DOI:
10.1039/C6QO00434B
(Research Article)
Org. Chem. Front., 2016,
3, 1493-1497
Direct vicinal difunctionalization of alkynes through trifluoromethylation and aminosulfonylation via insertion of sulfur dioxide under catalyst-free conditions†
Received
4th August 2016
, Accepted 29th August 2016
First published on 30th August 2016
Abstract
Direct vicinal difunctionalization of alkynes through a catalyst-free four-component reaction of Togni's reagent, alkynes, sulfur dioxide, and hydrazines at room temperature is developed, which generates (E)-3,3,3-trifluoroprop-1-ene-1-sulfonohydrazides in good yields. This one-pot transformation works efficiently under extremely mild conditions without any catalysts and additives. The reaction process proceeds through trifluoromethylation of alkynes and aminosulfonylation via insertion of sulfur dioxide with excellent stereoselectivity and regioselectivity. Additionally, this reaction shows a broad substrate scope with good functional group tolerance. A plausible mechanism is proposed, supported by DFT calculations.
The importance of fluorinated molecules has been recognized due to their wide existence in pharmaceuticals and materials.1 In the past decade, continuous approaches have been proposed for the construction of fluorinated skeletons.2,3 It is demonstrated that the incorporation of a CF3 group into small molecules would greatly improve their potential biological activities such as lipophilicity, bioavailability and metabolic stability.2 Thus, introduction of the trifluoromethyl group into small molecules has attracted particular interest.3 In spite of various protocols for fluorination, the radical process for access to fluoro-containing molecules is more and more attractive with the advantages of high reactivity, selectivity and efficiency under mild conditions. Recently, much attention has been paid to the direct vicinal difunctionalization of alkynes.4–6 For example, Cho's group reported the construction of trifluoromethylated alkenyl iodides from alkynes and CF3I by using photoredox catalysis under visible light.5a In particular, this approach provides an efficient route for the generation of highly functionalized olefins and for the construction of complex molecules, through the concomitant incorporation of a fluorinated moiety and a functional group.
In the past few years, advances in the preparation of sulfonyl compounds via insertion of sulfur dioxide have been witnessed.7–9 Compared with the conventional methods for the generation of sulfonyl compounds,9 the advantages of insertion of sulfur dioxide into organic molecules are obvious. So far, the procedures in transition metal catalysis or under metal-free conditions have been developed, avoiding the use of sulfur dioxide in the form of a toxic gas. The source of sulfur dioxide includes DABCO-bis (sulfur dioxide) (named: DABSO) and inorganic sulfite. As part of a program for the synthesis of sulfonyl compounds via insertion of sulfur dioxide in our laboratory,8 we envisioned that direct vicinal difunctionalization of alkynes through trifluoromethylation and aminosulfonylation would provide an efficient pathway for the formation of highly functionalized olefins. A proposed synthetic route is presented in Scheme 1. We hypothesized that a tandem radical process involving trifluoromethylation of alkynes and sulfonylation via insertion of sulfur dioxide would be feasible, providing 3,3,3-trifluoroprop-1-ene-1-sulfonyl compounds. We anticipated that highly functionalized olefins would be generated via this strategy. Prompted by the development of radical trifluoromethylation and insertion of sulfur dioxide, we therefore, initiated a program to explore the direct vicinal difunctionalization of alkynes through a four-component reaction of Togni's reagent, alkynes, sulfur dioxide, and hydrazines.
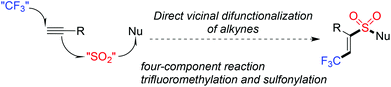 |
| Scheme 1 A proposed direct vicinal difunctionalization of alkynes through a four-component reaction of Togni's reagent, alkynes, sulfur dioxide, and hydrazines. | |
At the outset, a four-component reaction of Togni's reagent, 1-ethynyl-4-methylbenzene 1a, sulfur dioxide, and morpholin-4-amine 2a was selected as the model for reaction development (Table 1). The reaction was initially performed at 35 °C in acetonitrile. The experimental operation is simple: 1-ethynyl-4-methylbenzene 1a (0.2 mmol) was combined with Togni's reagent (0.24 mmol) and DABSO (0.16 mmol) in a tube. The tube was evacuated and backfilled with N2 three times before the addition of CH3CN (4.0 mL). Subsequently, morpholin-4-amine 2a (1.5 equiv.) was added to the solution. The mixture was stirred for half an hour until the completion of the reaction. To our delight, we observed the formation of the expected product 3a, with a 45% isolated yield (Table 1, entry 1). The structure of compound 3a was identified by X-ray crystallography analysis (see the ESI†). From this result, it seemed that the reaction proceeded through trifluoromethylation of alkyne and aminosulfonylation via the insertion of sulfur dioxide with excellent stereoselectivity and regioselectivity. Interestingly, the yield was increased to 69% when morpholin-4-amine 2a was added dropwise into the solution. Other solvents were then examined, however, no better results were obtained (Table 1, entries 3–7). The result was inferior when the reaction temperature was higher (Table 1, entries 8 and 9). The corresponding (E)-3,3,3-trifluoroprop-1-ene-1-sulfonohydrazide 3a was obtained in 71% yield when the reaction occurred at 25 °C (Table 1, entry 10). The yield was lower when the amount of morpholin-4-amine 2a was increased to 2.0 equivalents (Table 1, entry 11). Gratifyingly, the desired product could be afforded in 81% yield in the presence of 1.5 equivalents of Togni's reagent (Table 1, entry 12). It is noteworthy that the reaction proceeds under catalyst-free conditions. Moreover, no additive is necessary in the transformation. We envisioned that the advantages of this transformation including high efficiency, a metal free process, excellent stereoselectivity and regioselectivity, and mild conditions would make this approach attractive for further applications.
Table 1 Initial studies for the metal-free four-component reaction of Togni's reagent, alkyne 1a, DABCO·(SO2)2, and morpholin-4-amine 2a
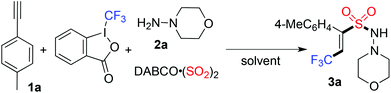
|
Entry |
T (°C) |
Solvent |
Yielda (%) |
Isolated yield based on 1-ethynyl-4-methylbenzene 1a.
Morpholin-4-amine 2a was added dropwise.
In the presence of 2.0 equivalents of morpholin-4-amine 2a.
In the presence of 1.5 equivalents of Togni's reagent.
|
1 |
35 |
CH3CN |
45 |
2b |
35 |
CH3CN |
69 |
3b |
35 |
DCE |
64 |
4b |
35 |
DMF |
39 |
5b |
35 |
DMSO |
39 |
6b |
35 |
THF |
19 |
7b |
35 |
1,4-Dioxane |
46 |
8b |
45 |
CH3CN |
57 |
9b |
55 |
CH3CN |
40 |
10b |
25 |
CH3CN |
71 |
11b,c |
25 |
CH3CN |
57 |
12b,d |
25 |
CH3CN |
81 |
Next, we started to explore the reaction scope of this metal-free four-component reaction of Togni's reagent, alkynes, sulfur dioxide, and hydrazines under the above optimized conditions. As shown in Table 2, all the reactions worked well to give rise to the desired products 3 in moderate to good yields. As expected, excellent stereoselectivity was observed, which afforded (E)-3,3,3-trifluoroprop-1-ene-1-sulfonohydrazides exclusively. Additionally, all reactions went to completion in half an hour at room temperature. For the substrates of 1-ethynylarenes, different functional groups including fluoro, chloro, bromo, methoxy, and thiophenyl were compatible under the standard conditions. For instance, the reaction of Togni's reagent, 3-ethynylthiophene, sulfur dioxide, and morpholin-4-amine 2a provided the corresponding product 3m in 70% yield. It is noteworthy that the reactions of alkyl acetylenes worked efficiently as well. For example, compound 3n was obtained in 47% yield when 3,3-dimethylbut-1-yne was utilized as the substrate. The reactions of other hydrazines were examined subsequently, and the products were generated as expected. Additionally, a desilylated product 3t containing (E)-3,3,3-trifluoroprop-1-ene-1-sulfonamide10 could be obtained with an acceptable yield (Scheme 2).
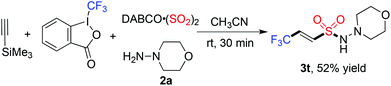 |
| Scheme 2 Reaction of Togni's reagent, trimethylsilylacetylene, DABCO·(SO2)2, and morpholin-4-amine 2a. | |
Table 2 Scope exploration of four-component reaction of Togni's reagent, alkynes, DABCO·(SO2)2, and hydrazines.a
Isolated yield based on alkyne 1.
|
|
In order to understand the mechanism of this catalyst-free four-component reaction, preliminary mechanistic investigation was carried out. 2,2,6,6-Tetramethyl-1-piperidinyloxy (TEMPO), an effective radical scavenger, was added in the reaction as shown in Scheme 3. Only a trace amount of product 3a was detected when 2.5 equivalents of TEMPO was added into the reaction under the standard conditions. Instead, the formation of compound TEMPO-CF34 with 79% yield was observed (Scheme 3, eqn (a)). This result suggested that a
was involved in the reaction as the key intermediate, which was presumably produced from Togni's reagent through single electron transfer (SET).11 To clarify the electron donor in the process of SET, morpholin-4-amine 2a and DABSO were added into the solution of Togni's reagent and TEMPO respectively (Scheme 3, eqn (b) and (c)). Interestingly, sulfur dioxide could also provide an electron to Togni's reagent, but the presence of morpholin-4-amine 2a was more effective. However, compound 4 could not be detected when Togni's reagent was combined with alkyne or DABCO in the presence of TEMPO (see the ESI†). According to these experimental observations, a plausible mechanism for the catalyst-free four-component reaction of Togni's reagent, alkynes, sulfur dioxide, and hydrazines was proposed, as shown in Scheme 4.
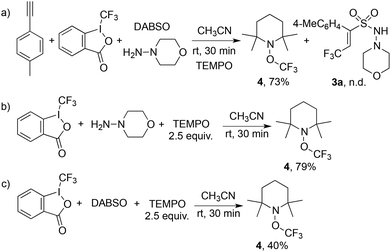 |
| Scheme 3 Mechanistic studies for the four-component reaction of Togni's reagent, alkynes, DABCO·(SO2)2, and hydrazines. | |
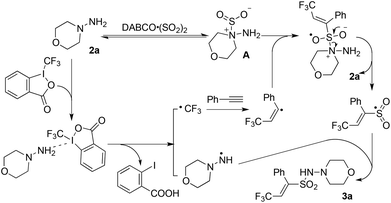 |
| Scheme 4 A possible mechanism for the catalyst-free four-component reaction of Togni's reagent, alkynes, DABCO·(SO2)2, and hydrazines. | |
In order to gain deeper insights into the reaction mechanism especially on how the
radical is given out and where the SO2 part in the product 3 is from, M06-2X density functional theory (DFT) calculations were performed with the Gaussian09 software package.12 A possible pathway was proposed and the corresponding free energy reaction profile is shown in Fig. 1. First, reactant 2 and Togni's reagent B can form complex 2B-COM, which is 9.5 kcal mol−1 more stable than the reactants. One of the two hydrogen atoms in the amino group forms a strong hydrogen bond with the saturated oxygen atom of Togni's reagent, and the distance between the H atom and the O atom is 2.13 Å. And the N–I distance is 3.01 Å. Then B is reduced via single-electron transfer (SET)13 and gives out C, free radical D and
as well. It can be inferred that this step is rate-determining. Subsequently,
attacks alkyne 1 to afford free radical F. Then formation of intermediate G occurs smoothly viaTS2 from the interaction of free radical F and hydrazine-SO2 complex A.8a The breaking of the S–N bond in G leads to free radical H. Finally, H combines with radical D to produce complex I first and then form product 3. Another pathway affording
from hydrazine-SO2A and B was also studied (Fig. S1, see the ESI†). Obviously, the step giving the
radical is more difficult than that in the former pathway, and this step is rate-determining too. So this path is less favoured, which is in good agreement with the experimental fact that hydrazine 2 is a good reagent producing the
radical, and the yield of CF3-TEMPO is high up to 79%. It is also worth noting in Fig. S1† that the free energy profile is bifurcated from radical F, with the corresponding two modes producing the H radical. In one branch SO2 comes from dissociation of AB-COM (in black), and in the other one from the second molecule A (in red). The profile shows that the former is more favoured.
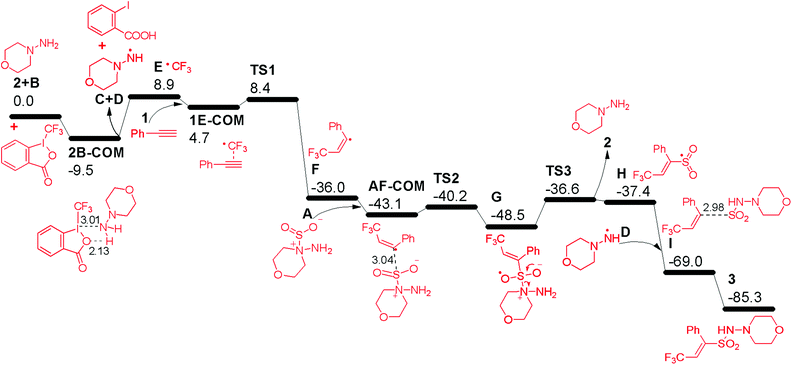 |
| Fig. 1 Free energy reaction profile (kcal mol−1) in acetonitrile from reactants 1, 2, A and B to product 3, calculated at the M06-2X/6-31+G(d,p) with LANL2DZ (for I) level. | |
Conclusions
In conclusion, we have described a direct vicinal difunctionalization of alkynes through a metal-free four-component reaction of Togni's reagent, alkynes, sulfur dioxide, and hydrazines, providing (E)-3,3,3-trifluoroprop-1-ene-1-sulfonohydrazides in good yields at room temperature. This one-pot transformation works efficiently under extremely mild conditions without any catalysts. Moreover, no additive is necessary in this transformation. During the reaction process, it proceeds through trifluoromethylation of alkynes and aminosulfonylation via insertion of sulfur dioxide with excellent stereoselectivity and regioselectivity. Additionally, this reaction shows a broad substrate scope with good functional group tolerance. A plausible mechanism is proposed, supported by DFT calculations. We believe that the advantages of this transformation including high efficiency, a metal-free process, excellent stereoselectivity and regioselectivity, simple experimental operation, and mild conditions will make this approach attractive for further applications.
Acknowledgements
Financial support from the National Natural Science Foundation of China (No. 21372046, 21532001) is gratefully acknowledged.
Notes and references
- For selected examples, see:
(a) J. Wang, M. Sánchez-Roselló, J. L. Aceña, C. DelPozo, A. E. Sorochinsky, S. Fustero, V. A. Soloshonok and H. Liu, Chem. Rev., 2013, 114, 2432 CrossRef PubMed;
(b) T. Liang, C. N. Neumann and T. Ritter, Angew. Chem., Int. Ed., 2013, 52, 8214 CrossRef CAS PubMed;
(c) C. Hollingworth and V. Gouverneur, Chem. Commun., 2012, 48, 929 RSC;
(d) O. A. Tomashenko and V. V. Grushin, Chem. Rev., 2011, 111, 4475 CrossRef CAS PubMed;
(e) J. Hu, W. Zhang and F. Wang, Chem. Commun., 2009, 7465 RSC;
(f) K. Müller, C. Faeh and F. Diederich, Science, 2007, 317, 1881 CrossRef PubMed;
(g) J.-A. Ma and D. Cahard, Chem. Rev., 2004, 104, 6119 CrossRef CAS PubMed;
(h)
Organofluorine Chemistry: Principles and Commercial Applications, ed. R. E. Banks, B. E. Smart and J. C. Tatlow, Plenum Press, New York, 1994 Search PubMed;
(i)
Fluorine in Bioorganic Chemistry, ed. J. T. Welch and S. Eswarakrishman, Wiley, New York, 1991 Search PubMed.
- For selected examples, see:
(a) C. Ni, M. Hu and J. Hu, Chem. Rev., 2015, 115, 765 CrossRef CAS PubMed;
(b) X. Liu, C. Xu, M. Wang and Q. Liu, Chem. Rev., 2015, 115, 683 CrossRef CAS PubMed;
(c) C. Alonso, E. M. Marigorta, G. Rubiales and F. Palacios, Chem. Rev., 2015, 115, 1847 CrossRef CAS PubMed;
(d) E. Merino and C. Nevado, Chem. Soc. Rev., 2014, 43, 6598 RSC;
(e) P. Yu, J.-S. Lin, L. Li, S.-C. Zheng, Y.-P. Xiong, L.-J. Zhao, B. Tan and X.-Y. Liu, Angew. Chem., Int. Ed., 2014, 53, 11890 CrossRef CAS PubMed;
(f) C. Yu, N. Iqbal, S. Park and E. J. Cho, Chem. Commun., 2014, 50, 12884 RSC;
(g) X.-L. Jiang, Z.-H. Chen, X.-H. Xu and F.-L. Qing, Org. Chem. Front., 2014, 1, 774 RSC;
(h) C. Matheis, K. Jouvin and L. J. Goossen, Org. Lett., 2014, 16, 5984 CrossRef CAS PubMed;
(i) D. J. Wilger, N. J. Gesmundo and D. A. Nicewicz, Chem. Sci., 2013, 4, 3160 RSC;
(j) G. Landelle, A. Panossian, S. Pazenok, J.-P. Vors and F. R. Leroux, Beilstein J. Org. Chem., 2013, 9, 2476 CrossRef PubMed;
(k) T. Xu and G. Liu, Org. Lett., 2012, 14, 5416 CrossRef CAS PubMed;
(l) X. Yang, T. Wu, R. J. Hipps and F. D. Toste, Chem. Rev., 2015, 115, 826 CrossRef CAS PubMed;
(m) X.-H. Xu, K. Matsuzaki and N. Shibata, Chem. Rev., 2015, 115, 731 CrossRef CAS PubMed;
(n) L. Chu and F.-L. Qing, Acc. Chem. Res., 2014, 47, 1513 CrossRef CAS PubMed;
(o) T. H. Rehm, Chem. Eng. Technol., 2016, 39, 66 CrossRef;
(p) Y. Zeng, C. Ni and J. Hu, Chem. – Eur. J., 2016, 22, 3210 CrossRef CAS PubMed;
(q) X.-H. Xu and F.-L. Qing, Curr. Org. Chem., 2015, 19, 1566 CrossRef CAS;
(r) Y. Lu, C. Liu and Q. Chen, Curr. Org. Chem., 2015, 19, 1638 CrossRef CAS;
(s) G. K. S. Prakash and J. Hu, Acc. Chem. Res., 2007, 40, 921 CrossRef CAS PubMed;
(t) C. Ni and J. Hu, Synthesis, 2014, 842 Search PubMed;
(u) X. Shen and J. Hu, Eur. J. Org. Chem., 2014, 4437 CrossRef CAS.
- For selected examples, see:
(a) T. Besset, T. Poisson and X. Pannecoucke, Chem. – Eur. J., 2014, 20, 16830 CrossRef CAS PubMed;
(b) H. Egami and M. Sodeoka, Angew. Chem., Int. Ed., 2014, 53, 8294 CrossRef CAS PubMed;
(c) T. Nishida, H. Ida, Y. Kuninobu and M. Kanai, Nat. Commun., 2014, 5, 3387 Search PubMed;
(d) H. Liu, Z. Gu and X. Jiang, Adv. Synth. Catal., 2013, 355, 617 CrossRef CAS;
(e) P. Chen and G. Liu, Synthesis, 2013, 2919 CAS;
(f) T. Liu, X. Shao, Y. Wu and Q. Shen, Angew. Chem., Int. Ed., 2012, 51, 540 CrossRef CAS PubMed;
(g) Y. Ye and M. S. Sanford, J. Am. Chem. Soc., 2012, 134, 9034 CrossRef CAS PubMed;
(h) J. Xu, Y. Fu, D.-F. Luo, Y.-Y. Jiang, B. Xiao, Z.-J. Liu, T.-J. Gong and L. Liu, J. Am. Chem. Soc., 2011, 133, 15300 CrossRef CAS PubMed;
(i) T. Furuya, A. S. Kamlet and T. Ritter, Nature, 2011, 473, 470 CrossRef CAS PubMed;
(j) J. Nie, H.-C. Guo, D. Cahard and J.-A. Ma, Chem. Rev., 2011, 111, 455 CrossRef CAS PubMed.
- For selected examples, see:
(a) P. Gao, X.-R. Song, X.-Y. Liu and Y.-M. Liang, Chem. – Eur. J., 2015, 21, 7648 CrossRef CAS PubMed;
(b) T. Besset, T. Poisson and X. Pannecoucke, Eur. J. Org. Chem., 2015, 2765 CrossRef CAS;
(c) S.-M. Wang, J.-B. Han, C.-P. Zhang, H.-L. Qin and J.-C. Xiao, Tetrahedron, 2015, 71, 7949 CrossRef CAS;
(d) X.-F. Wu, H. Neumann and M. Beller, Chem. – Asian J., 2012, 7, 1744 CrossRef CAS PubMed.
- For selected examples, see:
(a) N. Iqbal, J. Jung, S. Park and E. J. Cho, Angew. Chem., Int. Ed., 2014, 53, 539 CrossRef CAS PubMed;
(b) Z. Hang, Z. Li and Z.-Q. Liu, Org. Lett., 2014, 16, 3648 CrossRef CAS PubMed;
(c) T. Xu, C. W. Cheung and X. Hu, Angew. Chem., Int. Ed., 2014, 53, 4910 CrossRef CAS PubMed;
(d) P. G. Janson, I. Ghoneim, N. O. Ilchenko and K. J. Szabó, Org. Lett., 2012, 14, 2882 CrossRef CAS PubMed;
(e) R. Tomita, T. Koike and M. Akita, Angew. Chem., Int. Ed., 2015, 54, 12923 CrossRef CAS PubMed;
(f) Z. Li, A. García-Domínguez and C. Nevado, J. Am. Chem. Soc., 2015, 137, 11610 CrossRef CAS PubMed;
(g) S. Mizuta, S. Verhoog, K. M. Engle, T. Khotavivattana, M. O'Duill, K. Wheelhouse, G. Rassias, M. Médebielle and V. Gouverneur, J. Am. Chem. Soc., 2013, 135, 2505 CrossRef CAS PubMed;
(h) S. P. Pitre, C. D. McTiernan, H. Ismaili and J. C. Scaiano, ACS Catal., 2014, 4, 2530 CrossRef CAS;
(i) J. Jacquet, S. Blanchard, E. Derat, M. D. Murr and L. Fensterbank, Chem. Sci., 2016, 7, 2030 RSC;
(j) Y.-L. Ji, J.-J. Luo, J.-H. Lin, J.-C. Xiao and Y.-C. Gu, Org. Lett., 2016, 18, 1000 CrossRef CAS PubMed.
- For selected examples, see:
(a) Y. Wang, M. Jiang and J.-T. Liu, Chem. – Eur. J., 2014, 20, 15315 CrossRef CAS PubMed;
(b) Y. Wang, M. Jiang and J.-T. Liu, Org. Chem. Front., 2015, 2, 542 RSC;
(c) G.-C. Ge, X.-J. Huang, C.-H. Ding, S.-L. Wan, L.-X. Dai and X.-L. Hou, Chem. Commun., 2014, 50, 3048 RSC;
(d) Y. Li, Y. Lu, G. Qiu and Q. Ding, Org. Lett., 2014, 16, 4240 CrossRef CAS PubMed;
(e) Y.-L. Ji, J.-H. Lin, J.-C. Xiao and Y.-C. Gu, Org. Chem. Front., 2014, 1, 1280 RSC;
(f) J. Xu, Y.-L. Wang, T.-J. Gong, B. Xiao and Y. Fu, Chem. Commun., 2014, 50, 12915 RSC;
(g) H.-L. Hua, Y.-T. He, Y.-F. Qiu, Y.-X. Li, B. Song, P. Gao, X.-R. Song, D.-H. Guo, X.-Y. Liu and Y.-M. Liang, Chem. – Eur. J., 2015, 21, 1468 CrossRef CAS PubMed;
(h) W. Fu, M. Zhu, G. Zou, C. Xu, Z. Wang and B. Ji, J. Org. Chem., 2015, 80, 4766 CrossRef CAS PubMed;
(i) P. Gao, Y.-W. Shen, R. Fang, X.-H. Hao, Z.-H. Qiu, F. Yang, X.-B. Yan, Q. Wang, X.-J. Gong, X.-Y. Liu and Y.-M. Liang, Angew. Chem., Int. Ed., 2014, 53, 7629 CrossRef CAS PubMed;
(j) Y.-P. Xiong, M.-Y. Wu, X.-Y. Zhang, C.-L. Ma, L. Huang, L.-J. Zhao, B. Tan and X.-Y. Liu, Org. Lett., 2014, 16, 1000 CrossRef CAS PubMed.
-
(a) B. Nguyen, E. J. Emmet and M. C. Willis, J. Am. Chem. Soc., 2010, 132, 16372 CrossRef CAS PubMed;
(b) A. J. Emmet, C. S. Richards-Taylor, B. Nguyen, A. B. Garcia-Rubia, R. Hayter and M. C. Willis, Org. Biomol. Chem., 2012, 10, 4007 RSC;
(c) E. J. Emmett, B. R. Hayter and M. C. Willis, Angew. Chem., Int. Ed., 2013, 52, 12679 CrossRef CAS PubMed;
(d) E. J. Emmett, B. R. Hayter and M. C. Willis, Angew. Chem., Int. Ed., 2014, 53, 10204 CrossRef CAS PubMed;
(e) A. S. Deeming, C. J. Rusell, A. J. Hennessy and M. C. Willis, Org. Lett., 2014, 16, 150 CrossRef CAS PubMed;
(f) B. N. Rocke, K. B. Bahnck, M. Herr, S. Lavergne, V. Mascitti, C. Perreault, J. Polivkova and A. Shavnya, Org. Lett., 2014, 16, 154 CrossRef CAS PubMed;
(g) M. W. Johnson, S. W. Bagley, N. P. Mankad, R. G. Bergman, V. Mascitti and F. D. Toste, Angew. Chem., Int. Ed., 2014, 53, 4404 CrossRef CAS PubMed;
(h) P. Bisseret and N. Blanchard, Org. Biomol. Chem., 2013, 11, 5393 RSC;
(i) L. Martial, Synlett, 2013, 1595 Search PubMed;
(j) W. Li, H. Li, P. Langer, M. Beller and X.-F. Wu, Eur. J. Org. Chem., 2014, 3101 CrossRef;
(k) W. Li, M. Beller and X.-F. Wu, Chem. Commun., 2014, 50, 9513 RSC;
(l) X. Wang, L. Xue and Z. Wang, Org. Lett., 2014, 16, 4056 CrossRef CAS PubMed;
(m) A. S. Deeming, C. J. Russell and M. C. Willis, Angew. Chem., Int. Ed., 2015, 54, 1168 CrossRef CAS PubMed.
-
(a) D. Zheng, Y. An, Z. Li and J. Wu, Angew. Chem., Int. Ed., 2014, 53, 2451 CrossRef CAS PubMed;
(b) S. Ye and J. Wu, Chem. Commun., 2012, 48, 10037 RSC;
(c) S. Ye and J. Wu, Chem. Commun., 2012, 48, 7753 RSC;
(d) S. Ye, H. Wang, Q. Xiao, Q. Ding and J. Wu, Adv. Synth. Catal., 2014, 356, 3225 CrossRef CAS;
(e) Y. An, D. Zheng and J. Wu, Chem. Commun., 2014, 50, 11746 RSC;
(f) Y. Luo, X. Pan, C. Chen, L. Yao and J. Wu, Chem. Commun., 2015, 51, 180 RSC;
(g) D. Zheng, Y. Li, Y. An and J. Wu, Chem. Commun., 2014, 50, 8886 RSC;
(h) Y. Li, D. Zheng, Z. Li and J. Wu, Org. Chem. Front., 2016, 3, 574 RSC;
(i) R. Mao, D. Zheng, H. Xia and J. Wu, Org. Chem. Front., 2016, 3, 693 RSC;
(j) D. Zheng, R. Mao, Z. Li and J. Wu, Org. Chem. Front., 2016, 3, 359 RSC;
(k) K. Zhou, H. Xia and J. Wu, Org. Chem. Front., 2016, 3, 865 RSC;
(l) D. Zheng, Y. Kuang and J. Wu, Org. Biomol. Chem., 2015, 13, 10370 RSC.
-
(a) G. Liu, C. Fan and J. Wu, Org. Biomol. Chem., 2015, 13, 1592 RSC;
(b) C. S. Richards-Taylor, D. C. Blakemore and M. C. Willis, Chem. Sci., 2014, 5, 222 RSC;
(c) W. E. Truce and A. M. Murphy, Chem. Rev., 1951, 48, 69 CrossRef CAS PubMed;
(d) A. Shavnya, K. D. Hesp, V. Mascitti and A. C. Smith, Angew. Chem., Int. Ed., 2015, 54, 13571 CrossRef CAS PubMed;
(e) A. S. Deeming, C. J. Russell and M. C. Willis, Angew. Chem., Int. Ed., 2016, 55, 747 CrossRef CAS PubMed;
(f) C. C. Chen and J. Waser, Org. Lett., 2015, 17, 736 CrossRef CAS PubMed;
(g) A. Shavnya, S. B. Coffey, A. C. Smith and V. Mascitti, Org. Lett., 2013, 15, 6226 CrossRef CAS PubMed;
(h) A. S. Tsai, J. M. Curto, B. N. Rocke, A. R. Dechert-Schmitt, G. K. Ingle and V. Mascitti, Org. Lett., 2016, 18, 508 CrossRef CAS PubMed;
(i) W. Zhang and M. Luo, Chem. Commun., 2016, 52, 2980 RSC.
-
B. Michael, E. Marko and K. Christopher, PCT Int. Appl, 2013144179, 2013 Search PubMed.
- For selected examples, see:
(a) P. Yu, S.-C. Zheng, N.-Y. Yang, B. Tan and X.-Y. Liu, Angew. Chem., Int. Ed., 2015, 54, 4041 CrossRef CAS PubMed;
(b) N.-Y. Yang, Z.-L. Li, L. Ye, B. Tan and X.-Y. Liu, Chem. Commun., 2016, 52, 9052 RSC;
(c) Y. Cheng and S. Yu, Org. Lett., 2016, 18, 2962 CrossRef CAS PubMed.
- For computational details and references, see the ESI.†.
- L. Ling, K. Liu, X. Li and Y. Li, ACS Catal., 2015, 5, 2458 CrossRef CAS.
Footnote |
† Electronic supplementary information (ESI) available: Experimental procedure, characterization data, 1H & 13C NMR spectra of compounds 3. Compound 3a. CCDC 1485659. For ESI and crystallographic data in CIF or other electronic format see DOI: 10.1039/c6qo00434b |
|
This journal is © the Partner Organisations 2016 |
Click here to see how this site uses Cookies. View our privacy policy here.