DOI:
10.1039/C5QO00352K
(Research Article)
Org. Chem. Front., 2016,
3, 184-189
Synthesis of isoxazoline-featured oxindoles by iminoxyl radical-promoted cascade oxyalkylation/alkylarylation of alkenes†
Received
5th November 2015
, Accepted 9th December 2015
First published on 11th December 2015
Abstract
A novel, selective and metal-free iminoxyl radical-promoted cascade oxyalkylation/alkylarylation of unactivated alkenes and activated alkenes via tandem O–C/C–C/C–C bond formation was developed. By using this protocol, isoxazoline-featured oxindoles were successfully synthesized by a one-pot reaction using β,γ-unsaturated ketoximes and N-arylacrylamides as readily accessible substrates and TBHP as the commercially available oxidant.
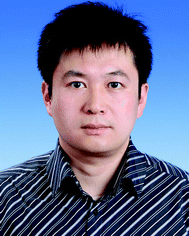 Bing Han | Bing Han obtained his BSc degree from Northwest Normal University in 2000, and then he moved to Lanzhou University and received his PhD degree in 2006 under the supervision of Prof. Zhong-Li Liu. After postdoctoral work with Prof. Jay K. Kochi at the University of Houston, USA, he started his independent research at Lanzhou University in 2008. Bing Han was selected in “The MOE New Century Excellent Talents” (2013) and was a winner of “The National Science Fund for Excellent Young Scholars” (2014). His current research interests are radical chemistry and physical organic chemistry. |
Introduction
Oxindoles are ubiquitous nitrogen heterocycles widely found in the core structure of natural alkaloids and bioactive molecules.1 As a result, the development of new methods for their facile synthesis has been an active area of research.2 Among these strategies for the synthesis of various functionalized oxindoles, those utilizing the vicinal difunctionalization of activated alkenes from N-arylacrylamides through a process of radical species addition followed by intramolecular aromatic homolytic substitution3 have been well developed.4 In these reports, a wide variety of functional groups, such as CF3,5 N3,6 NO2,7 aryl8 and alkyl,9 have been successfully introduced into the oxindole skeleton. For example, in 2013, the Li group developed a Fe-catalysed oxidative alkylarylation of N-arylacrylamides with a sp3 C–H bond adjacent to the heteroatom and an aryl sp2 C–H.10 You and Zhu described Cu and Fe-catalysed 1,2-cyanoalkylarylation of N-arylacrylamides for the construction of cyano-substituted oxindoles, respectively.11
Although these advances have been made in recent years, introduction of bioactive nitrogen-containing heterocycles in such a process has been rarely reported. Isoxazolines, for instance, represent a large class of nitrogen-containing heterocycles which possess attractive biochemical and pharmaceutical properties,12 have never been involved in the synthesis of functionalized oxindoles. Given that these two classes of five-membered nitrogen-containing heterocycles exhibit important functions in pharmaceuticals, the incorporation of isoxazoline into the oxindole skeleton through a radical-participated tandem reaction is of great interest for chemists and pharmacists.
An iminoxyl radical is a fascinating heteroatom-centered radical, however, it has not received the attention it deserves and its considerable synthetic potential has remained largely unappreciated.13,14 Very recently, we developed an iminoxyl radical-promoted cascade cyclization/addition/cyclization strategy taking advantage of its highly synthetic efficiency and less environmental impact15 for the synthesis of isoxazoline-functionalized phenanthridines by the reaction of β,γ-unsaturated ketoximes with 2-arylisonitriles (Scheme 1, up).14b In our continuous interest in the iminoxyl radical-participated tandem reaction, we anticipated that this strategy is also suitable for the synthesis of structurally novel isoxazoline-featured oxindoles by the reaction of β,γ-unsaturated ketoximes with N-arylacrylamides under oxidative conditions involving oxyalkylation/alkylarylation of both activated and unactivated alkenes through tandem O–C/C–C/C–C bond formation (Scheme 1, down).
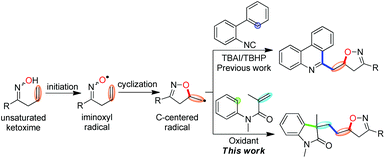 |
| Scheme 1 Iminoxyl radical-promoted cascade oxyalkylation/alkylarylation of alkenes. | |
Results and discussion
To test the hypothesis, the reaction of 2,2-dimethyl-1-phenylbut-3-en-1-one oxime (1a, 1.2 mmol) with N-methyl-N-phenylmethacrylamide (2a, 0.4 mmol) was carried out at 100 °C under oxidative conditions. Indeed, the reaction took place and gave the desired product isoxazoline-featured oxindole 3aa when a variety of oxidants were used. It can be clearly seen from Table 1 that TBHP (tert-butyl hydroperoxide) was more efficient than several other oxidants such as DCP (dicumyl peroxide), DTBP (di-tert-butyl peroxide), TBPB (tert-butyl peroxybenzoate), and K2S2O8 (Table 1, entries 1–6). Meanwhile, TBHP in decane gave a higher yield of the desired product than TBHP in aqueous solution (Table 1, entries 5 and 6). In addition, the high temperature (120 °C) and excess amount of TBHP (4.0 equiv.) did not give better results (Table 1, entries 7 and 8). The addition of solvent in the reaction would decrease the yield of 3aa indicating that the high concentration of substrates was essential for an efficient multi-step cascade reaction (Table 1, entry 9). When the usage amount of ketoxime 1a was reduced to 2 equivalents, the yield of 3aa was a little bit lower (Table 1, entries 10 and 11). Moreover, when the equivalent ratio of 1a to 2a was counterchanged to 1
:
3, the reaction only gave 3aa in 57% yield (Table 1, entry 12).
Table 1 Optimization of the reaction conditionsa

|
Entry |
Oxime (equiv.) |
Oxidant (equiv.) |
Solvent |
Temp (°C) |
Yieldb (%) |
Reaction conditions: 1a (1.2 mmol, 3.0 equiv.), 2a (0.4 mmol, 1.0 equiv.), oxidant (1.2 mmol, 3.0 equiv.), solvent (2 mL) if necessary, sealed tube, Ar, 48 h.
Yield of the isolated product.
TBHP (70% aqueous).
TBHP (5–6 M in decane).
Yield based on oxime. DTBP = di-tert-butyl peroxide, DCP = dicumyl peroxide, TBPB = tert-butyl peroxybenzoate, TBHP = tert-butyl hydroperoxide.
|
1 |
3 |
DCP (3) |
Neat |
100 |
48 |
2 |
3 |
DTBP(3) |
Neat |
100 |
32 |
3 |
3 |
TBPB (3) |
Neat |
100 |
65 |
4 |
3 |
K2S2O8(3) |
CH3CN |
100 |
60 |
5 |
3 |
TBHPc (3) |
Neat |
100 |
80 |
6
|
3
|
TBHP
(3)
|
Neat
|
100
|
88
|
7 |
3 |
TBHPd (3) |
Neat |
120 |
80 |
8 |
3 |
TBHPd (4) |
Neat |
100 |
69 |
9 |
3 |
TBHPd (3) |
PhCl |
100 |
69 |
10 |
2 |
TBHPd (3) |
Neat |
100 |
75 |
11 |
2 |
TBHPd (4) |
Neat |
100 |
64 |
12 |
0.33 |
TBHPd (3) |
Neat |
100 |
57e |
Substrate scope
With the optimized conditions in hand (Table 1, entry 6), a variety of β,γ-unsaturated ketoximes 1 were allowed to react with N-methyl-N-phenylmethacrylamide 2a and the results are illustrated in Table 2. Terminal alkene participated ketoximes reacted smoothly and gave the desired isoxazoline-featured oxindoles in moderate to good yields. Phenyls with a wide range of electronic properties substituted ketoximes were all tolerated well in the tandem reaction delivering the corresponding products in good yields (3ba–3da). Notably, in the case of p-CN-phenyl substituted ketoxime 1b, the obtained diastereoisomers could be separated as 3ba and 3ba′ in a ratio of 1
:
1. The structure of 3ba was confirmed by a single-crystal X-ray diffraction study (Fig. 1). Thiophene substituted β,γ-unsaturated ketoxime could also be used as the cascade partner with 2a to produce the desired product 3ea in 76% yield. In addition, the aliphatic included β,γ-unsaturated ketoxime also afforded the product 3fa in 60% yield. The tandem reaction involving 1,1-disubstituted alkenes was also successful, as demonstrated in the case of 3ga. However, when β,γ-unsaturated ketoxime 1h, in which the gem-dimethyl group was removed from the α-position, was involved in the reaction, the corresponding product 3ha was obtained in a remarkably lower yield. The result reveals that a gem-dimethyl group is necessary for an efficient reaction in this tandem process due to the gem-dimethyl effect (also called as Thorpe–Ingold effect).16
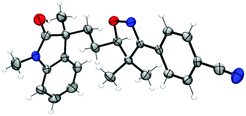 |
| Fig. 1 X-ray structure of 3ba (thermal ellipsoids are shown with 30% probability). | |
Table 2 Scope of β,γ-unsaturated ketoximesa,b,c
Reaction conditions: ketoximes 1 (1.2 mmol, 3.0 equiv.), N-aryl-N-methyl methacrylamide 2a (0.4 mmol, 1.0 equiv.) and TBHP (1.2 mmol, 3.0 equiv., about 0.24 mL, 5–6 M in decane), 100 °C, Ar, 24–48 h.
Yields of isolated products.
The diastereoisomeric ratios were determined by 1H NMR spectroscopy.
|
|
Gratifyingly, when γ,δ-unsaturated ketoxime 4 was allowed to react with 2a under the optimized reaction conditions, the cyclic nitrone-featured oxindole 5 and its diastereoisomer 5′ were obtained in a combined yield of 40%. The ratio of 5 to 5′ was 1
:
1. Such an outcome suggested that γ,δ-unsaturated ketoxime-derived iminoxyl radicals are sensitive to N-atom 5-exo-trig cyclization17 rather than O-atom 6-exo-trig cyclization, which is consistent with our previous observations.14 Therefore, the present protocol is also available for the preparation of cyclic nitrone-functionalized oxindole derivatives (Scheme 2).
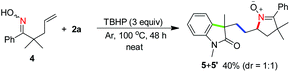 |
| Scheme 2 γ,δ-Unsaturated ketoxime-participated cascade sequence with 2a. | |
Having successfully achieved the cascade sequence with unsaturated ketoximes, the scope of the N-arylacrylamides 2 was also explored by reacting with oxime 1a under the optimal reaction conditions and the results are summarized in Table 3. N-Phenyl with a wide range of electronic properties at the para- and ortho-position substituted acrylamides all proceeded well in the reaction to yield the desired isoxazoline-featured oxindoles in moderate to excellent yields (3ab–3ag). When the substituent groups were at the ortho-position of the phenyl ring, the effect of the steric hindrance was very distinct and it produced relatively lower yields (3ae–3ag). N-Arylacrylamides bearing phenyl and benzyl as the N-protecting groups provided moderate yields of the corresponding isoxazoline-featured oxindoles 3ai and 3aj in 52% and 51% yields, respectively. However, N-free arylacrylamide hardly gave the desired product 3ah, which indicated that the N-protecting group was essential in an oxidative environment. In addition, a series of N-arylacrylamides bearing different functional groups at the 2-position of the acrylamide moiety, such as phenyl, benzyl, and ester groups could still provide the desired products in moderate to good yields (3ak–3am). Moreover, cyclization of the tetrahydroquinoline derivative furnished the tricyclic oxindole 3an in 59% yield. Finally, when 2o was employed as a substrate, the six-membered ring product 3ao was obtained in 37% yield.
Table 3 Scope of N-arylacrylamides 2a,b,c
Reaction conditions: ketoxime 1a (1.2 mmol, 3.0 equiv.), N-arylacrylamides 2 (0.4 mmol, 1.0 equiv.) and TBHP (1.2 mmol, 3.0 equiv., about 0.24 mL, 5–6 M in decane), 100 °C, Ar, 24–48 h.
Yields of isolated products.
The diastereoisomeric ratios were determined by 1H NMR spectroscopy.
|
|
To further investigate the applicability of this protocol, N-methyl-N-phenylphenylpropiolamide 6 was prepared as an alkyne-incorporated counterpart and tested in the reaction.18 Indeed, when aryl and thiophene substituted β,γ-unsaturated ketoximes were allowed to react with compound 6, isoxazoline-featured azaspiro[4.5]trienones (7a–7d) were obtained in 23–48% yields (Scheme 3). The structure of 7a was confirmed by a single-crystal X-ray diffraction study (Fig. 2). Apparently, the cascade reactions underwent an iminoxyl radical-promoted cyclization/addition/spirocyclization/oxygenation process through tandem O–C/C–C/C–C/C–O bond formation involving both alkenes and alkynes (see the ESI, Scheme S1† for the proposed mechanism).
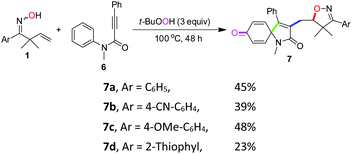 |
| Scheme 3 Radical tandem reaction of β,γ-unsaturated ketoximes 1 with N-arylpropiolamides 6. | |
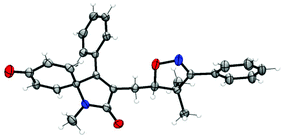 |
| Fig. 2 X-ray structure of 7a (thermal ellipsoids are shown with 30% probability). | |
Mechanism discussion
To gain further understanding about the reaction mechanism, by-product isolation and control experiments were conducted as shown in Scheme 4. When the model reaction was carried out, a part of ketoxime 1a was incorporated into the desired product 3aa in the form of isoxazoline and nearly half of the other was converted into isoxazoline 8 as the obtainable by-product via intramolecular hydroxygenation of alkenes (Scheme 4, eqn (1)). Meanwhile, the reaction of isoxazoline 8 with N-methyl-N-phenyl methacrylamide 2a could not afford the desired product 3aa under the standard conditions (Scheme 4, eqn (2)). Notably, the addition of a stoichiometric amount of radical scavenger TEMPO (2,2,6,6-tetramethyl-1-piperidinyloxy) to the model reaction resulted in the TEMPO-trapped isoxazoline 9 in 98% yield with the desired product 3aa undetected under the standard conditions (Scheme 4, eqn (3)). These results demonstrated clearly that the initiation step is the generation of the iminoxyl radical and an iminoxyl radical-promoted tandem cyclization/addition/cyclization mechanism should be exclusive.
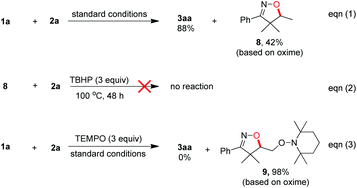 |
| Scheme 4 By-product isolation and control experiments. | |
Based on our observations and aforementioned mechanistic studies, a plausible mechanism is proposed in Scheme 5. Initially, the homolysis of TBHP is carried out by heating to generate the t-BuO radical and OH radical. The t-BuO radical or OH radical then abstracts an H-atom of β,γ-unsaturated ketoxime 1 to yield the corresponding iminoxyl radical I, and then the latter undergoes fast 5-exo-trig cyclization to yield the transient C-centered radical II. The reduction of C-centered radical II by oxime 1 gives isoxazoline 8 as the by-product, on the other hand, the intermolecular addition of radical II to the alkene moiety of substrate 2 provides intermediate III which subsequently undergoes intramolecular cyclization to give the intermediate IV. Finally, the isoxazoline-featured oxindole 3 is obtained by further oxidative aromatization of the intermediate IV by using TBHP.
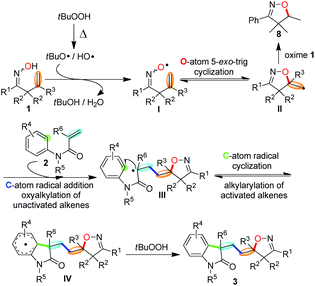 |
| Scheme 5 Proposed mechanism for the iminoxyl radical-promoted cascade oxyalkylation/alkylarylation of alkenes. | |
Conclusions
In conclusion, we have developed a novel, selective and metal-free iminoxyl radical-promoted cascade oxyalkylation/alkylarylation of unactivated/activated alkenes through a tandem intra-/intermolecular C–O/C–C/C–C bond formation process. By using this protocol, the structurally novel isoxazoline-featured oxindoles were successfully synthesized by a one-pot reaction using β,γ-unsaturated ketoximes and N-arylacrylamides as the readily accessible substrates and TBHP as the commercially available oxidant. Further studies on the iminoxyl radical promoted reaction are in progress in our laboratory.
Acknowledgements
We thank the NNSFC (21422205, 21272106), the Program for New Century Excellent Talents in University (NCET-13-0258), the “111” project, and the Fundamental Research Funds for the Central Universities (lzujbky-2014-k03, lzujbky-2014-60) for the financial support.
References
-
(a) B. M. Trost and M. K. Brennan, Synthesis, 2009, 3003 CrossRef CAS;
(b) B. S. Jensen, CNS Drug Rev., 2002, 8, 353 CrossRef CAS PubMed;
(c) C. V. Galliford and K. A. Scheidt, Angew. Chem., Int. Ed., 2007, 46, 8748 CrossRef CAS PubMed;
(d) G. S. Singh and Z. Y. Desta, Chem. Rev., 2012, 112, 6104 CrossRef CAS PubMed.
- For selected reviews, see:
(a) C. Marti and E. M. Carreira, Eur. J. Org. Chem., 2003, 2209 CrossRef CAS;
(b) J. E. M. N. Klein and R. J. K. Taylor, Eur. J. Org. Chem., 2011, 6821 CrossRef CAS. For selected example, see:
(c) Y.-X. Jia and E. P. Kündig, Angew. Chem., Int. Ed., 2009, 48, 1636 CrossRef CAS PubMed;
(d) S. Jaegli, W. Erb, P. Retailleau, J.-P. Vors, L. Neuville and J. Zhu, Chem. – Eur. J., 2010, 16, 5863 CrossRef CAS PubMed;
(e) G. An, W. Zhou, G. Zhang, H. Sun, J. Han and Y. Pan, Org. Lett., 2010, 12, 4482 CrossRef CAS PubMed;
(f) T. Wu, X. Mu and G. Liu, Angew. Chem., Int. Ed., 2011, 50, 12578 CrossRef CAS PubMed;
(g) X. Mu, T. Wu, H.-Y. Wang, Y.-L. Guo and G. Liu, J. Am. Chem. Soc., 2012, 134, 878 CrossRef CAS PubMed.
- For reviews, see:
(a) W. R. Bowman and J. M. D. Storey, Chem. Soc. Rev., 2007, 36, 1803 RSC;
(b) A. Studer and D. P. Curran, Angew. Chem., Int. Ed., 2011, 50, 5018 CrossRef CAS PubMed.
- For selected reviews on tandem radical cyclization of N-arylacrylamides, see:
(a) R.-J. Song, Y. Liu, Y.-X. Xie and J.-H. Li, Synthesis, 2015, 1195 CAS;
(b) J.-R. Chen, X.-Y. Yu and W.-J. Xiao, Synthesis, 2015, 604 Search PubMed. For selected examples, see:
(c) Y.-M. Li, M. Sun, H.-L. Wang, Q.-P. Tian and S.-D. Yang, Angew. Chem., Int. Ed., 2013, 52, 3972 CrossRef CAS PubMed;
(d) H. Wang, L.-N. Guo and X.-H. Duan, Chem. Commun., 2013, 49, 10370 RSC;
(e) M.-B. Zhou, R.-J. Song, X.-H. Ouyang, Y. Liu, W.-T. Wei, G.-B. Deng and J.-H. Li, Chem. Sci., 2013, 4, 2690 RSC;
(f) W. Kong, M. Casimiro, E. Merino and C. Nevado, J. Am. Chem. Soc., 2013, 135, 14480 CrossRef CAS PubMed;
(g) W. Kong, M. Casimiro, N. Fuentes, E. Merino and C. Nevado, Angew. Chem., Int. Ed., 2013, 52, 13086 CrossRef CAS PubMed;
(h) S.-L. Zhou, L.-N. Guo, H. Wang and X.-H. Duan, Chem. – Eur. J., 2013, 19, 12970 CrossRef CAS PubMed;
(i) M.-B. Zhou, C.-Y. Wang, R.-J. Song, Y. Liu, W.-T. Wei and J.-H. Li, Chem. Commun., 2013, 49, 10817 RSC;
(j) Y. Meng, L.-N. Guo, H. Wang and X.-H. Duan, Chem. Commun., 2013, 49, 7540 RSC;
(k) J.-H. Fan, W.-T. Wei, M.-B. Zhou, R.-J. Song and J.-H. Li, Angew. Chem., Int. Ed., 2014, 53, 6650 CrossRef CAS PubMed;
(l) M.-Z. Lu and T.-P. Loh, Org. Lett., 2014, 16, 4698 CrossRef CAS PubMed;
(m) T. Shen, Y. Yuan, S. Song and N. Jiao, Chem. Commun., 2014, 50, 4115 RSC;
(n) X.-J. Tang, C. S. Thomoson and W. R. Dolbier Jr., Org. Lett., 2014, 16, 4594 CrossRef CAS PubMed;
(o) C.-D. Pan, H.-L. Zhang and C.-J. Zhu, Org. Biomol. Chem., 2015, 13, 361 RSC.
-
(a) W. Wei, J. Wen, D. Yang, X. Liu, M. Guo, R. Dong and H. Wang, J. Org. Chem., 2014, 79, 4225 CrossRef CAS PubMed;
(b) L. Zhang, Z. Li and Z.-Q. Liu, Org. Lett., 2014, 16, 3688 CrossRef CAS PubMed;
(c) L. Shi, X. Yang, Y. Wang, H. Yang and H. Fu, Adv. Synth. Catal., 2014, 356, 1021 CrossRef CAS;
(d) Q. Lu, C. Liu, P. Peng, Z. Liu, L. Fu, J. Huang and A. Lei, Asian J. Org. Chem., 2014, 3, 273 CrossRef CAS.
-
(a) K. Matcha, R. Narayan and A. P. Antonchick, Angew. Chem., Int. Ed., 2013, 52, 7985 CrossRef CAS PubMed;
(b) Y. Yuan, T. Shen, K. Wang and N. Jiao, Chem. – Asian J., 2013, 8, 2932 CrossRef CAS PubMed;
(c) X.-H. Wei, Y.-M. Li, A.-X. Zhou, T.-T. Yang and S.-D. Yang, Org. Lett., 2013, 15, 4158 CrossRef CAS PubMed;
(d) J. Qiu and R. Zhang, Org. Biomol. Chem., 2014, 12, 4329 RSC.
-
(a) Y.-M. Li, X.-H. Wei, X.-A. Li and S.-D. Yang, Chem. Commun., 2013, 49, 11701 RSC;
(b) T. Shen, Y.-Z. Yuan and N. Jiao, Chem. Commun., 2014, 50, 554 RSC;
(c) X.-H. Wei, Q.-X. Wu and S.-D. Yang, Synlett, 2015, 1417 CAS.
-
(a) W. Fu, F. Xu, Y. Fu, M. Zhu, J. Yu, C. Xu and D. Zou, J. Org. Chem., 2013, 78, 12202 CrossRef CAS PubMed;
(b) S. Tang, D. Zhou and Y.-C. Wang, Eur. J. Org. Chem., 2014, 3656 CrossRef CAS.
-
(a) Z. Li, Y. Zhang, L. Zhang and Z.-Q. Liu, Org. Lett., 2014, 16, 382 CrossRef CAS PubMed;
(b) Q. Dai, J.-T. Yu, Y. Jiang, S.-J. Guo, H.-T. Yang and J. Cheng, Chem. Commun., 2014, 50, 3865 RSC;
(c) Y. Liu, J.-L. Zhang, R.-J. Song and J.-H. Li, Org. Chem. Front., 2014, 1, 1289 RSC.
- W.-T. Wei, M.-B. Zhou, J.-H. Fan, W. Liu, R.-J. Song, Y. Liu, M. Hu, P. Xie and J.-H. Li, Angew. Chem., Int. Ed., 2013, 52, 3638 CrossRef CAS PubMed.
-
(a) J. Li, Z.-G. Wang, N.-J. Wu, G. Gao and J.-S. You, Chem. Commun., 2014, 50, 15049 RSC;
(b) C. Pan, H. Zhang and C. Zhu, Org. Biomol. Chem., 2015, 13, 361 RSC.
-
(a) S. Castellano, D. Kuck, M. Viviano, J. Yoo, F. López-Vallejo, P. Conti, L. Tamborini, A. Pinto, J. L. Medina-Franco and G. Sbardella, J. Med. Chem., 2011, 54, 7663 CrossRef CAS PubMed;
(b) K.-H. Hwang, J.-S. Lim, S.-H. Kim, M.-S. Jeon, D.-G. Lee, K.-H. Chung, S.-J. Koo and J.-H. Kim, J. Agric. Food Chem., 2013, 61, 9285 CrossRef CAS PubMed;
(c) S. J. Buhrlage, C. A. Bates, S. P. Rowe, A. R. Minter, B. B. Brennan, C. Y. Majmudar, D. E. Wemmer, H. Al-Hashimi and A. K. Mapp, ACS Chem. Biol., 2009, 4, 335 CrossRef CAS PubMed;
(d) J. B. Sperry and D. Wright, Curr. Opin. Drug Discovery Dev., 2005, 8, 723 CAS.
- For structural properties of iminoxyl radicals, see:
(a) J. R. Thomas, J. Am. Chem. Soc., 1964, 86, 1446 CrossRef CAS;
(b) J. L. Brokenshire, G. D. Mendenhall and K. U. Ingold, J. Am. Chem. Soc., 1971, 93, 5278 CrossRef CAS;
(c) D. A. Pratt, J. A. Blake, P. Mulder, J. C. Walton, H.-G. Korth and K. U. Ingold, J. Am. Chem. Soc., 2004, 126, 10667 CrossRef CAS PubMed, and references therein;
(d) S.-S. Chong, Y. Fu, L. Liu and Q.-X. Guo, J. Phys. Chem. A, 2007, 111, 13112 CrossRef CAS PubMed. For iminoxyl radicals used as the O-centered radicals in reactions, see:
(e) B. M. Eisenhauer, M.-H. Wang, H. Labaziewicz, M. Ngo and G. D. Mendenhall, J. Org. Chem., 1997, 62, 2050 CrossRef CAS PubMed;
(f) Y.-Y. Liu, X.-H. Yang, J. Yang, R.-J. Song and J.-H. Li, Chem. Commun., 2014, 50, 6906 RSC;
(g) Y.-Y. Liu, J. Yang, R.-J. Song and J.-H. Li, Adv. Synth. Catal., 2014, 356, 2913 CrossRef CAS;
(h) I. B. Krylov, A. O. Terent'ev, V. P. Timofeev, B. N. Shelimov, R. A. Novikov, V. M. Merkulova and G. I. Nikishin, Adv. Synth. Catal., 2014, 356, 2266 CrossRef CAS;
(i) X. Zhu, Y.-F. Wang, W. Ren, F.-L. Zhang and S. Chiba, Org. Lett., 2013, 15, 3214 CrossRef CAS PubMed;
(j) F.-L. Zhang, Y.-F. Wang and S. Chiba, Org. Biomol. Chem., 2013, 11, 6003 RSC;
(k) X.-Q. Hu, G.-Q. Feng, J.-R. Chen, D.-M. Yan, Q.-Q. Zhao, Q. Wei and W.-J. Xiao, Org. Biomol. Chem., 2015, 13, 3457 RSC;
(l) D. Shi, H.-T. Qin, C. Zhu and F. Liu, Eur. J. Org. Chem., 2015, 5084 CrossRef CAS;
(m) B. C. Lemercier and J. G. Pierce, Org. Lett., 2015, 17, 4542 CrossRef CAS PubMed;
(n) W.-F. Li, P.-J. Jia, B. Han, D.-J. Li and W. Yu, Tetrahedron, 2013, 69, 3274 CrossRef CAS.
- For iminoxyl radicals used as the O- and N-centered radicals in reactions, see:
(a) B. Han, X.-L. Yang, R. Fang, W. Yu, C. Wang, X.-Y. Duan and S. Liu, Angew. Chem., Int. Ed., 2012, 51, 8816 CrossRef CAS PubMed;
(b) X.-L. Yang, F. Chen, N.-N. Zhou, W. Yu and B. Han, Org. Lett., 2014, 16, 6476 CrossRef CAS PubMed;
(c) X.-X. Peng, Y.-J. Deng, X.-L. Yang, L. Zhang, W. Yu and B. Han, Org. Lett., 2014, 16, 4650 CrossRef CAS PubMed.
- For selected reviews, see:
(a) A. J. McCarroll and J. C. Walton, Angew. Chem., Int. Ed., 2001, 40, 2224 CrossRef CAS;
(b) M. Malacria, Chem. Rev., 1996, 96, 289 CrossRef CAS PubMed;
(c) K. K. Wang, Chem. Rev., 1996, 96, 207 CrossRef CAS PubMed;
(d)
A.-L. Dhimane, L. Fensterbank and M. Malacvia, Polycyclic compounds via radical cascade reactions, in Radicals in Organic Synthesis, ed. P. Renaud and M. P. Sibi, Wiley-VCH, Weinheim, 2001, ch. 4.4, vol. 2, pp. 350–382 Search PubMed;
(e)
H. Togo, Advanced Free Radical Reactions for Organic Synthesis, Elsevier Science, Amsterdam, 2004, pp. 57–156 Search PubMed;
(f) S. Z. Zard, Chem. Soc. Rev., 2008, 37, 1603 RSC;
(g) A. G. Fallis and I. M. Brinza, Tetrahedron, 1997, 53, 17543 CrossRef CAS;
(h) S. Z. Zard, Synlett, 1996, 1148 CrossRef CAS;
(i) J. L. Esker and M. Newcomb, Adv. Heterocycl. Chem., 1993, 58, 1 CrossRef CAS;
(j) L. Stella, Angew. Chem., Int. Ed., 1983, 22, 337 CrossRef;
(k) P. Mackiewicz and R. Furstoss, Tetrahedron, 1978, 34, 3241 CrossRef CAS;
(l) R. S. Neale, Synthesis, 1971, 1 CrossRef CAS.
- For the Thorpe–Ingold effect, see:
(a) R. M. Beesley, C. K. Ingold and J. F. Thorpe, J. Chem. Soc., Trans., 1915, 107, 1080 RSC;
(b) S. M. Bachrach, J. Org. Chem., 2008, 73, 2466 CrossRef CAS PubMed;
(c) R. Salvio, L. Mandolini and C. Savelli, J. Org. Chem., 2013, 78, 7259 CrossRef CAS PubMed;
(d) A. L. Ringer and D. H. Magers, J. Org. Chem., 2007, 72, 2533 CrossRef CAS PubMed.
- For selected examples on the reaction of nitrogen-centered radicals, see:
(a) Z. Li, L. Song and C. Li, J. Am. Chem. Soc., 2013, 135, 4640 CrossRef CAS PubMed;
(b) L. Song, K. Liu and C. Li, Org. Lett., 2011, 13, 3434 CrossRef CAS PubMed;
(c) B. Janza and A. Studer, J. Org. Chem., 2005, 70, 6991 CrossRef CAS PubMed;
(d) K. C. Nicolaou, P. S. Baran, Y.-L. Zhong, S. Barluenga, K. W. Hunt, R. Kranich and J. A. Vega, J. Am. Chem. Soc., 2002, 124, 2233 CrossRef CAS PubMed;
(e) J. Guin, R. Frohlich and A. Studer, Angew. Chem., Int. Ed., 2008, 47, 779 CrossRef CAS PubMed;
(f) X.-Y. Duan, N.-N. Zhou, R. Fang, X.-L. Yang, W. Yu and B. Han, Angew. Chem., Int. Ed., 2014, 53, 3158 CrossRef CAS PubMed;
(g) X.-Y. Duan, X.-L. Yang, R. Fang, X.-X. Peng, W. Yu and B. Han, J. Org. Chem., 2013, 78, 10692 CrossRef CAS PubMed.
- For selected examples, see:
(a) X.-H. Yang, X.-H. Ouyang, W.-T. Wei, R.-J. Song and J.-H. Li, Adv. Synth. Catal., 2015, 357, 1161 CrossRef CAS;
(b) X.-H. Ouyang, R.-J. Song, Y. Li, B. Liu and J.-H. Li, J. Org. Chem., 2014, 79, 4582 CrossRef CAS PubMed;
(c) H.-L. Hua, Y.-T. He, Y.-F. Qiu, Y.-X. Li, B. Song, P. Gao, X.-R. Song, D.-H. Guo, X.-Y. Liu and Y.-M. Liang, Chem. – Eur. J., 2015, 21, 1468 CrossRef CAS PubMed;
(d) L.-J. Wang, A.-Q. Wang, Y. Xia, X.-X. Wu, X.-Y. Liu and Y.-M. Liang, Chem. Commun., 2014, 50, 13998 RSC;
(e) W.-T. Wei, R.-J. Song, X.-H. Ouyang, Y. Li, H.-B. Li and J.-H. Li, Org. Chem. Front., 2014, 1, 484 RSC.
Footnote |
† Electronic supplementary information (ESI) available. CCDC 1047910 (3ba) and 1047913 (7a). For ESI and crystallographic data in CIF or other electronic format see DOI: 10.1039/c5qo00352k |
|
This journal is © the Partner Organisations 2016 |