DOI:
10.1039/C5QO00432B
(Research Article)
Org. Chem. Front., 2016,
3, 447-452
Visible-light promoted intermolecular halofunctionalization of alkenes with N-halogen saccharins†
Received
18th December 2015
, Accepted 22nd January 2016
First published on 26th January 2016
Abstract
We reported herein a simple and efficient visible-light promoted intermolecular haloamination and haloetherification of alkenes using N-halosaccharin as the halogen and nitrogen/oxygen source (halo = Br, Cl). The reaction can be applied to a very broad range of alkenes to generate haloamines and haloethers with high isolated yields. With DBU as the base, metal-free C–H amination of aryl olefins has also been achieved with good yields.
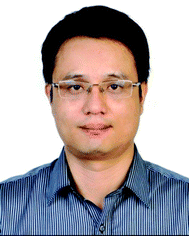 Sanzhong Luo | Sanzhong Luo was born in 1977 in Henan, China. He graduated from Zhengzhou University in 1999, and then spent his graduate studies successively in Nankai University, the Chinese Academy of Sciences (CAS), and the Ohio State University (USA), and received his PhD from CAS in 2005 under the supervision of Prof. Jin-Pei Cheng. He was a visiting scholar at Stanford University in 2009 (with B. M. Trost). He started his independent career in July 2005 in the Institute of Chemistry, Chinese Academy of Sciences (ICCAS) and became full professor there in 2011. His laboratory work focuses on asymmetric catalysis and synthesis, emphasizing the development of new catalysts and catalytic modes by drawing inspirations from nature. |
Introduction
Halofunctionalization of alkenes is a fundamental transformation to produce halogenated compounds as versatile synthons in organic synthesis.1 Typically, the reactions occur via halogenium ion or aziridinium ion intermediates (Scheme 1a and b).2,3 In catalytic halofunctionalization, both Lewis acids and Lewis bases have been reported to promote the reaction by facilitating halogenium/aziridinium ion formation (Scheme 1).4 Radical-based halofunctionalization was also known, in which photolysis or high temperatures are normally required to generate halogen radicals, and this type of radical process is of limited synthetic applicability due to the harsh reaction conditions and consequently less control over reactivity and selectivity.5 Previously, photolytic haloamidation with N-bromo imide has been reported by Skell (Scheme 1c).6 However, the process required UV-irradiation and the synthetic applicability remains largely underdeveloped. Herein, we have reported a distinctively simple and efficient haloamination and haloetherification of alkenes enabled by visible-light photolysis of N-bromosaccharin (Scheme 1).
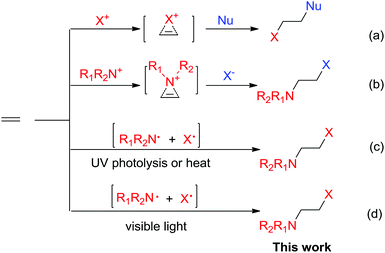 |
| Scheme 1 Strategies of halofunctionalization. | |
Results and discussion
Recently, we developed a visible-light promoted catalyst-free imidation of arenes and heteroarenes.7 In the reaction, N-bromosaccharin (NBSA) has been found to undergo visible light photolysis to generate imidyl and bromo radicals under rather mild conditions. In our continued efforts, we further examined the reaction of NBSA with simple alkenes under visible light conditions. When a solution of NBSA and styrene (1.1 equiv.) in CH2Cl2 was exposed to ambient light at ambient temperature, the reaction proceeded extremely fast to completion in 1 minute. Besides the expected bromoamidation adduct 3a (64% yield), we also isolated a bromoether adduct 4a in 31% yield (Fig. 1). No reaction was observed when the experiment was conducted in the dark (ESI, Scheme S1†). Unfortunately, further optimizations didn't lead to improvement in the chemoselectivity of the formation of bromoamidation and bromoetherification products. As these two adducts were easily separated, we went further to examine the applicability of this visible light photolytic reaction.
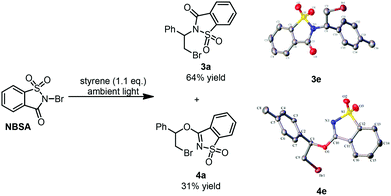 |
| Fig. 1 Reaction of NBSA and styrene. X-ray structures were determined for analogous 3e and 4e. | |
The scope of this mild halofunctionalization was next investigated (Scheme 2). Styrenes bearing either electron-withdrawing or electron-donating groups, were well-tolerated to give the bromoamidation and bromoetherification products in high isolated yields (3a–3f, 4a–4f). 2-Vinylnaphthalene was also feasible to generate the corresponding adducts (3h, 4h). Terminal substituted styrenes such as (E)- and (Z)-β-methylstyrene could also be applied in the reactions. In these cases, the (E)-isomer led to a diastereoselective anti-product in a >10
:
1 ratio for both amination and esterification adducts, while the (Z)-isomer showed rather poor anti/syn selectivity (1/2.6 and 1/1.8 for amination and esterification, respectively). These results stand in contrast to the high stereospecificity normally observed in a halogenium or aziridinium-type halofunctionalization process,8 and suggest a departure from the cationic mechanism.
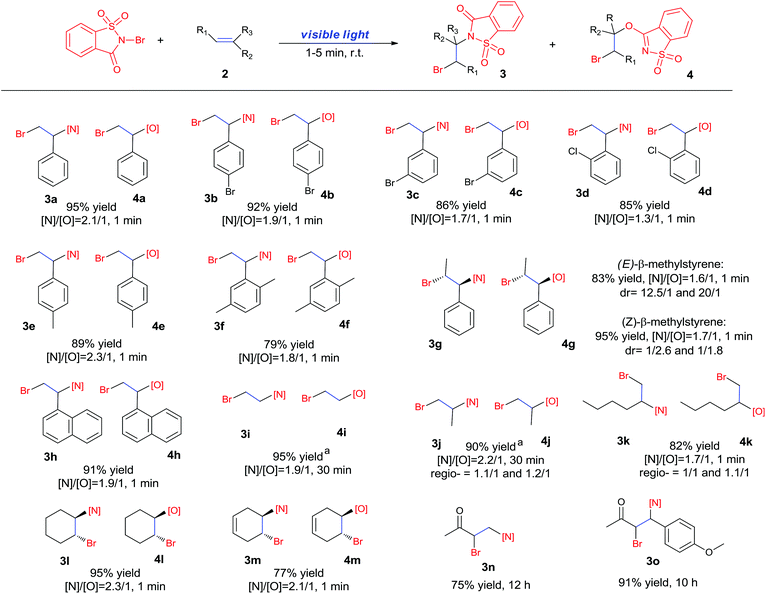 |
| Scheme 2 Substrate scope of visible-light promoted bromofunctionalization with NBSA. The general conditions: alkenes (1.1 equiv.), N-bromosaccharin (NBSA, 0.2 mmol), CH2Cl2 (1.0 mL), r.t., and ambient light. Yields and selectivities of the isolated products are based on the limiting NBSA. aThe reaction was carried out with an alkene balloon. | |
The reaction also worked well with aliphatic alkenes. In particular, industrial olefins such as ethylene and propene worked well in the reactions to give the bromoamidation and bromoetherification products in high yields in 30 min with a balloon (3i–3j, 4i–4j), which is much faster than the reaction catalyzed by Lewis acid.8 The reaction with ethylene can be conducted in the gram scale (5 mmol) with a similar performance (12 h, 91% yield, [N]/[O] ratio 2
:
1). In the cases with 1-hexene, bromoamidation and bromoetherification products were also isolated in high yields (3k, 4k). Cyclic alkenes such as cyclohexene and 1,4-cyclohexadiene could be readily converted to the corresponding products in high yields (3l–3m, 4l–4m). It is notable that the reaction was also applied to electron-deficient enones such as chalcone and methyl vinyl ketone to give single bromoamidation adducts (3n–3o) in high isolated yields, albeit with lower reactivity. In all these cases examined (Scheme 2), the amidation and etherification adducts were obtained in ca. 2
:
1 ratio. In addition, regioisomers involving N/O– and X addition to double bonds have been observed in the reactions of linear olefins such as propene and 1-hexene.
N-Chlorosaccharin (NCSA) was also examined in the reaction to generate chlorofunctionalization products. To our delight, the reaction could proceed rapidly with styrenes, aliphatic alkenes and cyclic alkenes to give the chloroamidation and chloroetherification products (Table 1, entries 1–4, 7 and 8). In particular, for ethylene and propene, the corresponding products (5f–5g, 6f–6g) were achieved in high yields in 1 hour, to note that these industrial olefins were not applicable in the Lewis acid catalyzed reaction.8 When (E)-β-methylstyrene and (Z)-β-methylstyrene were applied, chloroamine 5e and chloroether 6e were obtained in high yields, but with poor diastereoselectivity (anti/syn 1.7/1, and 1.8/1 ratios for (E)-isomer and 1/3.5, and 1/2.8 ratios for (Z)-isomer). The low diastereoselectivity in these cases is highly suggestive of a radical process rather than a cationic process.
Table 1 Substrate scope of visible-light promoted chlorofunctionalization with NCSAa
Recently, the development of direct C–H amination of alkenes has been of significant synthetic interest and most of the advancements are based on transition metal catalysed processes.9 On the basis of the mild halofunctionalization above, we reasoned that sequential halofunctionalization and elimination may readily lead to an imidation product, thus formulating a one pot protocol for direct C–H imidation of alkenes. When we treated styrene and NBSA in the presence of DBU, clean imidation was observed, giving the corresponding imidation product 7a in 68% yield (Scheme 3). Meanwhile, acetophenone was obtained as a minor product which should result from the bromoetherification adduct. Encouragingly, aryl olefins substituted with electron-donating and withdrawing groups could be readily converted to the corresponding vinyl amides with 57–69% isolated yields (Scheme 4, 7b–7f). To our delight, the reaction of internal aryl alkenes also worked well to give imidation adducts in moderate yields (7g–7i). Aliphatic alkenes have also been attempted, showing unfortunately no imidation adducts.
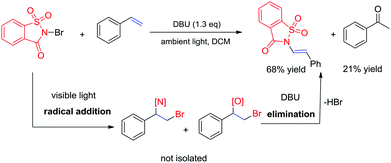 |
| Scheme 3 Imidation of NBSA and styrene. | |
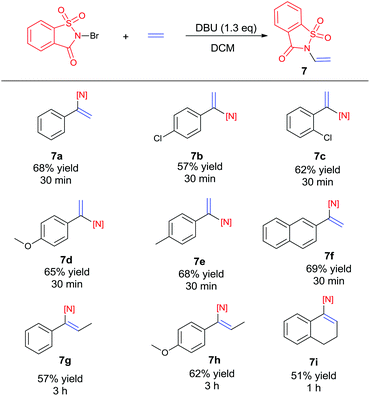 |
| Scheme 4 Substrate scope of visible-light promoted bromofunctionalization with NBSA. N-bromosaccharin (NBSA, 0.2 mmol), alkene (1.1 equiv.), DBU (1.3 eq.), CH2Cl2 (4.0 mL), r.t., ambient light. Isolated yield. | |
Mechanistically, the reactions are proposed to occur via a homolytic radical addition pathway (Scheme 5). On the basis of previous reports, the reaction is initiated by visible light photolysis of NBSA to generate saccharin imidyl radicals and bromo radicals.7 In this case, imidyl radicals may participate in radical addition as either N-centred or O-centred radicals and such a dual N/O radical character is known for succinic imide radical species.6,10 Bromo radical addition to styrene leads to a radical intermediate Int-1, which was quenched by N radicals or O radicals to give the halofunctionalization products. Given the fast reaction nature, a radical chain is likely involved in the continuous supply of bromo and N/O radical species after photolysis.
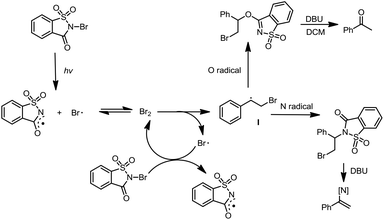 |
| Scheme 5 Proposed radical mechanism. | |
We further studied the photolytic nature of the reaction by in situ monitoring the reaction via in situ IR. No reaction was observed in the dark and the reaction was started instantly by exposure to light, which completed in 1 min to give the bromoamide and bromoether products (Fig. 2). The halofunctionalization adducts derived from aromatic alkenes undergo facile base assisted elimination to afford vinylated adducts in one pot and control experiments verified this conclusion (ESI, Scheme 2†).
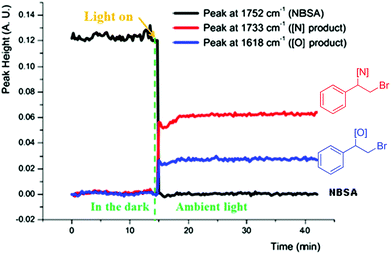 |
| Fig. 2 Light responsive behaviours by in situ monitoring the reaction. | |
Conclusions
In conclusion, we have developed an efficient visible-light promoted intermolecular halofunctionalization and amidation of alkenes using N-bromosaccharin as both the nitrogen and halogen source. N-Bromo and chlorosaccharins, endowed with a weakened N–X bond, are amenable to visible light promoted homolytic cleavage, facilitating reliable and controllable radical addition reactions. A broad range of olefins, including ethylene, propene as well as other simple olefins, were tolerated to give haloamidation and haloetherification products in high yields. A one pot radical addition–elimination protocol was also developed to afford vinyl amide products, formulating a formal direct C–H imidation of aryl alkenes, particularly internal alkenes, with moderate yields and good regioselectivity.
Acknowledgements
We thank the Natural Science Foundation of China (21390400, 21521002 and 21202171) and the National Basic Research Program of China (2011CB808600) for financial support.
References
-
J. E. G. Kemp, in Comprehensive Organic Synthesis, ed. B. M. Trost and I. Fleming, Pergamon, Oxford, 1991, vol. 7, pp. 469–513 CrossRef CAS;
W. H. Pearson, B. W. Lian and S. C. Bergmeier, in Comprehensive Heterocyclic Chemistry II, ed. A. Padwa, Pergamon Press, Oxford, 1996, vol. 1A, pp. 1–60 CrossRef CAS;
G. Thomas, Medicinal Chemistry: An Introduction, John Wiley & Sons, New Yok, 2000 CrossRef CAS; J. Qui and R. B. J. Silverman, Med. Chem., 2000, 43, 706 CrossRef CAS; T. Mukai, M. Hagimori, K. Arimitsu, T. Katoh, M. Ukon, T. Kajimoto, H. Kimura, Y. Magata, E. Miyoshi, N. Taniguchi, M. Node and H. Saji, Bioorg. Med. Chem., 2011, 19, 4312 CrossRef PubMed; S. R. Chemler and M. T. Bovino, ACS Catal., 2013, 3, 1076 CrossRef PubMed; D. J. Aberhart, S. J. Gould, H. J. Lin, T. K. Thiruvengadam and B. H. Weiller, J. Am. Chem. Soc., 1983, 105, 5461 CrossRef; N. O. Ilchenko, M. A. Cortés and K. J. Szabó, ACS Catal., 2016, 6, 447 CrossRef.
- Reactions occur via halogenium ions: M. S. Kharasch and H. M. Priestley, J. Am. Chem. Soc., 1939, 61, 3425 CrossRef CAS; J. R. Shelton and C. Cialdella, J. Org. Chem., 1958, 23, 1128 CrossRef; L. H. Zalkow and C. D. Kennedy, J. Org. Chem., 1964, 29, 1290 CrossRef; T. P. Seden and R. W. Turner, J. Chem. Soc. C, 1968, 876 RSC; H. Tera, S. Zawadzki and A. Zwierzak, Tetrahedron, 1981, 37, 2675 CrossRef; A. Klepacz and A. Zwierzak, Tetrahedron Lett., 2001, 42, 4539 CrossRef; W. Z. Yu, F. Chen, Y. A. Cheng and Y.-Y. Yeung, J. Org. Chem., 2015, 80, 2815 CrossRef PubMed.
- Reactions occur via aziridinium ions: G. Li, H.-X. Wei, S. H. Kim and M. Neighbors, Org. Lett., 1999, 1, 395 CrossRef CAS; G. Li, H.-X. Wei and S. K. Kim, Org. Lett., 2000, 2, 2249 CrossRef PubMed; H.-X. Wei, S. H. Kim and G. Li, Tetrahedron, 2001, 57, 3869 CrossRef; T. H. Chuang and K. B. Sharpless, Org. Lett., 2000, 2, 3555 CrossRef PubMed; G. Li, H.-X. Wei, S. H. Kim and M. D. Carducci, Angew. Chem., Int. Ed., 2001, 40, 4277 CrossRef; H.-X. Wei, S. H. Kim and G. Li, J. Org. Chem., 2002, 67, 4777 CrossRef PubMed; H.-X. Wei, S. Siruta and G. Li, Tetrahedron Lett., 2002, 43, 3809 CrossRef; D. Chen, C. Timmons, H.-X. Wei and G. Li, J. Org. Chem., 2003, 68, 5742 CrossRef PubMed; W. Pei, C. Timmons, X. Xu and G. Li, Org. Biomol. Chem., 2003, 1, 2919 Search PubMed; G. Li, K. S. R. S. Saibabu and C. Timmons, Eur. J. Org. Chem., 2007, 2745 CrossRef.
- J.-F. Wei, L.-H. Zhang, Z.-G. Chen, X.-Y. Shi and J.-J. Cao, Org. Biomol. Chem., 2009, 7, 3280 Search PubMed; J.-F. Wei, Z.-G. Chen, W. Lei, L.-H. Zhang, M.-Z. Wang, X.-Y. Shi and R.-T. Li, Org. Lett., 2009, 11, 421 CrossRef CAS PubMed; J. S. Yadav, B. V. S. Reddy, D. N. Chary and D. Chandrakanth, Tetrahedron Lett., 2009, 50, 1136 CrossRef; X. Ji, H. Mei, Y. Qian, J. Han, G. Li and Y. Pan, Synthesis, 2011, 3680 Search PubMed; H. Mei, J. Han, G. Li and Y. Pan, RSC Adv., 2011, 1, 429 RSC; S. E. Denmark, D. Kalyani and W. R. Collins, J. Am. Chem. Soc., 2010, 132, 15752 CrossRef PubMed; D. W. Tay, I. T. Tsoi, J. C. Er, G. Y. C. Leung and Y.-Y. Yeung, Org. Lett., 2013, 15, 1310 CrossRef PubMed; Z. Wang, Y. Zhang, H. Fu, Y. Jiang and Y. Zhao, Synlett, 2008, 2667 Search PubMed; G. Li, H.-X. Wei and S. H. Kim, Tetrahedron, 2001, 57, 8407 CrossRef; J.-L. Han, S.-J. Zhi, L.-Y. Wang, Y. Pan and G. Li, Eur. J. Org. Chem., 2007, 1332 CrossRef; H. Sun, J. Han, P. V. Kattamuri, Y. Pan and G. Li, J. Org. Chem., 2013, 78, 1171 CrossRef PubMed; Y. Cai, X. Liu, Y. Hui, J. Jiang, W. Wang, W. Chen, L. Lin and X. Feng, Angew. Chem., Int. Ed., 2010, 49, 6160 CrossRef PubMed; Y. Cai, X. Liu, J. Jiang, W. Chen, L. Lin and X. Feng, J. Am. Chem. Soc., 2011, 133, 5636 CrossRef PubMed; A. Alix, C. Lalli, P. Retailleau and G. Masson, J. Am. Chem. Soc., 2012, 134, 10389 CrossRef PubMed; V. V. Thakur, S. K. Talluri and A. Sudalai, Org. Lett., 2003, 5, 861 CrossRef PubMed; S. Minakata, Y. Yoneda, Y. Oderaotoshi and M. Komatsu, Org. Lett., 2006, 8, 967 CrossRef PubMed; J. Hayakawa, M. Kuzuhara and S. Minakata, Org. Biomol. Chem., 2010, 8, 1424 Search PubMed; S. Minakata and J. Hayakawa, Chem. Commun., 2011, 47, 1905 RSC; Y.-Y. Yeung, X. Gao and E. J. Corey, J. Am. Chem. Soc., 2006, 128, 9644 CrossRef PubMed; L. Zhou, J. Zhou, C. K. Tan, J. Chen and Y.-Y. Yeung, Org. Lett., 2011, 13, 2448 CrossRef PubMed.
- U. Lüning and A. Kirsch, Chem. Ber., 1993, 126, 1171 CrossRef CAS; Q. Qin, D. Ren and S. Yu, Org. Biomol. Chem., 2015, 13, 10295 Search PubMed ; Photoredox catalytic 1,2-functionalization: Y. Yasu, T. Koike and M. Akita, Angew. Chem., Int. Ed., 2012, 51, 9567 CrossRef PubMed; R. Tomita, Y. Yasu, T. Koike and M. Akita, Angew. Chem., Int. Ed., 2014, 53, 7144 CrossRef PubMed; K. Miyazawa, T. Koike and M. Akita, Chem. – Eur. J., 2015, 21, 11677 CrossRef PubMed; Y. Yasu, T. Koike and M. Akita, Org. Lett., 2013, 15, 2136 CrossRef PubMed; Y. Yasu, Y. Arai, R. Tomita, T. Koike and M. Akita, Org. Lett., 2014, 16, 780 CrossRef PubMed; M. H. Keylor, J. E. Park, C.-J. Wallentin and C. R. J. Stephenson, Tetrahedron, 2014, 70, 4264 CrossRef; J. D. Nguyen, J. W. Tucker, M. D. Konieczynska and C. R. J. Stephenson, J. Am. Chem. Soc., 2011, 133, 4160 CrossRef PubMed; J. W. Tucker, J. D. Nguyen, J. M. R. Narayanam, S. W. Krabbe and C. R. J. Stephenson, Chem. Commun., 2010, 46, 4985 RSC; C.-J. Wallentin, J. D. Nguyen, P. Finkbeiner and C. R. J. Stephenson, J. Am. Chem. Soc., 2012, 134, 8875 CrossRef PubMed; E. Kim, S. Choi, H. Kim and E. J. Cho, Chem. – Eur. J., 2013, 19, 6209 CrossRef PubMed; S. H. Oh, Y. R. Malpani, N. Ha, Y. S. Jung and S. B. Han, Org. Lett., 2014, 16, 1310 CrossRef PubMed; D. B. Bagal, G. Kachkovskyi, M. Knorn, T. Rawner, B. M. Bhanage and O. Reiser, Angew. Chem., Int. Ed., 2015, 54, 6999 CrossRef PubMed.
- J. C. Day, M. G. Katsaros, W. D. Kocher, A. E. Scott and P. S. Skell, J. Am. Chem. Soc., 1978, 100, 1950 CrossRef CAS; U. Lüning, D. S. McBain and P. S. Skell, J. Org. Chem., 1986, 51, 2077 CrossRef.
- L. Song, L. Zhang, S. Luo and J.-P. Cheng, Chem. – Eur. J., 2014, 20, 14231 CrossRef CAS PubMed.
- L. Song, S. Luo and J.-P. Cheng, Org. Lett., 2013, 15, 5702 CrossRef CAS PubMed.
- T. Hosokawa, M. Takano, Y. Kuroki and S.-I. Murahashi, Tetrahedron Lett., 1992, 33, 6643 CrossRef CAS; J. M. Lee, D.-S. Ahn, D. Y. Jung, J. Lee, Y. Do, S. K. Kim and S. Chang, J. Am. Chem. Soc., 2006, 128, 12954 CrossRef PubMed; X. Ji, H. Huang, W. Xiong, K. Huang, W. Wu and H. Jiang, J. Org. Chem., 2014, 79, 7005 CrossRef PubMed; Y. Yuan, W. Hou, D. Zhang-Negrerie, K. Zhao and Y. Du, Org. Lett., 2014, 16, 5410–5413 CrossRef PubMed; V. Timokhin, N. R. Anastasi and S. S. Stahl, J. Am. Chem. Soc., 2003, 125, 12996 CrossRef PubMed; V. Timokhin and S. S. Stahl, J. Am. Chem. Soc., 2005, 127, 17888 CrossRef PubMed; J. L. Brice, J. E. Harang, V. Timokhin, N. R. Anastasi and S. S. Stahl, J. Am. Chem. Soc., 2005, 127, 2868 CrossRef PubMed; M. M. Rogers, V. Kotov, J. Chatwichien and S. S. Stahl, Org. Lett., 2007, 9, 4331 CrossRef PubMed; D. Takeda, K. Hirano, T. Satoh and M. Miura, Org. Lett., 2013, 15, 1242 CrossRef PubMed; G. Wu and W. Su, Org. Lett., 2013, 15, 5278 CrossRef PubMed; T. W. Liwosz and S. R. Chemler, Chem. – Eur. J., 2013, 19, 12771 CrossRef PubMed; Y. H. Jang and S. W. Youn, Org. Lett., 2014, 16, 3720 CrossRef PubMed; Y.-L. Li, J. Li, A.-L. Ma, Y.-H. Huang and J. Deng, J. Org. Chem., 2015, 80, 3841 CrossRef PubMed.
- P. S. Skell and J. C. Day, J. Am. Chem. Soc., 1978, 100, 1951 CrossRef CAS; A. Kumar and M. D. Sevilla, J. Phys. Chem. B, 2013, 117, 11623 CrossRef PubMed; B. Han, X.-L. Yang, R. Fang, W. Yu, C. Wang, X.-Y. Duan and S. Liu, Angew. Chem., Int. Ed., 2012, 51, 8816 CrossRef PubMed; X.-Y. Duan, X.-L. Yang, R. Fang, X.-X. Peng, W. Yu and B. Han, J. Org. Chem., 2013, 78, 10692 CrossRef PubMed.
|
This journal is © the Partner Organisations 2016 |