DOI:
10.1039/C6QO00099A
(Research Article)
Org. Chem. Front., 2016,
3, 985-988
A general route to sulfones via insertion of sulfur dioxide promoted by cobalt oxide†
Received
12th March 2016
, Accepted 16th June 2016
First published on 20th June 2016
Abstract
A cobalt-promoted coupling reaction of triethoxysilanes, sulfur dioxide, and electrophiles is developed. Benzylic, allylic or alkyl bromides are all efficient as electrophiles in this transformation. Moreover, the reactions with other electrophilic partners including iodonium salts and electron-poor (hetero)aryl chlorides proceed smoothly as well under the standard conditions. The sulfinate intermediates are generated in situ from the reaction of triethoxysilanes and sulfur dioxide under cobalt-promoted conditions, which subsequently combine with various electrophiles to produce the corresponding sulfones in moderate to good yields.
Introduction
Utilization of sulfur dioxide as a reliable synthetic reagent in organic synthesis is promising and attractive, considering the enormous scale of annual production.1 In the past few years, remarkable efforts have been devoted to the insertion of sulfur dioxide into small molecules.2,3 The pioneering work was contributed by Willis and co-workers, which reported the generation of N-aminosulfonamides through a palladium-catalyzed three-component coupling of aryl iodides, DABCO·(SO2)2 and hydrazines. The utilization of the bench-stable solid DABCO·(SO2)2 as the source of sulfur dioxide extended the reaction practicability of sulfur dioxide, considering the handling problem of this toxic gaseous reagent. So far, the rapid development of the application of this sulfur dioxide surrogate has been witnessed, particularly in the area of transition-metal catalyzed transformations.
The sulfonyl-derived functional groups are widely present in pharmaceutical and agrochemical molecules.4,5 The compounds featuring the sulfone unit have also been used as essential intermediates in organic synthesis due to their versatile reactivities.6 However, traditional methods for the generation of sulfones, including alkylation of sulfonate salts and oxidation of the corresponding sulfides or sulfoxides,7 usually suffered from the scope limitation and required procedures with harsh reaction conditions associated with the utilization of sulfonyl chlorides as the starting materials.8 In the past few years, the sulfonylation process through the direct insertion of sulfur dioxide has been well developed. For example, the reaction of organometallic reagents (such as Grignard reagents, organolithium reagents, and organozinc reagents) with sulfur dioxide would produce metal sulfinates, which could combine with electrophiles to provide the corresponding sulfones.9 Transition-metal-catalyzed sulfination of widely available halides or boronic acids could also proceed well to generate the corresponding sulfones or sulfamides.10 So far, transition metals such as palladium, gold and copper have been successfully applied in the above process (Scheme 1). For instance, Toste and co-workers reported the synthesis of sulfones through a gold(I)-catalyzed sulfination of arylboronic acids via insertion of sulfur dioxide.10c A similar transformation using palladium catalysts was developed by Willis subsequently.10e Very recently, we developed a facile route to sulfones via a copper(I)-catalyzed coupling of triethoxysilanes, sulfur dioxide, and alkyl halides.10f However, the electrophiles were only limited to alkyl halides. The reaction failed to provide the corresponding products when other electrophiles were employed.
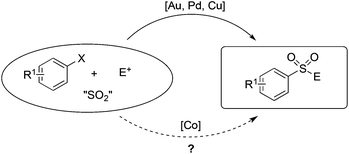 |
| Scheme 1 Transition-metal-catalyzed synthesis of sulfones via the insertion of sulfur dioxide. | |
Currently, development of catalysts based on earth-abundant first-row transition metals and catalysts has attracted much attention. Particularly, employing new non-precious-metal salts, e.g., those including Fe and Co, that can promote efficient insertion of sulfur dioxide is a challenging target. Among these earth-abundant first-row transition metals, cobalt salts have shown promising reactivities in various organic transformations.11 We envisioned that the utilization of cobalt salt in the fixation of sulfur dioxide would be attractive and highly desirable considering its low toxicity and low cost (Scheme 1). On the other hand, organosilanes have attracted continuous interest in cross-coupling reactions due to their advantages of low toxicity, high stability, ease of preparation and wide functional group tolerance.12 Herein, we would like to report a cobalt-promoted preparation of sulfinate derivatives from triethoxysilanes and DABCO·(SO2)2, and then combine with electrophilic partners to generate the corresponding sulfones.
Results and discussion
Our initial studies were performed for the coupling of triethoxyphenysilane 1a, DABCO·(SO2)2 and benzyl bromide 2a as a model for the optimization of the reaction conditions (Table 1). At the outset, the reaction was catalyzed with Co(OAc)2 in the presence of caesium fluoride in DMA under N2 at 100 °C (Table 1, entry 1). To our delight, the desired sulfonylation product 3a was produced in 29% yield. Further examination of solvents indicated that the reaction worked efficiently in DMF, affording the expected product 3a in 32% yield (Table 1, entries 2–5). No better results were obtained when other fluorine sources were employed instead of caesium fluoride (Table 1, entries 6 and 7). Other cobalt salts were next investigated and the yield was increased to 36% when CoO was utilized (Table 1, entries 8–12). Gratifyingly, the yield was improved to 48% while the amount of CoO was increased to 0.5 equivalents (Table 1, entry 13). Further exploration suggested that the reaction worked efficiently in the presence of 1.0 equivalent of CoO, leading to the corresponding sulfone 3a in 62% yield (Table 1, entry 14). This process took place smoothly at 80 °C as well, while a lower yield of 55% was obtained (Table 1, entry 15). Additionally, the reaction offered a much lower yield of 40% at 120 °C (Table 1, entry 16). Subsequently, water was added to this reaction as the co-solvent in view of the low solubility of the sulfinate salt in organic solvents. However, only 23% yield of product 3a was produced when 0.1 mL of H2O was added to the reaction mixture (Table 1, entry 17). Only a trace amount of product was detected when the reaction was carried out under air (Table 1, entry 18). The result was inferior when 1.0 equivalent of Co(OAc)2 was added to the reaction mixture, affording the corresponding product in 54% yield (Table 1, entry 19).
Table 1 Initial studies for the reaction of triethoxyphenylsilane 1a, DABCO·(SO2)2, and benzyl bromide 2aa

|
Entry |
Solvent |
“F” |
[Co] |
Yieldb (%) |
Reaction conditions: cobalt salt (0.03 mmol), triethoxyphenylsilane 1a (0.3 mmol), DABCO·(SO2)2 (0.3 mmol), benzyl bromide 2a (0.6 mmol), “F” source (0.6 mmol), solvent (2.0 mL), 100 °C, 12 h.
Isolated yield based on triethoxyphenylsilane 1a.
CoO (0.5 equivalents).
CoO (1.0 equivalent).
The reaction was performed at 80 °C.
The reaction occurred at 120 °C.
H2O (0.1 mL), DMF (2.0 mL).
Under air.
In the presence of Co(OAc)2 (1.0 equivalent).
|
1 |
DMA |
CsF |
Co(OAc)2 |
29 |
2 |
1,4-Dioxane |
CsF |
Co(OAc)2 |
25 |
3 |
CH3CN |
CsF |
Co(OAc)2 |
23 |
4 |
NMP |
CsF |
Co(OAc)2 |
30 |
5 |
DMF |
CsF |
Co(OAc)2 |
32 |
6 |
DMF |
NaF |
Co(OAc)2 |
Trace |
7 |
DMF |
TBAF |
Co(OAc)2 |
18 |
8 |
DMF |
CsF |
CoO |
36 |
9 |
DMF |
CsF |
CoBr2 |
29 |
10 |
DMF |
CsF |
CoCl2 |
30 |
11 |
DMF |
CsF |
Co(acac)2 |
27 |
12 |
DMF |
CsF |
CoCl(PPh3)3 |
33 |
13c |
DMF |
CsF |
CoO |
48 |
14d |
DMF |
CsF |
CoO |
62 |
15d,e |
DMF |
CsF |
CoO |
55 |
16d,f |
DMF |
CsF |
CoO |
40 |
17d,g |
H2O/DMF |
CsF |
CoO |
23 |
18d,h |
DMF |
CsF |
CoO |
Trace |
19i |
DMF |
CsF |
Co(OAc)2 |
54 |
The scope of the sulfonylation reaction of triethoxysilanes 1, DABCO·(SO2)2 and benzyl halides 2 was then investigated under the above optimized reaction conditions (Table 2). A range of triethoxysilanes 1 were combined with DABCO·(SO2)2 and benzyl halides 2 smoothly, leading to the corresponding products 3 in moderate to good yields. Triethoxyarylsilanes bearing electron-donating or electron-withdrawing groups on the aromatic ring were all tolerated well under the standard conditions, and the desired products 3a–3f were generated as expected. The reaction of triethoxyarylsilane bearing an ortho-substitutent worked efficiently as well to afford the corresponding product 3f in 57% yield. Heteroaromatic triethoxysilanes such as triethoxy(thiophen-2-yl)silane and 3-(triethoxysilyl)pyridine were next applied to this transformation, leading to the desired products 3g and 3h in moderate yields. Significantly, this process tolerated a broad range of triethoxyalkylsilanes including triethoxyallylsilane and triethoxybenzylsilane. For example, triethoxy(octyl)silane could be employed in this process, delivering the corresponding product 3l in 63% yield. Further investigation demonstrated that benzyl bromides with electron-withdrawing or electron-donating groups on the aromatic ring were both compatible in this transformation, affording the corresponding sulfones 3o–3v in moderate yields. Furthermore, the substrates featuring a variety of functional groups including ether, ester and halo were coupled smoothly under the standard conditions.
Table 2 Scope investigation of the sulfonylation reaction of triethoxysilane 1, DABCO·(SO2)2, and benzyl halide 2a,b
Reaction conditions: CoO (0.3 mmol), triethoxysilane 1 (0.3 mmol), DABCO·(SO2)2 (0.3 mmol), benzyl bromide 2 (0.6 mmol), CsF (0.6 mmol), DMF (2.0 mL), 100 °C, 12 h.
Isolated yield based on triethoxysilane 1.
|
|
We next expanded the scope of the electrophilic component in this cobalt-promoted sulfonylation reaction (Table 3). Other alkyl halides were examined initially. The allyl bromide (E)-(3-bromoprop-1-enyl)benzene was proven to be suitable for this transformation, providing the desired product 3w in 53% yield. However, the coupling with tert-butyl 2-bromoacetate afforded the corresponding product 3x in 26% yield. Reactions of other unactivated alkyl halides were carried out in the meantime, providing the desired sulfones (3y and 3z) in moderate yields.
Table 3 Sulfonylation reaction of triethoxysilane 1, DABCO·(SO2)2, and alkyl bromidesa
Isolated yield based on triethoxysilane 1.
|
|
Additionally, we found that diaryl sulfones could be generated smoothly while iodonium salts and electron-poor (hetero)aryl chlorides were applied as the electrophilic partners in the reaction of triethoxysilane 1 with the insertion of sulfur dioxide (Scheme 2). For example, sulfone 3aa was obtained in 60% yield under the standard conditions. When 2-chlorobenzo[d]thiazole or 2-chloro-4-nitropyridine was employed as the electrophile in the cobalt-promoted reaction of triethoxysilanes 1 with sulfur dioxide, the corresponding products were generated as expected. Thus, this approach provides an efficient pathway for the assembly of sulfones with heterocyclic units.
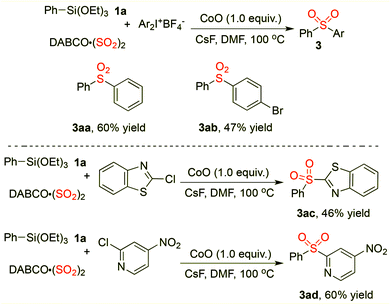 |
| Scheme 2 Sulfonylation reaction of triethoxysilane 1, DABCO·(SO2)2, and iodonium salts or electron-poor aryl chlorides. | |
Conclusions
In conclusion, we have described a facile route to sulfones via a cobalt-promoted three-component reaction of triethoxysilanes, DABCO·(SO2)2, and various electrophiles. The utilization of the less toxic and inexpensive cobalt catalyst is attractive and practical since palladium or gold catalysts were usually required in the previous reports. Moreover, this process takes place under mild conditions and shows broad functional group tolerance. The electrophilic partners including alkyl halides, iodonium salts, and electron-poor (hetero)aryl chlorides are involved in this reaction under standard conditions. We believe that the application of non-precious-metal salts in the fixation of sulfur dioxide would open a new avenue in this field.
Acknowledgements
Financial support from the National Natural Science Foundation of China (No. 21372046, 21532001, 81571401) and the New Hundred Talents Program of Shanghai Municipal Commission of Health and Family Planning (13B122) is gratefully acknowledged.
Notes and references
-
(a)
S. D. Burke, in Encyclopedia of Reagents for Organic Synthesis, ed. L. A. Paquette, Wiley, Chichester, 1995, vol. 7, p. 4688 Search PubMed;
(b) Z. Florjańczyk and D. Raducha, Pol. J. Chem., 1995, 69, 481 Search PubMed;
(c) P. Vogel, M. Turks, L. Bouchez, D. Markovic, A. Varela-Álvarez and J. A. Sordo, Acc. Chem. Res., 2007, 40, 931 CrossRef CAS PubMed;
(d)
Ullmann's Encyclopedia of Industrial Chemistry, ed. B. Elvers, S. Hawkins and W. Russey, VCH, Weinheim, Germany, 5th edn, 1994, vol. A25 Search PubMed.
- For reviews:
(a) G. Liu, C. Fan and J. Wu, Org. Biomol. Chem., 2015, 13, 1592 RSC;
(b) P. Bisseret and N. Blanchard, Org. Biomol. Chem., 2013, 11, 5393 RSC;
(c) A. S. Deeming, E. J. Emmett, C. S. Richards-Taylor and M. C. Willis, Synthesis, 2014, 2701 Search PubMed.
-
(a) B. Nguyen, E. J. Emmet and M. C. Willis, J. Am. Chem. Soc., 2010, 132, 16372 CrossRef CAS PubMed;
(b) E. J. Emmet, C. S. Garcia-Rubia, B. Nguyen, A. B. Garcia-Rubia, R. Hayter and M. C. Willis, Org. Biomol. Chem., 2012, 10, 4007 RSC;
(c) L. Martial, Synlett, 2013, 24, 1595 CrossRef;
(d) W. Li, H. Li, P. Langer, M. Beller and X.-F. Wu, Eur. J. Org. Chem., 2014, 3101 CrossRef;
(e) W. Li, M. Beller and X.-F. Wu, Chem. Commun., 2014, 50, 9513 RSC;
(f) X. Wang, L. Xue and Z. Wang, Org. Lett., 2014, 16, 4056 CrossRef CAS PubMed;
(g) A. S. Deeming, C. J. Russell and M. C. Willis, Angew. Chem., Int. Ed., 2015, 54, 1168 CrossRef CAS PubMed;
(h) S. Ye and J. Wu, Chem. Commun., 2012, 48, 7753 RSC;
(i) S. Ye and J. Wu, Chem. Commun., 2012, 48, 10037 RSC;
(j) D. Zheng, Y. An, Z. Li and J. Wu, Angew. Chem., Int. Ed., 2014, 53, 2451 CrossRef CAS PubMed;
(k) S. Ye, H. Wang, Q. Xiao, Q. Ding and J. Wu, Adv. Synth. Catal., 2014, 356, 3225 CrossRef CAS;
(l) Y. An, D. Zheng and J. Wu, Chem. Commun., 2014, 50, 11746 RSC;
(m) Y. Luo, X. Pan, C. Chen, L. Yao and J. Wu, Chem. Commun., 2015, 51, 180 RSC;
(n) D. Zheng, Y. Li, Y. An and J. Wu, Chem. Commun., 2014, 50, 8886 RSC;
(o) A. S. Tsai, J. M. Curto, B. N. Rocke, A. R. Dechert-Schmitt, G. K. Ingle and V. Mascitti, Org. Lett., 2016, 18, 508 CrossRef CAS PubMed.
-
(a) Y. Harrak, G. Casula, J. Basset, G. Rosell, S. Plescia, D. Raffa, M. G. Cusimano, R. Pouplana and M. D. Pujol, J. Med. Chem., 2010, 53, 6560 CrossRef CAS PubMed;
(b) D. A. Smith and R. M. Jones, Curr. Opin. Drug Discovery Dev., 2008, 11, 72 CAS;
(c) Y. Noutoshi, M. Ikeda, T. Saito, H. Osada and K. Shirasu, Front. Plant Sci., 2012, 3, 245 CrossRef PubMed.
-
(a)
M. Bartholow, Top 200 Drugs of 2011, Pharmacy Times. http://www.pharmacytimes.com/publications/issue/2012/July2012/Top-200-Drugs-of-2011, accessed on Jan 9, 2013 Search PubMed;
(b) For a list of top drugs by year, see: http://cbc.arizona.edu/njardarson/group/top-pharmaceuticals-poster, accessed on Jan 9, 2013;
(c) J. Drews, Science, 2000, 287, 1960 CrossRef CAS PubMed.
-
(a) P. J. Crowley, J. Fawcett, B. M. Kariuki, A. C. Moralee, J. M. Percy and V. Salafia, Org. Lett., 2002, 4, 4125 CrossRef CAS PubMed;
(b)
N. S. Simpkins, Sulfones in Organic Synthesis, Pergamon Press, Oxford, 1993 Search PubMed.
-
(a) K. Sato, M. Hyodo, M. Aoki, X.-Q. Zheng and R. Noyori, Tetrahedron, 2001, 57, 2469 CrossRef CAS;
(b) B. M. Trost and R. Baslau, J. Org. Chem., 1968, 53, 532 CrossRef;
(c) T. Durst, Compr. Org. Chem., 1979, 3, 171 CAS;
(d) G. E. Vennstra and B. Zwanenburg, Synthesis, 1975, 519 CrossRef CAS;
(e) Y. Ju, D. Kumar and R. S. Varma, J. Org. Chem., 2006, 71, 6697 CrossRef CAS PubMed.
-
(a) K. Baharami, M. M. Khodaei and D. Khaledian, Tetrahedron Lett., 2012, 53, 354 CrossRef;
(b) G. K. S. Prakash, T. Mathew and G. A. Olah, J. Org. Chem., 2007, 72, 5847 CrossRef CAS PubMed;
(c) S. W. Wright and K. N. Hallstrom, J. Org. Chem., 2006, 71, 1080 CrossRef CAS PubMed;
(d) V. Percec, T. K. Bera, B. B. De, Y. Sanai, J. Smith, M. N. Holerca, B. Barboiu, B. B. B. Grubbs and J. M. J. Fréchet, J. Org. Chem., 2001, 66, 2104 CrossRef CAS PubMed;
(e) R. J. Watson, D. Batty, A. D. Baxter, D. R. Hannah, D. A. Owen and J. G. Montana, Tetrahedron Lett., 2002, 43, 683 CrossRef CAS.
-
(a) C. C. Chen and J. Waser, Org. Lett., 2015, 17, 736 CrossRef CAS PubMed;
(b) A. S. Deeming, C. J. Rusell, A. J. Hennessy and M. C. Willis, Org. Lett., 2014, 16, 150 CrossRef CAS PubMed;
(c) B. N. Rocke, K. B. Bahnck, M. Herr, S. Lavergne, V. Mascitti, C. Perreault, J. Polivkova and A. Shavnya, Org. Lett., 2014, 16, 154 CrossRef CAS PubMed;
(d) E. J. Emmett, B. R. Hayter and M. C. Willis, Angew. Chem., Int. Ed., 2013, 52, 12679 CrossRef CAS PubMed.
-
(a) E. J. Emmett, B. R. Hayter and M. C. Willis, Angew. Chem., Int. Ed., 2014, 38, 10204 CrossRef PubMed;
(b) A. Shavnya, S. B. Coffey, A. C. Smith and V. Mascitti, Org. Lett., 2013, 15, 6226 CrossRef CAS PubMed;
(c) M. W. Johnson, S. W. Bagley, N. P. Mankad, R. G. Bergman, V. Mascitti and F. D. Toste, Angew. Chem., Int. Ed., 2014, 53, 4404 CrossRef CAS PubMed;
(d) A. Shavnya, K. D. Hesp, V. Mascitti and A. C. Smith, Angew. Chem., Int. Ed., 2015, 54, 13571 CrossRef CAS PubMed;
(e) A. S. Deeming, C. J. Russell and M. C. Willis, Angew. Chem., Int. Ed., 2016, 55, 747 CrossRef CAS PubMed;
(f) D. Zheng, R. Mao, Z. Li and J. Wu, Org. Chem. Front., 2016, 3, 359 RSC;
(g) W. Zhang and M. Luo, Chem. Commun., 2016, 52, 2980 RSC.
- For selected examples, see:
(a) K. Gao and N. Yoshikai, Acc. Chem. Res., 2014, 47, 1208 CrossRef CAS PubMed;
(b) W. Liu, H. Cao, J. Xin, L. Jin and A. Lei, Chem. – Eur. J., 2011, 17, 3588 CrossRef CAS PubMed;
(c) Q. Chen, L. Ilies, N. Yoshikai and E. Nakamura, Org. Lett., 2011, 13, 3232 CrossRef CAS PubMed;
(d) G. Cahiez and A. Moyeux, Chem. Rev., 2010, 110, 1435 CrossRef CAS PubMed.
- For selected examples, see:
(a) J. Yu, J. Liu, G. Shi, C. Shao and Y. Zhang, Angew. Chem., Int. Ed., 2015, 54, 4079 CrossRef CAS PubMed;
(b) J. He, R. Takise, H. Fu and J. Yu, J. Am. Chem. Soc., 2015, 137, 4618 CrossRef CAS PubMed;
(c) S. Shi and Y. Zhang, J. Org. Chem., 2007, 72, 5927 CrossRef CAS PubMed;
(d) S. K. Gurung, S. Thapa, A. S. Vangala and R. Giri, Org. Lett., 2013, 15, 5378 CrossRef CAS PubMed;
(e) Z. Wang and S. Chang, Org. Lett., 2013, 15, 1990 CrossRef CAS PubMed.
Footnote |
† Electronic supplementary information (ESI) available. See DOI: 10.1039/c6qo00099a |
|
This journal is © the Partner Organisations 2016 |