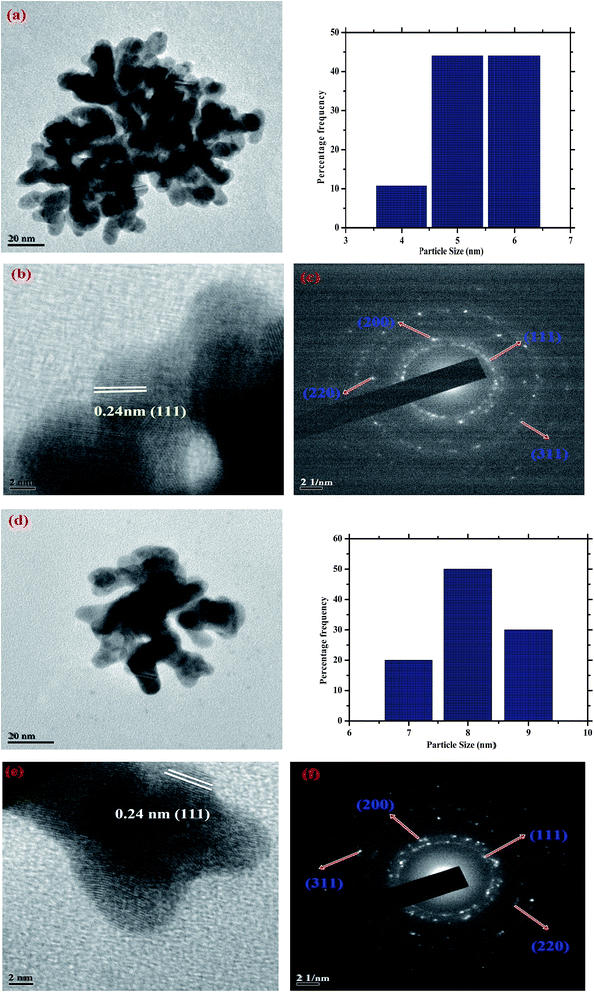 |
| Fig. 3 ((a) and (d)) represented the TEM images (inset size distribution) of the Au NCs formed using different concentrations of AcL extracts (10% and 40%), ((b) and (e)) represented the HRTEM images and ((c) and (f)) depicted the SAED pattern of the Au NCs formed using 10% and 40% of the extract respectively. | |
The SAED pattern (Fig. 3(c) and (f)) displayed a series of diffraction rings corresponding to (111), (200), (220) and (311) lattice planes of well organised Au nanocrystals.19 Hence, it was evident that the fabricated cluster size can be tuned by varying the concentration of the AcL extract. (Fig. 4(a) and (d)) corresponded to the TEM images of the synthesized Au NCs at 40 °C and 100 °C, respectively. The SAED pattern of (Fig. 4(a) and (d)) were shown in (Fig. 4(c) and (f)), respectively. The TEM micrographs (Fig. 4(a) and (d)) revealed that NCs with average size of 3–6 nm and 4–7 nm (shown in the inset of (Fig. 4(a) and (d))) were formed respectively indicating that the cluster size increased with the increase of temperature.
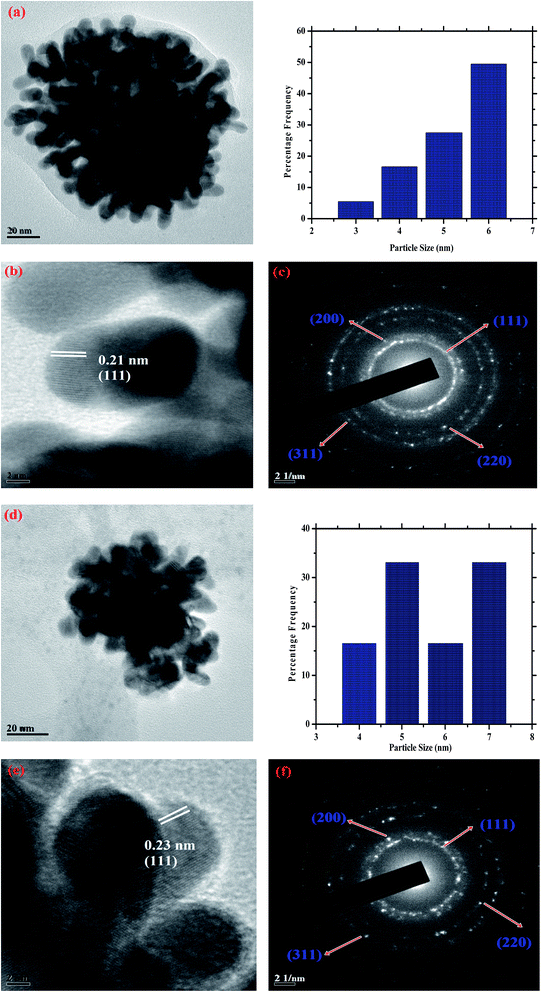 |
| Fig. 4 ((a) and (d)) corresponded to the TEM images (inset size distribution) of the synthesized Au NCs at 40 °C and 100 °C respectively. The SAED pattern of ((a) and (d)) are shown in ((c) and (f)) respectively and ((b) and (e)) are the magnified images of ((a) and (d)) respectively. | |
HR-TEM pattern indicated clear lattice fringes with fringe spacing of 0.21 nm (Fig. 4(b)) and 0.23 nm (Fig. 4(e)) that matches well with the (111) plane of Au crystal lattice.19
The d-spacings determined from SAED pattern (Fig. 4(c) and (f)) were 2.34, 2.05, 1.41 and 1.19 Å and could be indexed corresponding to reflections from (111), (200), (220) and (311) planes of Au NCs.19 Thus, it is apparent that the cluster size can be tuned with the change in temperature. Henceforth, the TEM pattern indicated that the cluster diameter can be adjusted by varying both the concentration and heating temperature.
3.4 XRD studies
To determine the crystal structure of the fabricated Au NCs, the XRD analysis has been carried out (Fig. 5). There were four different peaks at 2θ = 38°, 43°, 64° and 78° in the patterns and these peaks were the same as JCPDS (no-71-4610), which corresponded to the (111), (200), (220) and (311) planes of fcc of metallic gold. Thus the XRD depicted the crystalline nature of the cluster and was in accordance with the SAED results.20 The average crystalline size of Au NCs were calculated from the XRD (111) peak by applying Scherrer's equation: D = kλ/β
cos
θ, where λ is the X-ray wavelength of Kα (1.54 Å), θ is the Bragg angle, β is the full width at half-maximum in radians, and k (0.9) is an unknown shape factor.21 The average calculated crystalline size of the fabricated Au NCs was found to be 6 nm.
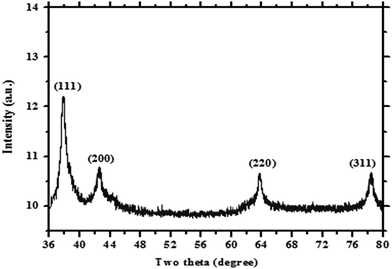 |
| Fig. 5 Represented the XRD pattern of the fabricated Au NCs. | |
Thus, the average crystalline size obtained from XRD was comparable to the cluster size obtained using TEM.
3.5 EDAX analysis
EDAX is a semi quantitative technique used to identify the elements present in the synthesized Au NCs. Fig. 6 illustrated the EDAX spectrum of the synthesized Au NCs. The EDAX spectra consolidated the existence of Au and S in the sample. In addition to these, signals corresponding to P, Si, K and Fe were also recognised and could be attributed owing to biomolecules such as flavonoids and amino acid cysteine and its derivatives present on the surface of the biosynthesized Au NCs.22 The EDAX spectra also showed weak signals at around 8 and 9 keV which was due to the Cu perhaps associated with the SEM grid used.23 The weight and atomic percentage of elements obtained from the EDAX analysis are shown in Table T1 (ESI T1†).
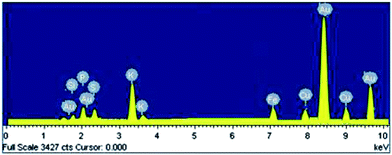 |
| Fig. 6 Illustrated the EDAX spectrum of the synthesized Au NCs. | |
3.6 FTIR studies
The FTIR measurements were carried out to perceive the functional groups accountable for the reduction as well as capping of the fabricated Au NCs (Fig. 7). The assignments of FTIR bands of the AcL extract and the synthesized Au NCs are summarized in Table T2 (ESI T2†). The main peaks that were recognized in the IR spectra of the extract were at 3440, 2550, 1611, 1358, 1012, 719 and 550 cm−1 which could be attributed to hydrogen bonded O–H stretching vibration of phenol overlapped with N–H stretching, S–H stretching vibration, amide I band owing to the stretching vibration of C
O in amide bond, amide II band due to N–H bending vibrations in amide bonds/C–H bond in aromatic hydrocarbon, C–O–H bending of carboxylic acid or in plane O–H bending vibration, C–H bending vibration of aromatic hydrocarbon and N–H wagging or out of plane O–H bending vibration, respectively.24
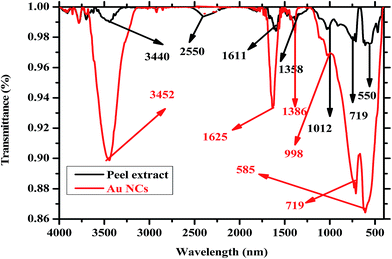 |
| Fig. 7 Displayed the FTIR spectra of the AcL extract and the fabricated Au NCs. | |
However, for the AcL extract mediated fabrication of Au NCs, the peak attributed to hydrogen bonded O–H stretching vibration of phenol overlapped with N–H stretching and transformed to 3452 cm−1 and happened to be relatively strong and broad. Additionally, no band attributed to S–H stretching vibration was observed in case of fabricated Au NCs. However, the amide I band, amide II band/C–H bond in aromatic hydrocarbon, C–O–H bending of carboxylic acid or in plane O–H bending vibration, and N–H wagging or out of plane O–H bending vibration shifted to 1625, 1386, 998 and 585 cm−1 respectively in case of the synthesized Au NCs. Hence, the above mentioned data indicated the presence of O–H, S–H, amide C
O, N–H and COOH group present in the AcL extract. These functional groups could be assigned owing to the presence of flavonoids and amino acid cysteine and its derivatives.7 The peak transformations in the IR spectra of the synthesized Au NCs were related to the NH2, SH and OH groups indicating that these functional groups were involved in their fabrication and capping.
3.7 Role of Allium cepa L. peel extract in the fabrication of Au NCs
Allium cepa L. peel extract contains biomolecules such as flavonoids, carbohydrates, saponins, amino acid cysteine and its derivatives such as S substituted cysteine sulphoxides and γ-glutamyl peptides. Additionally, it also contains vitamins such as B1, B2, B6, biotinic, nicotinic, folic, pentatonic and vitamin C.7 Though it is very difficult to suggest the exact mechanism for the formation of Au NCs, still it is perceived that in the biofabrication of Au NCs, the biomolecules present in the extract plays a vital role. It is considered that the amino acid cysteine and its S-substituted derivatives present in the extract are mainly responsible for the formation of cluster (as established from the FTIR spectra). It is believed that the precursor of Au(III) are first reduced to Au(I) and Au(0), when the aqueous solutions of the extract is added owing to the coating agent present in the extract (here due to the thiol group).25 Then, Au(I) is bounded to the coating agent on the outer sphere and Au(0) is wrapped in the center of the cluster by Au–Au interaction.25
3.8 Evaluation of the photocatalytic activity of the synthesized Au NCs
To access the photocatalytic activity of the fabricated Au NCs in aqueous medium in presence of sunlight, two different types of dyes RB and MB were chosen. The dye degradation process doesn't start immediately and the degradation process was recorded by monitoring alterations in the optical absorption spectrum of the reaction mixture, which was made free from the catalyst by centrifugation. At first, a control experiment was executed to verify the photocatalytic activity of the fabricated Au NCs. It was observed that under dark conditions in presence of Au NCs, both the solutions of dye showed negligible degradation (ESI†) (Fig. S1(a) and (c)). Further, when the degradation process was monitored in presence of sunlight without any catalyst, no photochemical reaction i.e. no degradation process was observed (Fig. S1(b) and (d)†). Hence, the result indicated that both solar irradiation and photo catalyst are essential for the efficient removal of these hazardous dyes.
Consequently, we carried out the degradation process in presence of Au NCs under solar irradiation and recorded the absorbance spectra. It was noticed that the absorption peak corresponding to RB and MB at 540 nm and 664 nm depicted fast degradation and faded away after 180 and 145 min, respectively (Fig. 8(a) and (b)). The percentage efficiency of photodegradation of dye with time was graphically represented by Fig. 8(c) and it was detected that about 98.9 and 95.1% of RB and MB was degraded respectively within 180 and 145 min in presence of sunlight using the fabricated Au NCs.
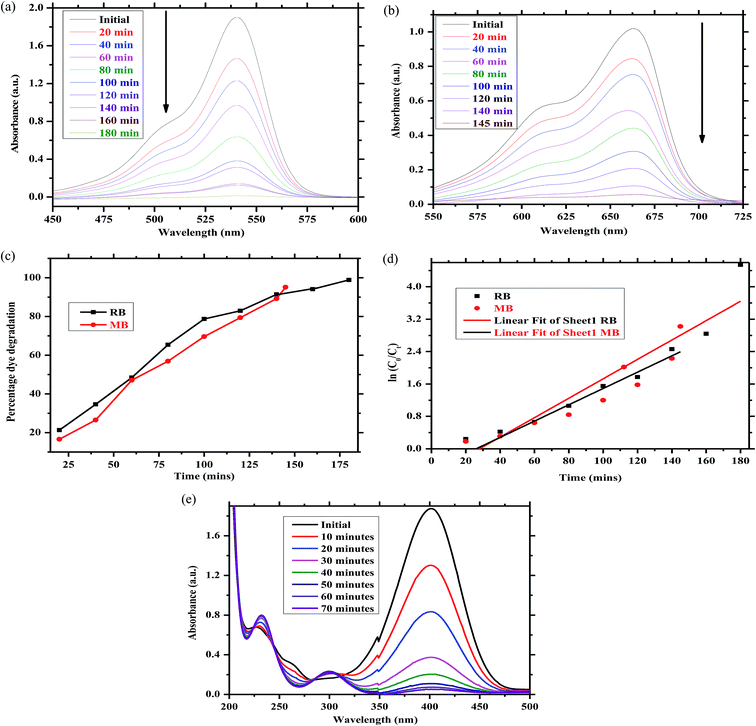 |
| Fig. 8 (a) represented the absorption spectra for photocatalytic degradation of RB using Au NCs. (b) represented the absorption spectra for photocatalytic degradation of MB using Au NCs. (c) represented the degradation capacity of the manufactured NCs for RB and MB. (d) represented the plot of ln (C0/Ct) vs. irradiation time t for the degradation of dyes. (e) represented the absorption spectra for the reduction of 4-NP by NaBH4 in aqueous medium in presence of synthesized Au NCs as catalyst. | |
Hence, it was perceived that the rate of degradation of dyes solely depended upon the chemical structure of the target dye. The rates of degradation of these dyes in presence of NCs were according to pseudo-first order reaction and their kinetics may be expressed as follows.26
|
ln (C0/Ct) = kt
| (1) |
where
Ct and
C0 are the concentration of the dyes at time
t and 0, respectively;
k = pseudo-first order rate constant and
t = time in min.
Fig. 8(d) represented the plot of ln
(C0/Ct) vs. irradiation time t for the degradation of dyes. The plot displayed a linear relationship and hence, slope of the line represented the rate constant (k) for the degradation of dyes. The value of k was found to be 2.92 × 10−3 and 2.86 × 10−3 min−1 for RB and MB, respectively. Table 1 depicted a comparison of dye degradation between the present study and the other investigations reported in the literature. It is apparent from the Table 1 that the catalysis reaction rate is either equivalent or better in our present process compared to most of the reported literature data. In our present case, we believe that due to the presence NCs, the electron transfer rate was much faster which increased the catalysis reaction rate.30
Table 1 Comparative analysis of the experimental output of the present study and studies conducted by other researches for the photocatalytic degradation of dyes using NPs
NPs/NCs |
Dye |
Volume (ml) |
% degradation |
Time (min) |
Catalyst conc. (g) |
Ref |
Au |
RB |
200 |
98.9 |
180 |
0.10 |
Present study |
Au |
MB |
200 |
95.1 |
145 |
0.10 |
Ag |
MB |
100 |
100 |
3600 |
0.10 |
27 |
Sn–SnO2 |
MB |
50 |
98 |
180 |
0.10 |
28 |
ZnO–SnO2 |
MB |
250 |
100 |
240 |
0.10 |
28 |
Graphen-e-polyaniline |
RB |
100 |
56 |
180 |
0.10 |
29 |
3.9 Probable mechanism for the photocatalytic activity of the Au NCs
A photocatalytic mechanism involves two components: photo and catalysis. The former portion is related with interaction of light material which comprises of photon absorption, charge creation, dynamics, and surface trapping. While, the later portion is correlated with surface reactivity and surface radical formation that is connection among H2O, O2, and organic pollutants.30 Therefore, the photocatalytic mechanism can be summed up as follows:
Initially, the solar irradiation is absorbed by the Au NCs which are photo excited and experiences plasmonic decay by three mechanisms:31
1. The absorbed molecules absorb photon and gains energy from the plasmonic structure of Au NCs and the process is known as elastic radiative re-emission of photons.
2. Next, a non radiative Landau damping is experienced by the photon energy which converts it to a single e−/h+ pair excitations. Then via columbic inelastic scattering, the excited primary electrons generate many other electrons.
3. Lastly, due to the interaction between the adsorbate and the excited surface plasmons, the induction of a direct electron injection into the adsorbate takes place.
Secondly, owing to plasmonic decay, the electrons and holes generated can react with O2 and H2O molecules to furnish active species; anionic super oxide radical (O2−˙) and hydroxyl radical (OH˙), respectively. In the next step, protonation of the superoxide ion (O2−˙) occurs which leads to the formation of hydro peroxyl radical (HO2˙). These hydro peroxyl radical then converts to H2O2 which ultimately dissociates into highly reactive hydroxyl radicals (OH˙). Lastly, both reduction as well as oxidation takes place on the surface of the photocatalyst.
Therefore, the complete degradation process can be represented by the Scheme 1, and the related reactions are shown in eqn ((2)–(10)).
|
Au + hν → h+ (Au) + e− (Au)
| (2) |
|
H2O (ads) + h+ → OH˙ + H+ (ads)
| (3) |
|
O2−˙ (ads) + H+ ⇄ HOO˙ (ads)
| (5) |
|
2HOO˙ (ads) → H2O2 (ads) + O2
| (6) |
|
H2O2 (ads) → 2OH˙ (ads)
| (7) |
|
Dye + OH˙ → CO2 + H2O (dye intermediates)
| (8) |
|
Dye + h+ → oxidation products
| (9) |
|
Dye + e− → reduction products
| (10) |
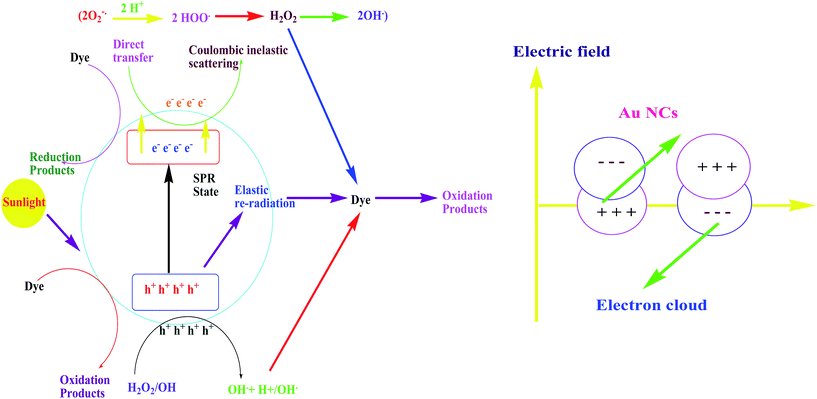 |
| Scheme 1 Schematic representation of the photodegradation process using Au NCs under solar irradiation. | |
3.10 Evaluation of photostability of the Au NCs
To illustrate the photo stability of the catalyst, recycling reactions were carried out for the degradation of both RB and MB. In each test, Au NCs (catalyst) was separated from the solution, washed with ethanol and dried in vacuum.32 It was found that the catalyst exhibits excellent stability even after 3 cycles and the results are depicted in ESI (S2).†
3.11 Identification of the intermediate products of dye degradation
The intermediates generated during the degradation process were analyzed using liquid chromatography-mass spectroscopy (LC-MS) technique and were identified by comparison with commercial standards and by interpretation of their fragment ions in the mass spectra.
All the identified intermediate products of the dye degradation are presented in the ESI (S3).†
3.12 Catalytic reduction of 4-NP to 4-AP employing Au NCs as catalyst in aqueous medium
To explore the catalytic efficiency of the fabricated Au NCs, the reduction of 4-NP to 4-AP was examined in the presence of NaBH4. 4-NP in aqueous medium exhibited an absorption band centered at 317 nm (Fig. S4(a)†). However, on subsequent addition of freshly prepared solution of NaBH4 to the 4-NP solution led to a bathochromic shift to 403 nm (Fig. S4(a)†) and the light yellow colour solution changed to intense yellow owing to the formation of 4-nitrophenolate ions in alkaline condition.33 In absence of any catalyst, the peak owing to 4-nitrophenolate doesn't undergoes any reduction and the peak at 403 nm remains unaltered even up to 3 days (Fig. S4(b)†).
The yellow colour of 4-NP slowly faded after the addition of 150 μl of 0.01 g of Au NCs and finally completely vanished on complete reduction of 4-NP. This decolourization reaction was monitored spectrophotometrically with time. It has been noticed that the peak owing to 4-NP successively decreases with time and there is a simultaneous appearance of a new peak due to 4-AP at 298 nm with progressive increase in intensity (Fig. 8(e)). The complete reduction of 4-NP was completed within 70 min. Considering much higher concentration of NaBH4 compared to 4-NP, the reaction was found to follow pseudo-first order kinetics and the rate constant was estimated by a linear plot of ln
(Ct/C0) vs. reduction time in minutes and the rate constant was found to be 5.71 × 10−2 min−1 (Fig. S4(c)†). About 97.3% of 4-NP was reduced to 4-AP employing NaBH4 in presence of Au NCs as catalyst (Fig. S4(d)†). Hence, Au NCs was found as an efficient catalyst in the reduction of 4-NP in presence of NaBH4.
3.13 Mechanism of reduction of 4-NP to 4-AP
The mechanism of reduction of 4-NP to 4-AP by NaBH4 in presence of Au NCs is illustrated and represented in ESI (S5).†
3.14 Antibacterial activity of the fabricated Au NCs
The efficacy of Au NCs as ideal antibacterial agents arises owing to their wide applicability and varied properties such as non-toxicity, polyvalent effect, ease of functionalization, detection and photo thermal capability.34 Literature survey revealed that their antibacterial potential can be attributed due to their ability to attach with bacterial membrane followed by membrane potential modification, ATP level reduction and inhibition of DNA, RNA and protein synthesis leading to cell lysis.35,36
Subsequently, in the present study we have explored and compared the antibacterial potential of the fabricated Au NCs, aqueous extract of AcL and tetracycline antibiotic as control against a gram negative bacterium, Escherichia coli at the concentration of 200 μg ml−1 by well diffusion method (Fig. 9). The results of the zone of inhibition around each well are represented in Table T3 (ESI T3†). Results indicated that Au NCs have highest bacterial susceptibility (30 mm), followed by the antibiotic (20 mm) and aqueous extract of AcL (6 mm) the least.
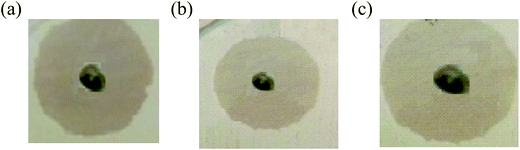 |
| Fig. 9 Represented the zone of inhibition produced by (a) antibiotic tetracycline, (b) AcL extract and (c) fabricated Au NCs against Escherichia coli bacteria. | |
The highest susceptibility of Au NCs arises due to their large surface area and high penetrating power which makes them easy to interact with the bacterial membrane, leading to transformations in the bacterial cell and ultimately leading to cell death.37 While the AcL extract also exhibited antibacterial activity to some extent due to the presence of amino acids in the extract but comparatively negligible as compared to the Au NCs.38
Hence, the result indicated that these fabricated Au NCs have considerable amount of antibacterial activity compared to that of the AcL extract.
4. Conclusions
Herein, we exploited the peel extracts of Allium cepa L., which doesn't have any utility and is considered as a common household waste material for the rapid fabrication of luminescent Au NCs at different reaction temperature and concentration of the extract. It is perceived that the amino acid cysteine and its S-substituted derivatives present in the extract are liable for the fabrication of the cluster. The present study is thus free from the use of any surfactant, solvent, capping agent, template and revealed the dual functional ability of the peel extract solutions. Hence, the aforesaid fabrication methodology is green, simple, economic and environment friendly method which is an improvisation in exploring the waste material for the development of Au NCs for sustainable environmental applications.
The Au NCs so developed was explored for the remediation of hazardous compounds (RB, MB and 4-NP) and was used as an antibacterial agent. The degradation of RB, MB and 4-NP followed pseudo first order kinetics with a degradation efficiency of 98.9, 95.1 and 97.3% respectively. The degradation products were analyzed using LC-MS technique and it was observed that the dye initially undergoes removal of oxy group and demethylation resulting in the formation of some intermediate products. The exhausted NCs were effectively regenerated and were analyzed using TEM and SAED pattern. The renewed NCs showed dye removal efficiency of 98.5, 97.3, 96.2% for RB and 94.8, 93.7 and 92.7% for MB respectively for 1st, 2nd and 3rd rounds of regeneration cycles.
Furthermore, the NCs were effectively found to inhibit the growth of E. coli bacteria. These high competences of the NCs have presented a promising and effective treatment methodology for the removal of dyes and phenols from the industrial effluents. Therefore, the present study has unfastened a pioneering way for synthesizing Au NCs and their applicability for the remediation of hazardous compounds and their ability as antibacterial agents. Hence, the fabrication of luminescent Au NCs by this technique and its utilization in the abatement of industrial effluents and as antibacterial agents are quite justified.
Acknowledgements
Tanur Sinha is grateful to NIT Silchar for providing the financial assistance and SAIF-NEHU Shillong, SAIF-IIT Bombay and CSMCRI-Gujarat for providing the TEM, FTIR, LC-MS EDAX and XRD facilities.
References
- Y. Yue, T. Y. Liu, H. W. Li, Z. Liu and Y. Wu, Microwave-assisted synthesis of BSA-protected small gold nanoclusters and their fluorescence-enhanced sensing of silver(I) ions, Nanoscale, 2012, 4, 2251–2254 RSC.
- L. Yan, Y. Cai, B. Zheng, H. Yuan, Y. Guo, D. Xiao and M. M. F. Choi, Microwave-assisted of BSA-stabilized and HAS-protected gold nanoclusters with red emission, J. Mater. Chem., 2012, 22, 1000–1005 RSC.
- H. Bönnemann and K. S. Nagabhushana, Metal Nanoclusters in Catalysis and Materials Science, Elsevier, 2008, ch. 2 Search PubMed.
- https://www.en.wikipedia.org/wiki/Nanoclusters.
- M. A. H. Muhammed, P. K. Verma, S. K. Pal, A. Retnakumari, M. Koyakutty, S. Nair and T. Pradeep, Luminescent quantum clusters of gold in bulk by albumin-induced core etching of nanoparticles: metal ion sensing, metal-enhanced luminescence, and biolabelling, Chem.–Eur. J., 2010, 16, 10103–10112 CrossRef.
-
(a) J. Zhang, Y. Yuan, G. Liang, M. N. Arshad, H. A. Albar, T. R. Sobahi and S. H. Yu, Microwave-facilitated rapid synthesis of gold nanoclusters with tunable optical property for sensing ions and fluorescent ink, Chem. Commun., 2015, 51, 10539–10542 RSC;
(b) M. O. Bello, I. O. Olabanji, M. A. Hammed and T. D. Okunade, Characterization of domestic onion wastes and bulb (Allium cepa L.): fatty acids and metal contents, Int. Food Res. J., 2013, 20, 2153–2158 Search PubMed.
- http://www.herballegacy.com/Wilson_Chemical.html.
- V. K. Gupta, Subhas, application of low-cost adsorbents for dye removal-a review, J. Environ. Manage., 2009, 90, 2313–2342 CrossRef CAS.
- A. Safavi and S. Momeni, Highly efficient degradation of azo dyes by palladium/hydroxyapatite/Fe3O4 nanocatalyst, J. Hazard. Mater., 2012, 201–202, 125–131 CrossRef CAS.
- A. G. Prado, L. B. Bolzon, C. P. Pedroso, A. O. Moura and L. L. Costa, Nb2O5 as efficient and recyclable photocatalyst for indigo carmine degradation, Appl. Catal., B, 2008, 82, 219–224 CrossRef CAS.
- R. Nilsson, R. Nordlinder, U. Wass, B. Meding and L. Belin, Asthma, rhinitis, and dermatitis in workers exposed to reactive dyes, Br. J. Ind. Med., 1993, 50, 65–70 CAS.
- S. Pandey and S. B. Mishra, Catalytic reduction of p-nitrophenol by using platinum nanoparticles stabilised by guar gum, Carbohydr. Polym., 2014, 113, 525–531 CrossRef CAS.
- T. Aditya, A. Pal and T. Pal, Nitroarene reduction: a trusted model reaction to test nanoparticle catalysts, Chem. Commun., 2015, 51, 9410–9431 RSC.
- D. Pathania, M. Kumari and V. K. Gupta, Fabrication of ZnS–cellulose nanocomposite for drug delivery, antibacterial and photocatalytic activity, Mater. Des., 2015, 87, 1056–1064 CrossRef.
- L. Y. Chen, C. W. Wang, Z. Yuan and H. T. Chang, Fluorescent gold nanoclusters: recent advances in sensing and imaging, Anal. Chem., 2015, 87, 216–229 CrossRef CAS.
- L. Zhang and E. Wang, Metal nanoclusters: new fluorescent probes for sensors and bioimaging, Nano Today, 2014, 9, 132–157 CrossRef CAS.
- S. Chen, 4-Hydroxythiophenol-protected gold nanoclusters in aqueous media, Langmuir, 1999, 15, 7551–7557 CrossRef CAS.
- C. F. Bohren and D. R. Huffman, Absorption and scattering of light by small particles, John Wiley and Son, New York, 2004, ch. 12 Search PubMed.
- P.-A. Buffat, M. Flueli, R. Spycher, P. Stadelmann and J.-P. Borel, Crystallographic structure of small gold particles studied by high-resolution electron microscopy, Faraday Discuss., 1991, 92, 173–187 RSC.
- T. Sinha and M. Ahmaruzzaman, A novel and greener approach for shape controlled synthesis of gold and gold–silver
core shell nanostructure and their application in optical coatings, Spectrochim. Acta, Part A, 2015, 145, 280–288 CrossRef CAS.
- T. Sinha and M. Ahmaruzzaman, High-value utilization of egg shell to synthesize silver and gold–silver core shell nanoparticles and their application for the degradation of hazardous dyes from aqueous phase-a green approach, J. Colloid Interface Sci., 2015, 453, 115–131 CrossRef CAS.
- N. Srivastava and M. Mukhopadhyay, Biosynthesis of SnO2 nanoparticles using bacterium Erwinia herbicola and their photocatalytic activity for degradation of dyes, Ind. Eng. Chem. Res., 2014, 53, 13971–13979 CrossRef CAS.
- A. Kumar, S. Aerry, A. Saxena, A. De and S. Mozumder, Copper nanoparticulates in guar-gum: a recyclable catalytic system for the Huisgen [3 + 2]-cycloaddition of azides and alkynes without additives under ambient conditions, Green Chem., 2012, 14, 1298–1301 RSC.
- T. Sinha and M. Ahmaruzzaman, Green synthesis of copper nanoparticles for the efficient removal (degradation) of dye from aqueous phase, Environ. Sci. Pollut. Res., 2015, 1–9 Search PubMed.
- M. Cui, Y. Zhao and Q. Song, Synthesis, optical properties and applications of ultra-small luminescent gold nanoclusters, Trends Anal. Chem., 2014, 57, 73–82 CrossRef CAS.
- T. Sinha, M. Ahmaruzzaman and A. Bhattacharjee, A simple approach for the synthesis of silver nanoparticles and their 2 application as a catalyst for the photodegradation of methyl violet 6B 3 dye under solar irradiation, J. Environ. Chem. Eng., 2014, 2, 2269–2279 CrossRef CAS.
- S. A. Ansari, M. M. Khan, M. O. Ansari, J. Lee and M. H. Cho, Visible light-driven photocatalytic and photoelectrochemical studies of Ag–SnO2 nanocomposites synthesized using an electrochemically active biofilm, RSC. Adv., 2014, 4, 26013–26021 RSC.
- A. K. Sinha, M. Pradhan, S. Sarkar and T. Pal, Large-scale solid-state synthesis of Sn–SnO2 nanoparticles from layered SnO by sunlight: a material for dye degradation in water by photocatalytic reaction, Environ. Sci. Technol., 2013, 47, 2339–2345 CrossRef CAS.
- S. Ameen, H. K. Seo, M. S. Akhtar and H. S. Shin, Novel graphene/polyaniline nanocomposites and its photocatalytic activity toward the degradation of rose bengal dye, Chem. Eng. J., 2012, 210, 220–228 CrossRef CAS.
- S. R. Kavitha, M. Umadevi, S. R. Janai and T. Balkrishanan, R Ramanibai fluorescence quenching and photocatalytic degradation of textile dyeing waste water by silver nanoparticles, Spectrochim. Acta, Part A, 2014, 127, 115–121 CrossRef CAS.
- F. Dong, T. Xiong, Y. Sun, Z. Zaiwang, Z. Ying, X. Feng and Z. Wu, A semimetal bismuth element as a direct plasmonic photocatalyst, Chem. Commun., 2014, 50, 10386–10389 RSC.
- S. Praharaj, S. Nath, S. K. Ghosh, S. Kundu and T. Pal, Immobilization and Recovery of Au Nanoparticles from Anion Exchange Resin: Resin-Bound Nanoparticle Matrix as a Catalyst for the Reduction of 4-Nitrophenol, Langmuir, 2004, 20, 9889–9892 CrossRef CAS.
- E. Lima, R. Guerra, V. Lara and A. Guzmán, Gold nanoparticles as efficient antimicrobial agents for Escherichia coli and Salmonella typhi, Chem. Cent. J., 2013, 7, 1–7 CrossRef PubMed.
- V. K. Gupta, D. Pathania, M. Asif and G. Sharma, Liquid phase synthesis of pectin–cadmium sulfide nanocomposite and its photocatalytic and antibacterial activity, J. Mol. Liq., 2014, 196, 107–112 CrossRef CAS.
- P. Prema and S. Thangapandiyan, In vitro antibacterial activity of gold nanoparticles capped with polysaccharide stabilizing agents, Int. J. Pharm. Pharm. Sci., 2013, 5, 310–314 CAS.
- Ł. Klapiszewski, T. Rzemieniecki, M. Krawczyk, D. Malina, M. Norman, J. Zdarta, I. Majchrzak, A. Dobrowolska, K. Czaczyk and T. Jesionowski, Kraft lignin/silica–AgNPs as a functional material with antibacterial activity, Colloids Surf., B, 2015, 134, 220–228 CrossRef PubMed.
- K. Adavallan and N. Krishnakumar, Mulberry leaf extract mediated synthesis of gold nanoparticles and its anti-bacterial activity against human pathogens, Adv. Nat. Sci.: Nanosci. Nanotechnol., 2014, 5, 025018–025026 CrossRef.
- K. Singh, M. Panghal, S. Kadyan, U. Chaudhary and J. P. Yadav, Antibacterial Activity of Synthesized Silver Nanoparticles from Tinospora cordifolia against Multi Drug Resistant Strains of Pseudomonas aeruginosa Isolated from Burn Patients, J. Nanomed. Nanotechnol., 2014, 5, 1000192–1000198 Search PubMed.
Footnote |
† Electronic supplementary information (ESI) available. See DOI: 10.1039/c5ra21495e |
|
This journal is © The Royal Society of Chemistry 2016 |