DOI:
10.1039/C5RA20403H
(Paper)
RSC Adv., 2016,
6, 4145-4150
Fabrication of an Ag/Fe2O3/ZnO ternary composite with enhanced photocatalytic performance†
Received
2nd October 2015
, Accepted 10th December 2015
First published on 15th December 2015
Abstract
A novel Ag/Fe2O3/ZnO ternary composite was fabricated using the chemical deposition and photochemical deposition methods. Its structure and optical properties were characterized using X-ray diffraction (XRD), scanning electron microscopy (SEM), transmission electron microscopy (TEM) and ultraviolet-visible spectrophotometry. The Ag/Fe2O3/ZnO ternary composite exhibited a greater improvement in photocatalytic activity compared with the Fe2O3/ZnO or Ag/ZnO binary composite. The degradation rate of Ag/Fe2O3/ZnO towards methyl orange (MO) and iodoform (CHI3) within 120 min was 80% and 94%, respectively. More importantly, the Ag/Fe2O3/ZnO composite had a much higher degradation efficiency towards iodoform, which indicates its efficient and selective degradation characteristics. The results show that the Ag/Fe2O3/ZnO ternary composite holds great potential in the removal of carcinogenic iodoform from sewage.
1. Introduction
Environment and human health are now threatened by the large amount of pollutants in wastewater. This threat is still great despite the fact that there is a low amount of halogenated hydrocarbon in wastewater, for example, iodoform, which can cause cancer.1–3 Thus, the removal of such toxic organic pollutants in wastewater is a highly essential issue. Semiconductor photocatalysts, such as TiO2 and ZnO, can be a green and efficient technology in the field of sewage treatment, because they can effectively dispose many organic pollutants in the sewage compared with other approaches. In addition, most photocatalytic materials are non-toxic, stable and low-cost.4,5
Although ZnO has numerous advantages, such as high quantum efficiency, non-toxicity and strong ultraviolet absorption,6 its catalytic efficiency is still not ideal. The main reasons for this include the following reason: (1) the band gap of ZnO is too wide, only the ultraviolet part of sunlight can be used; (2) ZnO is an amphoteric oxide semiconductor, it easily dissolves in strong acid and alkali solution; (3) the electron–hole pairs in ZnO are easy to recombine, which reduces its photocatalytic efficiency. In this regard, combining ZnO with other metal compounds, such as CuO and Fe2O3, has been proven to be effective in improving the photocatalytic performance of ZnO. However, ZnO binary composite materials also have some defects. They cannot selectively photodegrade some hazard pollutants in wastewater, especially halogenated hydrocarbons. Furthermore, their degradation efficiency decreases obviously when there is a variety of pollutants in wastewater. Recent studies have found that ZnO-based ternary composites have greater superiority than binary composites.7,8 Moreover, they have the function of selective degradation of some pollutants.9 In addition, it has been found that Ag has strong adsorption of highly carcinogenic halogenated hydrocarbon.10
In this study, an Ag/Fe2O3/ZnO ternary composite was synthesized via chemical deposition and photochemical deposition processes. The photocatalytic activity of Ag/Fe2O3/ZnO was investigated in comparison with Fe2O3/ZnO or Ag/ZnO. Furthermore, the effects of structure and interfacial electronic interactions of ZnO, Fe2O3 and Ag on their photocatalytic activity were also investigated. The Ag/Fe2O3/ZnO ternary composite had effective photodegradation towards MO and iodoform under the irradiation of simulated daylight, and it had a higher degradation efficiency towards iodoform. The results indicate the potential applications of Ag/Fe2O3/ZnO in sewage treatment.
2. Experiments
2.1 Preparation of the Ag/Fe2O3/ZnO ternary composite
First, a ZnO seed layer was prepared via a sol–gel method. Briefly, 40 mL anhydrous ethanol, 0.5 M zinc acetate-2-hydrate, and 0.5 M ethanol amine were mixed and stirred at 70 °C for 3 h. The resultant sol was transparent. The solution was then spin-coated on a glass substrate at 500 rpm for 10 s and 3000 rpm for 30 s, respectively. After that, the glass substrate was heated at 300 °C for 2 h to remove the solvent. Subsequently, the growth of ZnO nanorods (NR) was carried out by suspending the glass substrate in a 40 mL beaker that was filled with an equimolar aqueous solution of 0.1 M zinc nitrate hexahydrate (Zn(NO3)2·6H2O) and 0.1 M methenamine (C6H12N4, HMT) at 75 °C for 3 h.11,12
Fe2O3 nanoparticles were then deposited on the ZnO nanorods via photochemical deposition. The ZnO nanorod-coated substrate was immersed in a mixture of 4 mM FeCl3·6H2O and NaNO3·6H2O aqueous solution, and then irradiated with UV light for 1 h (wavelength 365 nm, power 56 W and working distance 0.3 m).13,14
Ag nanoparticles were further prepared via photochemical deposition. The Fe2O3/ZnO nanorod-coated substrate was immersed in a 1 mM AgNO3 and NaNO3·6H2O aqueous solution, and then irradiated with UV light (wavelength 365 nm, power 56 W and working distance 0.3 m) at room temperature for 40 min. The prepared sample was post-annealed in ambient air at 400 °C for 2 h to obtain a crystal structure.
2.2 Characterization
The crystal structure of the products was characterized via X-ray diffraction (XRD, Siemens D5005). Scanning electron microscopy (SEM, S4800) was employed to investigate their morphology. Transmission electron microscopy (TEM), high-resolution electron microscopy (HRTEM) and selected area electron diffraction (SAED) were conducted on a Tecnai G2 F20 S-TWIN. UV-vis absorption spectra were obtained using a UV-2401PC spectrometer.
2.3 Photocatalytic studies
Iodoform (halogenated hydrocarbon) and MO (organic dye) are two common pollutants in wastewater. Iodoform and MO were chosen to investigate the photo-degradation characteristic of the Ag/Fe2O3/ZnO ternary composite and Fe2O3/ZnO and Ag/ZnO binary composite. Samples were placed in 30 mL of iodoform solution (1.65 mg L−1) and MO solution (6.67 mg L−1) under the irradiation of simulated sunlight (xenon lamp, wavelength 320–780 mm, and light intensity at the sample is approximately 50 mW cm−2). Aliquots of iodoform and MO were taken every 30 min to analyze their ultraviolet absorbance.
To investigate the reusability of the Ag/Fe2O3/ZnO ternary composite, the Ag/Fe2O3/ZnO modified glass substrates were placed in 30 mL of iodoform solution (1.65 mg L−1) and MO solution (6.67 mg L−1) under the irradiation of simulated sunlight for 5 cycles. In each cycle, the iodoform and MO solutions were degraded by Ag/Fe2O3/ZnO for 120 min and then their maximum absorbance was measured. In the next cycle, the solutions of iodoform and MO were replaced, and the same Ag/Fe2O3/ZnO modified glass substrates were reused to degrade iodoform and MO for 120 min and then their maximum absorbance was measured.
3. Result and discussion
3.1 Characterization of materials
Fig. 1 shows the XRD pattern of the as-prepared sample. The typical diffraction peaks (0 0 2), (1 0 2), (1 1 0), (1 0 3), (2 0 1) of hexagonal wurtzite ZnO are observed, which are in good agreement with the standard patterns (JSPDS no. 36-1451). In addition, three diffraction peaks (2 0 6), (2 1 12), (5 2 1) (JCPDS no. 13-0458) of Fe2O3 and four diffraction peaks (2 2 0), (3 1 1), (3 3 1), (4 2 0) (JSPDS no. 01-1164) of Ag are observed, which reveal that the obtained Ag/Fe2O3/ZnO ternary composite has a good crystalline structure.
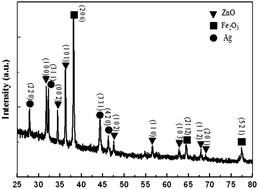 |
| Fig. 1 XRD patterns of the Ag/Fe2O3/ZnO ternary composite. | |
The as-prepared samples were characterized via SEM. As shown in Fig. 2a, it is obvious that the wurtzite ZnO rods have a hexagonal structure, and their surface is relatively smooth (Fig. 2a inset). Under the irradiation of UV light, the Fe2O3 nanoparticles are coated on the surface of ZnO to form a Fe2O3/ZnO binary composite (Fig. 2b), which results in a rough layer on the nanorods (Fig. 2b inset). After the decoration of Ag nanoparticles, granular materials are observed on the surface of Fe2O3/ZnO (Fig. 2c inset), which indicates the formation of the Ag/Fe2O3/ZnO ternary composite.
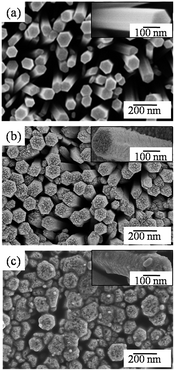 |
| Fig. 2 SEM images of (a) ZnO nanorod arrays, (b) Fe2O3/ZnO binary composite and (c) Ag/Fe2O3/ZnO ternary composite. | |
To further clarify the structure and morphology of the Ag/Fe2O3/ZnO ternary composite, HRTEM was performed. As shown in Fig. 3a, it is noted that the product has hierarchical structures. The high-magnification image of Fig. 3a (indicated by the white rectangle) suggests that the lattice spacing is about 0.248 nm, 0.155 nm and 0.144 nm (Fig. 3b), which correspond to the (1 0 1) plane of ZnO, (5 2 1) plane of Fe2O3, and (2 2 0) plane of Ag. The data indicate the existence of ZnO, Fe2O3 and Ag in the composite. The corresponding selected area electron diffraction (SAED) patterns further confirm the successful formation of the Ag/Fe2O3/ZnO ternary composite (Fig. 3c).
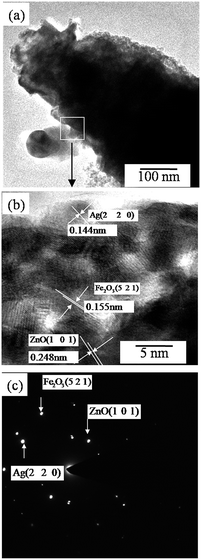 |
| Fig. 3 (a) TEM image of the Ag/Fe2O3/ZnO ternary composite, (b) HR-TEM image of the area indicated by the white rectangle in (a), and (c) the SAED pattern of (a). | |
3.2 Growth mechanism
The proposed growth mechanism of the Ag/Fe2O3/ZnO ternary composite is shown in Fig. 4. First, with the addition of HMT and Zn(OH)42−, the ZnO rod arrays were coated on the glass substrate with the ZnO seed layer under hydrothermal treatment at 75 °C (ref. 15) (Fig. 4a). Second, under UV irradiation, ZnO could form electronic–hole pairs. As a result, a great deal of OH− and Fe3+ were deposited on the surface of the ZnO nanorods to form [Fe(OH)4]− ions, and then they removed OH− and H2O to form Fe2O3 (ref. 16) (Fig. 4b). Likewise, with continued UV irradiation, more and more Fe2O3 particles were obtained, which led to a thick layer covered on the surface of ZnO (Fig. 4c). Finally, in the aqueous solution of AgNO3 and NaNO3·6H2O, Ag+ and OH− were deposited on the surface of Fe2O3/ZnO, thus generating nanosized silver particles17 (Fig. 4d).
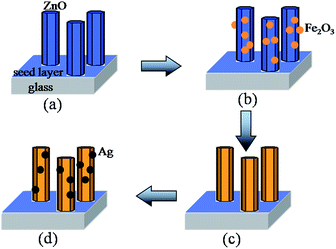 |
| Fig. 4 The proposed growth model of the Ag/Fe2O3/ZnO ternary composite, (a) ZnO nanorods were grown on a glass substrate, (b) Fe2O3 nanoparticles were attached to the surface of ZnO, (c) the Fe2O3 layer was coated on the surface of ZnO and (d) Ag nanoparticles were uniformly deposited on the surface of Fe2O3/ZnO to form the Ag/Fe2O3/ZnO ternary composite. | |
3.3 Photocatalytic activity
Fig. 5 shows the UV-vis absorption spectra of Ag/ZnO, Fe2O3/ZnO and Ag/Fe2O3/ZnO. Remarkably, the absorption wavelength of the Ag/Fe2O3/ZnO ternary composite is up to 650 nm, which is much wider than that of Ag/ZnO or Fe2O3/ZnO. This result indicates that Ag/Fe2O3/ZnO can improve the absorption intensity in the visible region. Therefore, the Ag/Fe2O3/ZnO ternary composite has great potential in terms of light degradation.
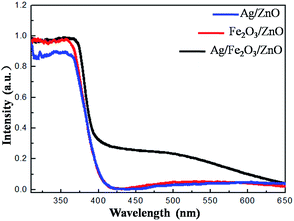 |
| Fig. 5 UV-vis absorption spectra of Ag/ZnO, Fe2O3/ZnO and Ag/Fe2O3/ZnO. | |
The photodegradation efficiency of Fe2O3/ZnO, Ag/ZnO, and Ag/Fe2O3/ZnO under illumination and without illumination (Fig. S1†) was investigated. To calculate the concentration of the iodoform and MO solutions, aliquots of the solutions were collected every 30 min and the absorption intensity at their maximum absorbance wavelength was measured. Fig. 6 shows the UV-vis absorption spectra of iodoform and MO degraded by Fe2O3/ZnO, Ag/ZnO, and Ag/Fe2O3/ZnO. When Ag/ZnO was used as the photocatalyst material, the characteristic absorption peaks of iodoform and MO dropped to about 44% and 50% of their initial absorption intensity after 120 min (Fig. 6a), respectively. As for Fe2O3/ZnO, the absorption peaks of iodoform and MO reduced to about 40% of their initial absorption intensity after 120 min (Fig. 6b). Interestingly, when the Ag/Fe2O3/ZnO composite was used, the absorption peaks of iodoform and MO rapidly decreased to about 5% and 22% of their initial intensity after 120 min, respectively (Fig. 6c). Obviously, the Ag/Fe2O3/ZnO ternary composite had a much higher degradation efficiency towards iodoform and MO compared to Ag/ZnO or Fe2O3/ZnO.
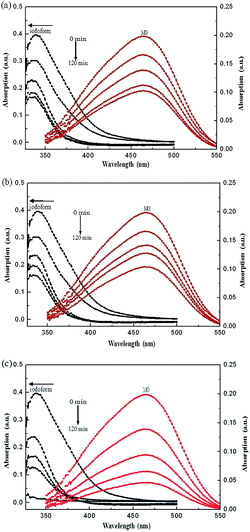 |
| Fig. 6 Time-dependent absorption spectra of iodoform and MO degraded by (a) Ag/ZnO, (b) Fe2O3/ZnO and (c) Ag/Fe2O3/ZnO. | |
By considering the peak intensity of MO and iodoform, we plotted the corresponding degradation rates of Fe2O3/ZnO, Ag/ZnO and Ag/Fe2O3/ZnO, as shown in Fig. 7. Degradation efficiency (y-axis), is defined as (1 − C/C0) × 100%, and the X-axis is reaction time in minutes. C0 is the initial concentration after the equilibrium adsorption, and C is the remaining concentration of iodoform and MO.18,19 Under the irradiation of simulated sunlight, the degradation efficiency of Ag/Fe2O3/ZnO for iodoform and MO was 94% and 80% (curve 1 and 2) after 120 min, respectively, which are much higher than that of Fe2O3/ZnO (58% and 60%, curve 3 and 4, respectively) or Ag/ZnO (56% and 50%, curve 5 and 6, respectively). In addition, in the mixture of three organics, the degradation efficiency of Ag/Fe2O3/ZnO for iodoform, MO and RhB was 89%, 45% and 40% (Fig. S2†), respectively. The data indicate that Ag/Fe2O3/ZnO exhibits a greater improvement in photocatalytic activity compared to Fe2O3/ZnO or Ag/ZnO. More importantly, the results also suggest that the Ag/Fe2O3/ZnO ternary composite has efficient and selective degradation towards iodoform.
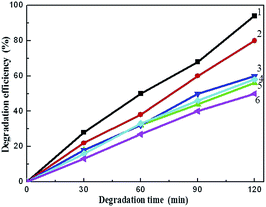 |
| Fig. 7 Photo-degradation efficiency of iodoform and MO under illumination. Degradation curve of Ag/Fe2O3/ZnO for iodoform (curve 1), and for MO (curve 2), degradation curve of Fe2O3/ZnO for MO (curve 3), and for iodoform (curve 4), degradation curve of Ag/ZnO for iodoform (curve 5), and for MO (curve 6). | |
3.4 Reusability of the Ag/Fe2O3/ZnO composite
To examine the reusability of the Ag/Fe2O3/ZnO ternary composite, it was used as the photocatalyst material for 5 cycles. The iodoform and MO solutions were replaced every 120 min and their maximum absorbance was measured. After every cycle, the corresponding degradation rates of Ag/Fe2O3/ZnO for iodoform and MO were about 92%, 89%, 86%, 85% and 82%, and 75%, 73%, 71%, 70% and 68% (Fig. 8b), respectively. The degradation efficiency of the Ag/Fe2O3/ZnO ternary composite for iodoform and MO solutions dropped to about 10% and 7% after 5 cycles (Fig. 8a), respectively. The reason for this may be that the Ag/Fe2O3/ZnO ternary composite participates in the process of degradation, and thus there is some consumption of Ag/Fe2O3/ZnO after each cycle.
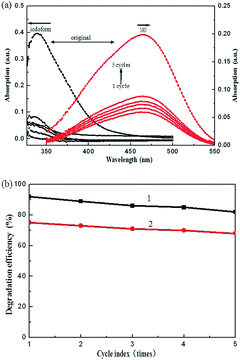 |
| Fig. 8 (a) Cycle time-dependent absorption spectra of iodoform and MO solutions degraded by the Ag/Fe2O3/ZnO ternary composite, and (b) cycle degradation curves of Ag/Fe2O3/ZnO for iodoform (curve 1) and MO (curve 2). | |
3.5 Proposed mechanism for the enhancement of photocatalytic activity by Ag/Fe2O3/ZnO
The different components in the Ag/Fe2O3/ZnO ternary composite, Fe2O3/ZnO and Ag/ZnO binary composite result in the difference in photocatalytic activities. The proposed photocatalytic degradation mechanism of iodoform and MO by Ag/Fe2O3/ZnO is illustrated in Fig. 9. The process can be described as follows: (1) under illumination, the electrons of ZnO in Ag/Fe2O3/ZnO are excited to generate electron–hole pairs, (2) the electrons in the valence band (VB) of ZnO are transferred to the (CB) conduction band of Fe2O3, (3) and then they are transferred to the CB of Ag. The high separation rate of electron–hole pairs makes it easier to form O2− active ions and OH˙ radicals on the surface of Ag/Fe2O3/ZnO to degrade iodoform and MO and to enhance photo-degradation activity. This process can be written as follows:20–25 |
H2O2 + O2− → OH˙ + O2 + OH−
| (4) |
|
OH˙ + pollutants → CO2 + H2O
| (5) |
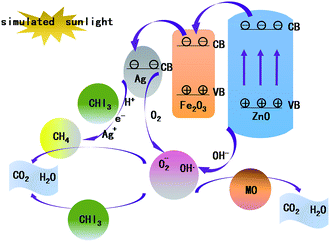 |
| Fig. 9 Schematic of iodoform and MO degradation by the Ag/Fe2O3/ZnO ternary composite. | |
At the same time, under the action of H+ and e−, iodoform can be decomposed by Ag due to the following reactions:26–28
|
Ag2+ + H2O → Ag+ + H+ + OH˙
| (7) |
|
CHI3 + 3H+ + 3e− → CH4 + 3I−
| (8) |
|
CH4 + OH˙ → H2O + CO2
| (9) |
This provides another way for the Ag/Fe2O3/ZnO to degrade iodoform, which can improve the degradation efficiency of iodoform. Taken together, the abovementioned reactions account for the selective property of the Ag/Fe2O3/ZnO ternary composite towards iodoform degradation.29
4. Conclusions
In summary, an Ag/Fe2O3/ZnO ternary composite has been successfully synthesized via chemical deposition and photochemical deposition in an aqueous solution. The Ag/Fe2O3/ZnO ternary composite shows a much higher photocatalytic performance compared with the Fe2O3/ZnO or Ag/ZnO binary composite. Moreover, the Ag/Fe2O3/ZnO ternary composite displays efficient and selective degradation of carcinogenic iodoform in sewage. This provides a promising strategy for the efficient degradation of halogenated hydrocarbons.
Acknowledgements
We acknowledge the financial support from the Ministry of Education of China (IRT1148), the Priority Academic Program Development of Jiangsu Higher Education Institutions (YX03001), the Natural Science Foundation of Jiangsu Province (BK20131376, BY2014015), the Opening Project of State Key Laboratory of New Ceramic and Fine Processing (KF201310) and the Synergistic Innovation Center for Organic Electronics and Information Displays.
References
- M. Veldhoen, K. Hirota, A. M. Westendorf, J. Buer, L. Dumoutier, J.-C. Renauld and B. Stockinger, Nature, 2008, 106–109 CrossRef CAS PubMed.
- J. W. Harper, Nature, 2007, 499–500 CrossRef CAS PubMed.
- L. L. Xu, B. Wei, W. L. Liu, H. L. Zhang, C. Y. Su and J. X. Che, Nanoscale Res. Lett., 2013, 536 CrossRef PubMed.
- J. F. Lei, L. B. Li, X. H. Shen, K. Du, J. Ni, C. J. Liu and W. S. Li, Langmuir, 2013, 13975–13981 CrossRef CAS PubMed.
- V. S. Vyas and B. V. Lotsch, Nature, 2015, 521, 41–42 CrossRef CAS PubMed.
- B. Yin, Y. Qiu, H. Q. Zhang, J. X. Lei, Y. Chang, J. Y. Ji, Y. M. Luo, Y. Zhao and L. Z. Hu, RSC Adv., 2015, 11469–11474 RSC.
- M. Lan, G. L. Fan, L. Yang and F. Li, RSC Adv., 2015, 5725–5734 RSC.
- J. J. D. Yoreo, P. U. P. A. Gilbert, N. A. J. M. Sommerdijk, R. L. Penn, S. Whitelam, D. Joester, H. Z. Zhang, J. D. Rimer, A. Navrotsky, J. F. Banfield, A. F. Wallace, F. M. Michel, F. C. Meldrum, H. Cölfen and P. M. Dove, Science, 2015, 6247 Search PubMed.
- J. J. Xue, S. S. Ma, Y. M. Zhou, Z. W. Zhang and P. Jiang, RSC Adv., 2015, 18832–18840 RSC.
- H. R. Liu, G. X. Shao, J. F. Zhao, Z. X. Zhang, Y. Zhang, J. Liang, X. G. Liu, H. S. Jia and B. S. Xu, J. Phys. Chem. C, 2012, 16182–16190 CAS.
- C. L. Zhang, M. F. Shao, F. Y. Ning, S. M. Xu, Z. H. Li, M. Wei, D. G. Evans and X. Duan, Nano Energy, 2015, 231–239 CrossRef CAS.
- L. Zhang, H. Z. Li, Y. Liu, Z. Tian, B. Yang, Z. B. Sun and S. Q. Yan, RSC Adv., 2014, 48703–48711 RSC.
- S. R. Wang, J. X. Zhang, J. D. Yang, X. L. Gao, H. X. Zhang, Y. S. Wang and Z. Y. Zhu, RSC Adv., 2015, 10048–10057 RSC.
- C. Karunakaran, P. Vinayagamoorthy and J. Jayabharathi, Langmuir, 2014, 15031–15039 CrossRef CAS PubMed.
- A. Wei, L. H. Pan and W. Huang, Mater. Sci. Eng., B, 2011, 1409–1421 CrossRef CAS.
- Y. J. Liu, L. Sun, J. G. Wu, T. Fang, R. Cai and A. Wei, Mater. Sci. Eng., B, 2015, 9–13 CrossRef.
- M. Behrens, F. Studt, I. Kasatkin, S. Kühl, M. Hävecker, F. Abild-Pedersen, S. Zander, F. Girgsdies, P. Kurr, B.-L. Kniep, M. Tovar, R. W. Fischer, J. K. Nørskov and R. Schlögl, Science, 2012, 893–897 CrossRef CAS PubMed.
- T. T. Jiang, X. Y. Qin, Y. Sun and M. Yu, RSC Adv., 2015, 65595–65599 RSC.
- J. N. Schrauben, R. Hayoun, C. N. Valdez, M. Braten, L. Fridley and J. M. Mayer, Science, 2012, 1298–1301 CrossRef CAS PubMed.
- N. T. Khoa, S. W. Kim, D.-H. Yoo, S. Cho, E. J. Kim and S. H. Hahn, ACS Appl. Mater. Interfaces, 2015, 3524–3531 CAS.
- K. Wu, J. Chen, J. R. McBride and T. Lian, Science, 2015, 632–635 CrossRef CAS PubMed.
- T. G. Xu, L. W. Zhang, H. Y. Cheng and Y. F. Zhu, Appl. Catal., B, 2011, 382–387 CrossRef CAS.
- Y. L. Lai, M. Meng and Y. F. Yu, Appl. Catal., B, 2010, 491–501 CrossRef CAS.
- X. M. Hou and L. X. Wang, RSC Adv., 2014, 56945–56951 RSC.
- B. X. Li and Y. F. Wang, Superlattices Microstruct., 2010, 615 CrossRef CAS.
- C. Comninellis, Electrochim. Acta, 1994, 1857–1862 CrossRef CAS.
- S. A. Ansari, M. M. Khan, M. O. Ansari, J. Lee and M. H. Cho, J. Phys. Chem. C, 2013, 27023–27030 CAS.
- C. L. Parworth, M. K. Tucker, B. E. Holmes and G. L. Heard, J. Phys. Chem. A, 2011, 13133–13138 CrossRef CAS PubMed.
- J. Liu, Y. Liu, N. Y. Liu, Y. Z. Han, X. Zhang, H. Huang, Y. Lifshitz, S.-T. Lee, J. Zhong and Z. H. Kang, Science, 2015, 970–974 CrossRef CAS PubMed.
Footnotes |
† Electronic supplementary information (ESI) available. See DOI: 10.1039/c5ra20403h |
‡ These authors contributed equally to this work. |
|
This journal is © The Royal Society of Chemistry 2016 |