DOI:
10.1039/C6QO00020G
(Research Article)
Org. Chem. Front., 2016,
3, 630-634
Ascorbic acid promoted [4 + 2] benzannulation: a mild, operationally simple approach to the synthesis of phenanthrenes†
Received
15th January 2016
, Accepted 19th March 2016
First published on 21st March 2016
Abstract
A mild, simple and metal-free radical [4 + 2] benzannulation for the generation of phenanthrenes has been developed. A substoichiometric reductant, ascorbic acid (vitamin C), is harnessed as the radical initiator. This method has a broad substrate scope, and various substituted phenanthrenes were obtained in moderate to good yields at room temperature without irradiation.
Introduction
Polycyclic aromatic hydrocarbons, especially phenanthrenes, are a prominent class of compounds in organic chemistry. The structure of phenanthrene could be found in diverse natural products,1 pharmaceuticals,2 and functional materials.3 Thus, many kinds of methods have been reported for the synthesis of phenanthrenes over the past few decades.4 One of the methods for their preparation is transition metal-catalyzed [4 + 2] benzannulation of biaryl compounds with alkynes, and it has drawn much attention.5 Other approaches, including intramolecular aryl–aryl bond formation from stilbene derivatives,6 ring-closing olefin metathesis,7 and cycloisomerization,8 contribute as alternatives to provide phenanthrenes.
Arenediazonium salts can serve as precursors of highly reactive aryl radicals in C–C bond forming reactions,9 and the [4 + 2] benzannulation of biaryl diazonium salts with alkynes could afford phenanthrenes efficiently from readily-available starting materials.10 In 2012, Zhou's report demonstrated an eosin Y-catalyzed, visible light-induced synthesis of phenanthrenes.11 In this chemistry, oxidative quenching of the photo-excited photocatalyst with biaryl diazonium salts gives biaryl radicals. After that, Studer and co-workers employed isoamyl nitrite for in situ diazonium salt generation, Bu4NI as a radical initiator for the formation of an aryl radicals, which undergo addition to alkynes and subsequent base-promoted homolytic aromatic substitution (BHAS) to provide phenanthrenes.12 However, in these two protocols, either heating or irradiation is required for the transformation.
In fact, arenediazonium ions generated in situ could be reduced to aryl radicals by a “green” reducing reagent, L-threo-ascorbic acid, through an inner-sphere mechanism under very mild conditions. In this case, ascorbic acid indeed acts as a reductant instead of a Lewis acid, for which solid evidence has been reported by Bravo-Díaz and co-workers.13 This elegant method has recently received much attention, and it was successfully utilized by Martin and Carrillo for the metal-free C–H arylation of (hetero)arenes.14 Later, our group reported an ascorbic acid promoted synthesis of aryl sulfides,15 involving the reaction of aryl radicals and disulfides. The strategy was also applied by Ranu's group for the oxidative arylation of vinylarenes,16 which allows the assembly of 1,2-diarylated ethanones. We speculate that biaryl radicals generated by this manner may react with alkynes to produce substituted phenanthrenes via radical cascade processes. Herein, we wish to report an ascorbic acid promoted [4 + 2] benzannulation with ortho-amino-biaryls nitrosated in situ under mild conditions without irradiation (Scheme 1).
 |
| Scheme 1 Ascorbic acid promoted [4 + 2] benzannulation with ortho-amino-biaryls nitrosated in situ. | |
Results and discussion
To test our hypothesis, biphenyl-2-amine (1a) was treated with methyl propiolate (2a), ascorbic acid, and tert-butyl nitrite in acetonitrile at room temperature for 12 hours under nitrogen (Table 1, entry 1). To our delight, the substituted phenanthrene was obtained in 58% yield along with biphenyl as the main by-product,17 and the hydroamination product could not be detected. The reaction time, amount of 2a and different solvents were then screened to optimize the reaction conditions, and the results are shown in Table 1. A reaction time of 12 hours, five equivalents of 2a and DMSO as the solvent provided the highest yield (Table 1, entry 6). Considering that the innate chain might be a BHAS process,12 a base was tested as an additive. However, the addition of a base resulted in an obvious decrease of the yield (Table 1, entries 12 and 13). This could be because diazonium salts are labile towards bases. When the experiment was conducted in the absence of ascorbic acid, the reaction became sluggish and only 18% yield was obtained after a reaction time of 12 hours (Table 1, entry 14). Although the amount of alkyne has been reduced compared to Studer's protocol,12 five equivalents of alkyne is still a large excess and this is a big obstacle for its practical application. We tried to reduce the amount in some ways,18 and succeeded to obtain 73% yield with three equivalents of 2a by using 0.5 mL DMSO as the solvent.
Table 1 Reaction optimizationa

|
Entry |
Solvent |
2a (equiv.) |
Additive |
Yield (%)b |
Reaction conditions: 1a (0.2 mmol), 2a (excess), ascorbic acid (10 mol%), t-BuONO (1.5 equiv., 0.3 mmol), solvent (1 mL), additive (1 equiv.), under N2, rt.
Isolated yields.
8 h.
24 h.
Without ascorbic acid.
DMSO (0.5 mL).
|
1 |
CH3CN |
3 |
— |
58, 51,c 55d |
2 |
CH3CN |
5 |
— |
63 |
3 |
CH3CN |
10 |
— |
60 |
4 |
PhCF3 |
5 |
— |
56 |
5 |
CH3OH |
5 |
— |
35 |
6
|
DMSO
|
5
|
—
|
71
|
7 |
DMF |
5 |
— |
21 |
8 |
NMP |
5 |
— |
10 |
9 |
1,4-Dioxane |
5 |
— |
24 |
10 |
THF |
5 |
— |
5 |
11 |
H2O |
5 |
— |
15 |
12 |
DMSO |
5 |
NaOAc |
29 |
13 |
DMSO |
5 |
CsOAc |
3 |
14e |
DMSO |
5 |
— |
18 |
15
|
DMSO
|
3
|
—
|
73
|
With the optimal reaction parameters identified, various alkynes were investigated to explore the scope of the [4 + 2] benzannulation. As shown in Table 2, the reaction tolerated a series of aryl alkynes besides methyl and ethyl propiolates. A variety of aryl alkynes carrying electron-donating and -withdrawing substituents at the para position could successfully react with 1a to afford products in good yields. Alkyloxy, acetyl, trifluoromethyl, and carbon–halogen bonds remained intact during the ascorbic acid promoted benzannulation. The yields were almost the same obtained by the reactions of 1a with arylacetylenes 2d, 2l and 2m respectively, indicating that the steric effect is not significant. It is important to note that heteroaryl alkynes 2o and 2p could provide the corresponding products with good yields (3ao and 3ap). Cyclopropyl acetylene (2q) and trimethylsilylacetylene (2r) could also serve as substrates for this transformation, and moderate yields were obtained by both of the reactions. This radical [4 + 2] benzannulation was also tested on two internal alkynes (2s, 2t), however, low yields were obtained by both of the reactions.
Table 2 Ascorbic acid promoted [4 + 2] benzannulation: alkyne scopea
Reaction conditions: 1a (0.2 mmol), 2 (1 mmol), ascorbic acid (10 mol%), t-BuONO (0.3 mmol), DMSO (1 mL), under N2, rt, isolated yield.
Reaction conditions: 1a (0.2 mmol), 2 (0.6 mmol), ascorbic acid (10 mol%), t-BuONO (0.3 mmol), DMSO (0.5 mL), under N2, rt, isolated yield.
|
|
Having established the scope of the broad scope of alkynes, we next focused on varying the substituents on the aminobiphenyl component (Table 3). The substituents, including alkyl, alkyloxy, halo, cyano, trifluoromethyl and nitro, on both aromatic rings were all tolerated in the benzannulation. The reaction of phenylacetylene with substrate 1e gave a relatively low yield, because of the low solubility of 1e in DMSO. meta-Difluoro-biaryl amine (1h) worked well for the reaction. However, when only one ortho hydrogen atom was available, the yield turned out to be lower (3ic).
Table 3 Ascorbic acid promoted [4 + 2] benzannulation: aminodiphenyl scopea
Reaction conditions: 1 (0.2 mmol), 2c (1 mmol), ascorbic acid (10 mol%), t-BuONO (0.3 mmol), DMSO (1 mL), under N2, rt, isolated yield.
|
|
In order to further demonstrate the practicality and generality of the ascorbic acid promoted [4 + 2] benzannulation, biphenyl-2-amine (1a) and phenylacetylene (2c) were employed in a gram-scale reaction, which could be effectively scaled up with slightly lower efficiency, affording 3ac in 78% yield (Scheme 2).
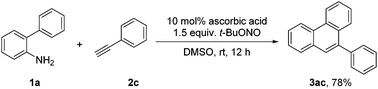 |
| Scheme 2 Scale-up experiment. Reaction conditions: 1a (4 mmol), 2c (12 mmol), ascorbic acid (10 mol%), t-BuONO (6 mmol), DMSO (10 mL), under N2, rt. Isolated yield. | |
According to previous reviews by Studer and Curran,19 diverse radical cascade reactions, including BHAS, are grouped under electron catalysis. So a possible mechanism of this transformation with an electron-catalyzed innate cycle is depicted in Scheme 3. In the first step, biaryl amine 1a is nitrosated by tert-butyl nitrite to produce diazonium salt A. The reaction was initiated upon the protonation of A by ascorbic acid, resulting in the formation of diazonium salt Bvia the change of the anion to ascorbate. The diazonium ion is reduced by ascorbate through a single-electron transfer to generate a biaryl radical C, an ascorbyl radical and nitrogen. The ascorbyl radical could dismutate into dehydroascorbic acid and ascorbic acid, which would react with another dizonium salt A. Meanwhile, vinyl radical D is afforded by the addition of an aryl radical C to alkyne, followed by an intramolecular radical cyclization to give cyclized radical intermediate E. Then, deprotonated by tert-butanolate, the conjugated radical anion F is formed, and t-BuOH generated could accelerate the dismutation of the ascorbyl radical.20 Finally, product 3 was obtained after the formal liberation of an electron from F. In this step, diazonium salt A is easily reduced by this electron to give tert-butanolate and another radical C, which completes the innate chain cycle. The possibility of propagation by electron transfer from cyclohexadienyl radical E to the diazonium salt A is small, based on the report by Majek and Wangelin.21
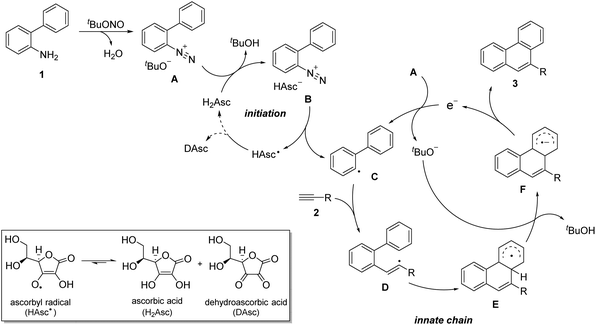 |
| Scheme 3 Proposed mechanism. | |
Conclusions
In summary, we have described a mild, operationally simple, and metal-free approach to generate phenanthrenes. In this chemistry, various substituted phenanthrenes were obtained from readily-prepared starting materials with a substoichiometric amount of ascorbic acid at room temperature. The radical initiator ascorbic acid and its decomposition product dehydroascorbic acid are both edible. The mildness of this protocol makes it appealing for a variety of applications.
Experimental section
General procedure for the ascorbic acid promoted synthesis of phenanthrenes
A 10 mL Schlenk tube with a magnetic stirring bar was charged with L-ascorbic acid (0.1 equiv., 4 mg), ortho-amino-biaryl (0.2 mmol) and alkyne (1.0 mmol, 5 equiv. or 0.6 mmol, 3 equiv.). The tube was evacuated and backfilled with dry nitrogen (this operation was repeated three times). DMSO (1 mL or 0.5 mL) was then added by a syringe. The mixture was stirred vigorously for 1 minute before t-BuONO (0.3 mmol, 1.5 equiv., 36 μL) was added via a syringe. After the resulting mixture was stirred at r.t. for 12 hours, the reaction mixture was diluted with water (10 mL) and extracted with diethyl ether (3 × 10 mL). The combined organic layers were washed with brine, dried over K2SO4, and concentrated in vacuo. Purification of the crude product was achieved by column chromatography.
Acknowledgements
We gratefully acknowledge Natural Science Foundation of Jiangsu Province (BK 20131346, BK 20140776). This work was also supported by National Natural Science Foundation of China (21476116, 21402093) and Chinese Postdoctoral Science Foundation (2015M571761).
Notes and references
-
(a) Z. Wang, P. Wei, X. Xizhi, Y. Liu, L. Wang and Q. Wang, J. Agric. Food Chem., 2012, 60, 8544 CrossRef CAS PubMed;
(b) G. M. Cragg and D. J. Newman, J. Nat. Prod., 2004, 67, 232 CrossRef CAS PubMed;
(c) Z. Li, Z. Jin and R. Huang, Synthesis, 2001, 2365 CAS.
-
(a) J. G. Cannon, P. R. Khonje and J. P. Long, J. Med. Chem., 1975, 18, 110 CrossRef CAS PubMed;
(b) L. Wei, A. Brossi, R. Kendall, K. F. Bastow, S. L. Morris-Natschke, Q. Shi and K.-H. Lee, Bioorg. Med. Chem., 2006, 14, 6560 CrossRef CAS PubMed;
(c) P. Datla, M. D. Kalluri, K. Basha, A. Bellary, R. Kshirsagar, Y. Kanekar, S. Upadhyay, S. Singh and V. Rajagopal, Br. J. Pharmacol., 2010, 160, 1158 CrossRef CAS PubMed.
-
(a) R. Mitsuhashi, Y. Suzuki, Y. Yamanari, H. Mitamura, T. Kambe, N. Ikeda, H. Okamoto, A. Fujiwara, M. Yamaji, N. Kawasaki, Y. Maniwa and Y. Kubozono, Nature, 2010, 464, 76 CrossRef CAS PubMed;
(b) J. Li, G. Hu, N. Wang, T. Hu, Q. Wen, P. Lu and Y. Wang, J. Org. Chem., 2013, 78, 3001 CrossRef CAS PubMed;
(c) Y. Matsuo, Y. Sato, M. Hashiguchi, K. Matsuo and E. Nakamura, Adv. Funct. Mater., 2009, 19, 2224 CrossRef CAS;
(d) K. Pelzer, L. Greenman, G. Gidofalvi and D. A. Mazziotti, J. Phys. Chem. A, 2011, 115, 5632 CrossRef CAS PubMed.
- For reviews, see:
(a) R. G. Harvey, Curr. Org. Chem., 2004, 8, 303 CrossRef CAS;
(b) S. Kotha, S. Misra and S. Halder, Tetrahedron, 2008, 64, 10775 CrossRef CAS;
(c) A. J. Floyd, S. F. Dyke and S. E. Ward, Chem. Rev., 1976, 76, 509 CrossRef CAS;
(d) K. H. Dötz, Angew. Chem., Int. Ed. Engl., 1984, 23, 587 CrossRef;
(e) P. de Mendoza and A. M. Echavarren, Pure Appl. Chem., 2010, 82, 801 CrossRef CAS.
-
(a) Z. Shi, S. Ding, Y. Cui and N. Jiao, Angew. Chem., Int. Ed., 2009, 48, 7895 CrossRef CAS PubMed;
(b) C. Wang, S. Rakshit and F. Glorius, J. Am. Chem. Soc., 2010, 132, 14006 CrossRef CAS PubMed;
(c) A. Matsumoto, L. Ilies and E. Nakamura, J. Am. Chem. Soc., 2011, 133, 6557 CrossRef CAS PubMed;
(d) H. Yoshida, S. Kawashima, Y. Takemoto, K. Okada, J. Ohshita and K. Takaki, Angew. Chem., Int. Ed., 2012, 51, 235 CrossRef CAS PubMed.
-
(a) J. F. Almeida, L. Castedo, D. Fernández, A. G. Neo, V. Romero and G. Tojo, Org. Lett., 2003, 5, 4939 CrossRef CAS PubMed;
(b) K. Wang, M. Lü, A. Yu, X. Zhu and Q. Wang, J. Org. Chem., 2009, 74, 935 CrossRef CAS PubMed;
(c) E. C. Taylor, J. G. Andrade, G. J. H. Rall and A. McKillop, J. Am. Chem. Soc., 1980, 102, 6513 CrossRef CAS.
-
(a) A. Iuliano, P. Piccioli and D. Fabbri, Org. Lett., 2004, 6, 3711 CrossRef CAS PubMed;
(b) E. R. Walker, S. Y. Leung and A. G. M. Barrett, Tetrahedron Lett., 2005, 46, 6537 CrossRef CAS.
-
(a) Y.-L. Liu, Y. Liang, S.-F. Pi, X.-C. Huang and J.-H. Li, J. Org. Chem., 2009, 74, 3199 CrossRef CAS PubMed;
(b) Y. Kwon, H. Cho and S. Kim, Org. Lett., 2013, 15, 920 CrossRef CAS PubMed.
- For reviews, see:
(a) C. Galli, Chem. Rev., 1988, 88, 765 CrossRef CAS;
(b) F. Mo, G. Dong, Y. Zhang and J. Wang, Org. Biomol. Chem., 2013, 11, 1582 RSC;
(c) D. P. Hari and B. König, Angew. Chem., Int. Ed., 2013, 52, 4734 CrossRef CAS PubMed.
- R. Leardini, D. Nanni, A. Tundo and G. Zanardi, Synthesis, 1988, 333 CrossRef CAS.
- T. Xiao, X. Dong, Y. Tang and L. Zhou, Adv. Synth. Catal., 2012, 354, 3195 CrossRef CAS.
- M. Hartmann, C. G. Daniliuc and A. Studer, Chem. Commun., 2015, 51, 3121 RSC.
- U. Costas-Costas, E. González-Romero and C. Bravo-Díaz, Helv. Chim. Acta, 2001, 84, 632 CrossRef CAS.
- F. P. Crisostomo, T. Martin and R. Carrillo, Angew. Chem., Int. Ed., 2014, 53, 2181 CrossRef CAS PubMed.
- M. J. Bu, G. P. Lu and C. Cai, Synlett, 2015, 1841 CAS.
- B. Majhi, D. Kundu and B. C. Ranu, J. Org. Chem., 2015, 80, 7739 CrossRef CAS PubMed.
- The quenching of the biphenyl radical by the solvent and trace oxygen generates biphenyl via hydrogen abstraction. See:
(a) M. Majek, F. Filace and A. Jacobi von Wangelin, Chem. – Eur. J., 2015, 21, 4518 CrossRef CAS PubMed;
(b) J. Hofmann, H. Jasch and M. R. Heinrich, J. Org. Chem., 2014, 79, 2314 CrossRef CAS PubMed.
- For the experiments in an attempt to reduce the amount of alkyne see the ESI.†.
-
(a) A. Studer and D. P. Curran, Nat. Chem., 2014, 6, 765 CrossRef CAS PubMed;
(b) A. Studer and D. P. Curran, Angew. Chem., Int. Ed., 2016, 55, 58 CrossRef CAS PubMed.
- J. J. Warren and J. M. Mayer, J. Am. Chem. Soc., 2008, 130, 7546 CrossRef CAS PubMed.
- M. Majek, F. Filace and A. J. V. Wangelin, Beilstein J. Org. Chem., 2014, 10, 981 CrossRef CAS PubMed.
Footnote |
† Electronic supplementary information (ESI) available: Experimental procedures for the synthesis, spectral data and NMR spectra. See DOI: 10.1039/c6qo00020g |
|
This journal is © the Partner Organisations 2016 |
Click here to see how this site uses Cookies. View our privacy policy here.