DOI:
10.1039/C6QO00257A
(Research Article)
Org. Chem. Front., 2016,
3, 1250-1253
Switching between intermolecular and intramolecular reactions using flow microreactors: lithiation of 2-bromo-2′-silylbiphenyls†
Received
9th June 2016
, Accepted 25th July 2016
First published on 26th July 2016
Abstract
Reaction-pathway control of 2-lithio-2′-silylbiphenyls was accomplished in flow microreactor systems. Effective switching between the intermolecular reaction with an electrophile and the intramolecular reaction was performed at will by choosing an appropriate residence time and temperature region.
Introduction
Recently, selective formation of different compounds from one set of starting materials and/or intermediates has attracted significant interest in organic chemistry.1 If one set of starting materials and/or intermediates results in more than one compound in a selective manner by simply changing reaction conditions, such a method would serve as a powerful tool in organic synthesis. Herein we report that the switching between intermolecular and intramolecular reactions can be accomplished based on residence time and temperature control in flow microreactor systems.
A great number of chemical syntheses with flow microreactor systems2–4 have been developed and utilized in various fields, in both academia and industry. Recent investigations revealed significant features of flow microreactor systems, greatly improving the selectivity of chemical reactions: fast mixing due to short diffusion paths and fast heat transfer by virtue of a high surface-to-volume ratio. In addition, short residence time in a micro channel is beneficial for controlling highly reactive intermediates. Various chemical reactions for organic synthesis have been developed by taking advantage of the aforementioned features of flow microreactor systems.5 In addition, several reactions can be integrated by the use of flow microreactors and hence, the usefulness and power of organic synthesis have been greatly enhanced.6 Recently, we have reported that the generation of highly reactive aryllithiums based on a halogen–lithium exchange of aryl halides followed by reactions with electrophiles could be conducted in flow microreactor systems.7 We have also studied generation and reactions of 2-lithio-2′-silylbiphenyls, important intermediates for the synthesis of siloles.8,9 During the course of our studies on the halogen–lithium exchange of 2-bromo-2′-(trimethylsilyl)biphenyl using flow microreactor systems, we observed that the resulting 2-lithio-2′-(trimethylsilyl)biphenyl underwent the competitive intermolecular and intramolecular reactions shown in Fig. 1. Thus, we studied the selectivity in detail by changing the residence time and the temperature.
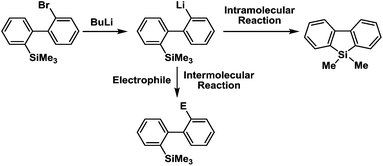 |
| Fig. 1 Intermolecular and intramolecular reactions of 2-lithio-2′-(trimethylsilyl)biphenyl generated by the halogen–lithium exchange of 2-bromo-2′-(trimethylsilyl)biphenyl. | |
Results and discussion
First, the Br–Li exchange of 2-bromo-2′-(trimethylsilyl)biphenyl followed by trapping with methanol was examined using a flow microreactor system consisting of two T-shaped micromixers (M1 and M2) and two microtube reactors (R1 and R2) shown in Fig. 2 with various residence times (tR1) in R1, and temperatures (T).
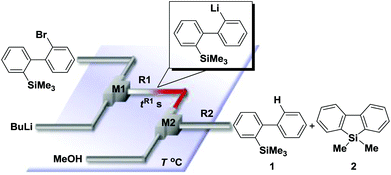 |
| Fig. 2 A flow microreactor system for the Br–Li exchange of 2-bromo-2′-(trimethylsilyl)biphenyl with n-BuLi (1.05 eq.) and the subsequent reaction with methanol. T-Shaped micromixers: M1 and M2, microtube reactors: R1 and R2. | |
The results are summarized in Fig. 3, in which the yields of 2-bromo-2′-(trimethylsilyl)biphenyl, 2-(trimethylsilyl)biphenyl (1), and 9,9-dimethyl-9-silafluorene (2) are plotted against T and tR1 as a contour map with scattered overlay. In Fig. 3a, the recovery of the starting material, 2-bromo-2′-(trimethylsilyl)biphenyl is plotted against the temperature (T) and the residence time in R1 (tR1) as a contour map with scattered overlay. In a low temperature (T < −60 °C) – short residence time (tR1 < 3.14 s) region, the starting material remained unchanged to some extent because the bromine–lithium exchange is slow at low temperatures. In Fig. 3b, the yields of the intermolecular trapping product, 2-(trimethylsilyl)biphenyl (1) is plotted against T and tR1. High yields were obtained with shorter residence times at low temperatures (such as tR1 < 0.79 s, T < −40 °C). The increase in T caused the decrease in the yield presumably because the intramolecular reaction of the 2-lithio-2′-(trimethylsilyl)biphenyl intermediate with the trimethylsilyl group took place. In fact, at higher temperatures (T > 0 °C), the product derived from the intramolecular reaction, 9,9-dimethyl-9-silafluorene (2) was obtained in higher yields (Fig. 3c).
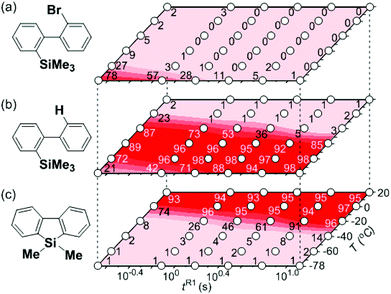 |
| Fig. 3 Temperature-residence time (in R1) map for the halogen–lithium exchange reaction of 2-bromo-2′-(trimethylsilyl)biphenyl with n-BuLi followed by the reaction with methanol: (a) a contour map with scatter overlay of the recovery of 2-bromo-2′-(trimethylsilyl)biphenyl, (b) a contour map with scatter overlay of the yield of 2-(trimethylsilyl)biphenyl (1), and (c) a contour map with scatter overlay of the yield of 9,9-dimethyl-9-silafluorene (2). | |
Thus, the reaction pathways could be controlled by choosing an appropriate temperature-residence time region. For example, 2-(trimethylsilyl)biphenyl (1), the product derived from the intermolecular trapping of 2-lithio-2′-(trimethylsilyl)biphenyl was obtained in 96% yield at tR1 = 0.79 s and T = −40 °C suppressing the intramolecular reaction. In contrast, 9,9-dimethyl-9-silafluorene (2), the product derived from the intramolecular reaction was obtained in 94% yield at tR1 = 0.79 s and T = 20 °C.
Under the optimized conditions (T = −40 °C, tR1 = 0.79 s) for the intermolecular trapping, the reactions with methyl trifluoromethanesulfonate and isopropyl pinacol borate were successfully carried out to obtain the corresponding products in good yields (Table 1). Moreover, the generation and reactions of other 2-lithio-2′-silylbiphenyl such as 2-lithio-2′-(dimethylphenylsilyl)biphenyl and 2-lithio-2′-(methyldiphenylsilyl)biphenyl were successfully achieved to selectively obtain the intermolecular trapping products or intramolecular reaction products in good yields at will by choosing an appropriate temperature-residence time region.
Table 1 Generations and reactions of 2-lithio-2′-silylbiphenyl
Substrate |
T (°C) |
t
R1 (s) |
Electrophile |
Product |
Yield (%) |
|
20 |
0.79 |
MeOH |
|
94 |
|
−40 |
0.79 |
MeOH |
|
96 |
|
−40 |
0.79 |
MeOTf |
|
94 |
|
−40 |
0.79 |
PinBOiPr |
|
81 |
|
20 |
12.9 |
MeOH |
|
92 |
|
−78 |
6.28 |
MeOH |
|
91 |
|
20 |
3.14 |
MeOH |
|
96 |
|
−60 |
12.6 |
MeOH |
|
100 |
We have already reported that monolithiation of dibromobiphenyl can be successfully conducted using a flow microreactor system, although the reaction results in a significant amount of dilithiated product in a conventional macro batch system.10 Thus, we integrated two sets of Br–Li exchange reactions starting from 2,2′-dibromobiphenyl. For this purpose, we used an integrated flow microreactor system consisting of four micromixers (M1, M2, M3, and M4) and four microtube reactors (R1, R2, R3, and R4) shown in Fig. 4. 2-Lithio-2′-bromobiphenyl was selectively generated in M1 and R1 at 0 °C (tR1 = 0.39 s). The reaction with the trimethylsilyl chloride was carried out in M2 and R2 at the same temperature (tR2 = 15 s). Then, the second Br–Li exchange with n-BuLi in M3 and R3, followed by reaction with isopropyl pinacol borate in M4 and R4, were carried out by using an appropriate condition (−40 °C, tR3 = 0.53 s) to obtain the corresponding products derived from intermolecular trapping with isopropyl pinacol borate in 71% yield.
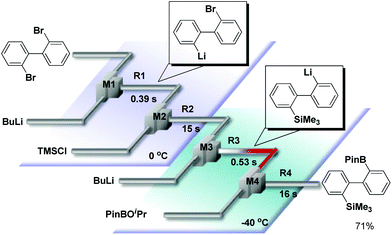 |
| Fig. 4 An integrated flow microreactor system for two sets of Br–Li exchange reaction starting from 2,2′-dibromobiphenyl. T-Shaped micromixers: M1, M2, M3, and M4, microtube reactors: R1, R2, R3, and R4. | |
Conclusions
In conclusion, the present reactions serve as a showcase of effective switching of the reaction pathways by residence time – temperature control of flow microreactor systems.11 Either the intermolecular reaction of lithio-2′-silylbiphenyls with subsequently added electrophiles or their intramolecular reaction with the silyl group was achieved at will by choosing appropriate reaction conditions. The method serves as a nice tool for synthesizing 2′-substituted 2-silylbiphenyls and 9-silafluorenes, which may provide useful intermediates for functional materials.
Acknowledgements
This work was partially supported by a Grant-in-Aid for Scientific Research (S) (number 26220804), Scientific Research (S) (number 25220913), Scientific Research (B) (number 26288049), and Scientific Research on Innovative Areas 2707 Middle molecular strategy from MEXT (no. 15H05849).
Notes and references
-
(a) M. M. Midland and R. L. Halterman, J. Org. Chem., 1981, 46, 1227 CrossRef CAS;
(b) A. J. Laurent and S. Leniak, Tetrahedron Lett., 1992, 33, 3311 CrossRef CAS;
(c) N. Dyatkina, B. Costisella, F. Theil and M. von Janta-Lipinski, Tetrahedron Lett., 1994, 35, 1961 CrossRef CAS;
(d) S. Kobayashi and T. Hayashi, J. Org. Chem., 1995, 60, 1098 CrossRef CAS;
(e) K. Hiroya, N. Suzuki, A. Yasuhara, Y. Egawa, A. Kasano and T. Sakamoto, J. Chem. Soc., Perkin Trans. 1, 2000, 4339 RSC;
(f) C. Feng, X. Wang, B.-Q. Wang, K.-Q. Zhao, P. Hu and Z. J. Shi, Chem. Commun., 2012, 48, 356 RSC.
- Books on flow microreactor synthesis:
(a)
W. Ehrfeld, V. Hessel and H. Löwe, Microreactors, Wiley-VCH, Weinheim, Germany, 2000 CrossRef;
(b)
V. Hessel, S. Hardt and H. Löwe, Chemical Micro Process Engineering, Wiely-VCH, Weinheim, Germany, 2004 Search PubMed;
(c)
V. Hessel, A. Renken, J. C. Schouten and J. Yoshida, Micro Process Engineering, Wiley-Blackwell, Chichester, United Kingdom, 2009 Search PubMed;
(d)
T. Wirth, Microreactors in Organic Chemistry and Catalysis, Wiley, New York, United States, 2013 Search PubMed.
- Reviews on flow microreactor synthesis:
(a) K. Jähnisch, V. Hessel, H. Löwe and M. Baerns, Angew. Chem., Int. Ed., 2004, 43, 406 CrossRef PubMed;
(b) G. N. Doku, W. Verboom, D. N. Reinhoudt and A. Van den Berg, Tetrahedron, 2005, 61, 2733 CrossRef CAS;
(c) J. Yoshida, A. Nagaki, T. Iwasaki and S. Suga, Chem. Eng. Technol., 2005, 28, 259 CrossRef CAS;
(d) P. Watts and S. J. Haswell, Chem. Soc. Rev., 2005, 34, 235 RSC;
(e) K. Geyer, J. D. C. Codée and P. H. Seeberger, Chem. – Eur. J., 2006, 12, 8434 CrossRef CAS PubMed;
(f) A. J. DeMello, Nature, 2006, 442, 394 CrossRef CAS PubMed;
(g) H. Song, D. L. Chen and R. F. Ismagilov, Angew. Chem., Int. Ed., 2006, 45, 7336 CrossRef CAS PubMed;
(h) J. Kobayashi, Y. Mori and S. Kobayashi, Chem. – Asian J., 2006, 1, 22 CrossRef CAS PubMed;
(i) M. Brivio, W. Verboom and D. N. Reinhoudt, Lab Chip, 2006, 6, 329 RSC;
(j) B. P. Mason, K. E. Price, J. L. Steinbacher, A. R. Bogdan and D. T. McQuade, Chem. Rev., 2007, 107, 2300 CrossRef CAS PubMed;
(k) B. Ahmed-Omer, J. C. Brandt and T. Wirth, Org. Biomol. Chem., 2007, 5, 733 RSC;
(l) P. Watts and C. Wiles, Chem. Commun., 2007, 443 RSC;
(m) T. Fukuyama, M. T. Rahman, M. Sato and I. Ryu, Synlett, 2008, 151 CAS;
(n) R. L. Hartman and K. F. Jensen, Lab Chip, 2009, 9, 2495 RSC;
(o) J. P. McMullen and K. F. Jensen, Annu. Rev. Anal. Chem., 2010, 3, 19 CrossRef CAS PubMed;
(p) J. Yoshida, H. Kim and A. Nagaki, ChemSusChem, 2011, 4, 331 CrossRef CAS PubMed;
(q) C. Wiles and P. Watts, Green Chem., 2012, 14, 38 RSC;
(r) A. Kirschning, L. Kupracz and J. Hartwig, Chem. Lett., 2012, 41, 562 CrossRef CAS;
(s) D. T. McQuade and P. H. Seeberger, J. Org. Chem., 2013, 78, 6384 CrossRef CAS PubMed;
(t) K. S. Elvira, X. C. Solvas, R. C. R. Wootton and A. J. DeMello, Nat. Chem., 2013, 5, 905 CrossRef CAS PubMed;
(u) J. C. Pastre, D. L. Browne and S. V. Ley, Chem. Soc. Rev., 2013, 42, 8849 RSC;
(v) I. R. Baxendale, J. Chem. Technol. Biotechnol., 2013, 88, 519 CrossRef CAS;
(w) J. Yoshida, A. Nagaki and D. Yamada, Drug Discovery Today: Technol., 2013, 10, e53 CrossRef PubMed;
(x) T. Fukuyama, T. Totoki and I. Ryu, Green Chem., 2014, 16, 2042 RSC.
- Some selected recent examples:
(a) D. Cantillo, M. Baghbanzadeh and C. O. Kappe, Angew. Chem., Int. Ed., 2012, 51, 10190 CrossRef CAS PubMed;
(b) W. Shu and S. L. Buchwald, Angew. Chem., Int. Ed., 2012, 51, 5355 CrossRef CAS PubMed;
(c) F. Lévesque and P. H. Seeberger, Angew. Chem., Int. Ed., 2012, 51, 1706 CrossRef PubMed;
(d) K. C. Basavaraju, S. Sharma, R. A. Maurya and D. P. Kim, Angew. Chem., Int. Ed., 2013, 52, 6735 CrossRef CAS PubMed;
(e) C. Brancour, T. Fukuyama, Y. Mukai, T. Skrydstrup and I. Ryu, Org. Lett., 2013, 15, 2794 CrossRef CAS PubMed;
(f) J. D. Nguyen, B. Reiß, C. Dai and C. R. J. Stephenson, Chem. Commun., 2013, 49, 4352 RSC;
(g) C. Battilocchio, J. M. Hawkins and S. V. Ley, Org. Lett., 2013, 15, 2278 CrossRef CAS PubMed;
(h) A. S. Kleinke and T. F. Jamison, Org. Lett., 2013, 15, 710 CrossRef CAS PubMed;
(i) K. Asano, Y. Uesugi and J. Yoshida, Org. Lett., 2013, 15, 2398 CrossRef CAS PubMed;
(j) L. Guetzoyan, N. Nikbin, I. R. Baxendale and S. V. Ley, Chem. Sci., 2013, 4, 764 RSC;
(k) S. Fuse, Y. Mifune and T. Takahashi, Angew. Chem., Int. Ed., 2014, 53, 851 CrossRef CAS PubMed;
(l) Z. He and T. F. Jamison, Angew. Chem., Int. Ed., 2014, 53, 3353 CrossRef CAS PubMed;
(m) A. Nagaki, Y. Takahashi and J. Yoshida, Chem. – Eur. J., 2014, 20, 7931 CrossRef CAS PubMed.
-
(a) Y. Tomida, A. Nagaki and J. Yoshida, J. Am. Chem. Soc., 2011, 133, 3744 CrossRef CAS PubMed;
(b) A. Nagaki, C. Matsuo, S. Kim, K. Saito, A. Miyazaki and J. Yoshida, Angew. Chem., Int. Ed., 2012, 51, 3245 CrossRef CAS PubMed;
(c) A. Nagaki, D. Ichinari and J. Yoshida, J. Am. Chem. Soc., 2014, 136, 12245 CrossRef CAS PubMed;
(d) A. Nagaki, Y. Tsuchihashi, S. Haraki and J. Yoshida, Org. Biomol. Chem., 2015, 13, 7140 RSC;
(e) A. Nagaki, K. Imai, S. Ishiuchi and J. Yoshida, Angew. Chem., Int. Ed., 2015, 54, 1914 CrossRef CAS PubMed.
-
(a) S. Suga, D. Yamada and J. Yoshida, Chem. Lett., 2010, 39, 404 CrossRef CAS;
(b) A. Nagaki, A. Kenmoku, Y. Moriwaki, A. Hayashi and J. Yoshida, Angew. Chem., Int. Ed., 2010, 49, 7543 CrossRef CAS PubMed.
-
(a) A. Nagaki, H. Kim and J. Yoshida, Angew. Chem., Int. Ed., 2008, 47, 7833 CrossRef CAS PubMed;
(b) A. Nagaki, H. Kim and J. Yoshida, Angew. Chem., Int. Ed., 2009, 48, 8063 CrossRef CAS PubMed;
(c) A. Nagaki, H. Kim, Y. Moriwaki, C. Matsuo and J. Yoshida, Chem. – Eur. J., 2010, 16, 11167 CrossRef CAS PubMed;
(d) A. Nagaki, H. Kim, C. Matuo and J. Yoshida, Org. Biomol. Chem., 2010, 8, 1212 RSC;
(e) H. Kim, A. Nagaki and J. Yoshida, Nat. Commun., 2011, 2, 264 CrossRef PubMed.
-
(a) S. Yamaguchi, C. Xu and T. Okamoto, Pure Appl. Chem., 2006, 78, 721 CAS;
(b) S. Yamaguchi and K. Tamao, J. Organomet. Chem., 2002, 653, 223 CrossRef CAS;
(c) M. Hissler, P. W. Dyer and E. Reau, Coord. Chem. Rev., 2003, 244, 1 CrossRef CAS;
(d)
Silicon-Containing Polymers: The Science and Technology of their Synthesis and Applications, ed. R. G. Jones, W. Ando and J. Chojnowski, Kluwer Academic Publishers, Dordrecht, The Netherlands, 2000 Search PubMed.
-
(a) D. Seyferth, S. C. Vick, M. L. Shannon, T. F. O. Lim and D. P. Duncan, J. Organomet. Chem., 1977, 135, C37 CrossRef CAS;
(b) H. Sakurai, Y. Kamiyama and Y. Nakadaira, J. Am. Chem. Soc., 1977, 99, 3879 CrossRef CAS;
(c) D. Seyferth, D. P. Duncan and S. C. Vick, J. Organomet. Chem., 1977, 125, C5 CrossRef CAS;
(d) R.-J. Hwang and P. P. Gaspar, J. Am. Chem. Soc., 1978, 100, 6626 CrossRef CAS;
(e) T. J. Barton and G. T. Bums, J. Organomet. Chem., 1979, 179, C17 CrossRef CAS;
(f) T. J. Barton, W. D. Wulff, E. V. Arnold and J. Clardy, J. Am. Chem. Soc., 1979, 101, 2733 CrossRef CAS;
(g) E. E. Siefert, K. L. Lob, R. A. Ferrieri and Y.-N. Tang, J. Am. Chem. Soc., 1980, 102, 2285 CrossRef CAS;
(h) H. Sakurai, Y. Kamiyama, A. Mikoda, T. Kobayashi, K. Sasaki and Y. Nakadaira, J. Organomet. Chem., 1980, 201, C14 CrossRef CAS;
(i) P. Jutzi and A. Karl, J. Organomet. Chem., 1981, 214, 289 CrossRef CAS;
(j) M. Ishikawa, H. Sugisawa, O. Harata and M. Kumada, J. Organomet. Chem., 1981, 217, 43 CrossRef CAS;
(k) M. Ishikawa, K. Nishimura, H. Sugisawa and M. Kumada, J. Organomet. Chem., 1981, 218, C21 CrossRef CAS.
-
(a) A. Nagaki, N. Takabayashi, Y. Tomida and J. Yoshida, Org. Lett., 2008, 10, 3937 CrossRef CAS PubMed;
(b) A. Nagaki, N. Takabayashi, Y. Tomida and J. Yoshida, Beilstein J. Org. Chem., 2009, 5, 16 Search PubMed;
(c) Y. Ishigaki, T. Suzuki, J. Nishida, A. Nagaki, N. Takabayashi, H. Kawai, K. Fujiwara and J. Yoshida, Materials, 2011, 4, 1906 CrossRef CAS.
-
(a) A. Nagaki, H. Kim and J. Yoshida, Angew. Chem., Int. Ed., 2009, 48, 8063 CrossRef CAS PubMed;
(b) A. Asai, A. Takata, Y. Ushiogi, Y. Iinuma, A. Nagaki and J. Yoshida, Chem. Lett., 2011, 40, 39 CrossRef;
(c) A. Nagaki, Y. Takahashi, A. Henseler, C. Matsuo and J. Yoshida, Chem. Lett., 2015, 44, 214 CrossRef;
(d) H. Kim, K. I. Min, K. Inoue, D. J. Im, D. P. Kim and J. Yoshida, Science, 2016, 352, 691 CrossRef CAS PubMed.
Footnote |
† Electronic supplementary information (ESI) available. See DOI: 10.1039/c6qo00257a |
|
This journal is © the Partner Organisations 2016 |